Fig. 23.1
(a–c): Anatomy of the aortic valve and root (a) Aortic valve in cross section. Each cusp is marked with (L), (R), and (N) representing the left, right, and noncoronary cusps, respectively. The left and right coronary arteries can be observed arising from their respective cusp. Note also how each cusp forms a pocket, termed the sinus of Valsalva. (b) Aortic valve viewed with the left cusp transected longitudinally. Open arrows note the level of the sinotubular junction, distal to which the ascending aorta (ASC AO) begins. Solid black arrows demonstrate the ostia of the right and left coronary arteries. (R), (L), and (N) mark the respective coronary cusps. The triangular space between each cusp is identified as the interleaflet triangle or trigone. The dotted line represents the area of fibrous continuity between the aortic and mitral valves. (c) Long-axis view of the left ventricular outflow tract, the aortic root, and the ascending aorta. The aortic root includes all structures between the annulus and the sinotubular junctions (the valve leaflets, their attachments, and the interleaflet triangles). The annulus of the aortic valve, the level of the sinuses of Valsalva, and the sinotubular junction are marked by dotted lines (Ho 2009)
Pathophysiology
Despite the immense variability in the anatomy and presentation of valvular AS, the pathology has a common element: increased left ventricular afterload that ultimately results in an imbalance between myocardial oxygen demand and coronary perfusion. In utero, the increased afterload can result in different phenotypes depending on the severity of the stenosis and the developmental stage at which it occurs (Marantz and Grinenco 2015). Those with lesser degrees of obstruction that develop late in gestation often maintain an adequate left ventricular size and function to accommodate a cardiac output in postnatal life (Allen 2013). Such individuals may present with failure to thrive or tachypnea as infants or may remain asymptomatic throughout childhood. Significant stenosis early in gestation, on the other hand, results in varying cardiac morphologies ranging from HLHS to severe left ventricular dilation (Affolter and Ghanayem 2014; Marantz and Grinenco 2015). The consequence of these more severe manifestations is a left ventricle that cannot manage the systemic circulation. Survival in postnatal life is then dependent on an atrial level shunt and a patent ductus arteriosus (“ductal-dependent” for systemic blood flow). The right ventricle in these cases accepts the bulk or all of the cardiac output and ejects not only to the pulmonary arteries but also systemically through the ductus arteriosus. Ductal closure invariably results in cardiovascular collapse and death.
In the child or adolescent with aortic stenosis, the pathophysiology is comparable to that observed in adults in that myocardial hypertrophy develops in response to the increased afterload (Opie 2004). By Laplace’s law (wall stress = pressure × radius/2 × wall thickness), hypertrophy acts to reduce wall stress while maintaining stroke volume and cardiac output, but at the expense of an increased left ventricular end-diastolic pressure (LVEDP) and increased myocardial oxygen demand (Donner et al. and 1983). Coronary blood flow can thus be compromised as the gradient between aortic end-diastolic pressure and LVEDP diminishes. Conditions that decrease diastolic blood pressure or increase myocardial oxygen demand can thus predispose toward ischemia, myocardial dysfunction, and arrhythmias. At greatest risk is the subendocardium due to the high compressive forces at this location and distance from the epicardial coronary arteries (Allen 2013). In utero, marked elevations in intracavitary pressures and increased myocardial oxygen demand can result in endomyocardial fibroelastosis (EFE), which can further contribute to postnatal ventricular dysfunction (Alsoufi et al. and 2007). If untreated, aortic stenosis ultimately overwhelms the left ventricle’s compensatory mechanisms and congestive heart failure ensues.
Critical Aortic Stenosis of the Neonate
Nearly 10 % of patients with congenital valvular AS present within the first year of life (Brown et al. 2003; Hastreiter et al. and 1963; McCrindle et al. 2001). Those who develop signs of failure as the ductus arteriosus closes are deemed to have critical aortic stenosis. Such neonates may present in cardiogenic shock as both systemic and coronary blood flow are compromised due to ductal closure. End-organ injury, myocardial ischemia, acidosis, and ultimately death result if untreated. Resuscitation entails the initiation of prostaglandin E1 to reopen the ductus and the use of inotropic and ventilatory support as needed to maximize oxygen delivery.
Definitive management is guided by the central decision of whether to employ a strategy of single-ventricle palliation versus biventricular repair (Alsoufi et al. 2007). Guiding this decision are factors such as the degree of left-sided hypoplasia observed and the presence of associated lesions like ventricular septal defects, arch hypoplasia, or coarctation of the aorta (Fig. 23.2). Those with severe hypoplasia of the left ventricle and mitral valve can proceed down the pathway of the Norwood procedure, the hybrid procedure, or heart transplantation depending on institutional preference and organ availability. Alternatively, if the neonate were to have only isolated critical AS with otherwise adequately sized structures, a strategy conducive to biventricular repair would be chosen. The “gray area” between these two extremes, however, presents a dilemma. Several studies have attempted to identify predictive factors to assist in the process of assessing the adequacy of the left ventricle to accommodate the systemic circulation (Hammon et al. 1988; Lofland et al. 2001; Rhodes et al. and 1991; Schwartz et al. 2001). Commonly referenced is the regression equation developed by the Congenital Heart Surgeons’ Society that is used to predict 5-year survival probability with Norwood-type palliation versus a biventricular approach (Lofland et al. 2001). Parameters assessed included patient age, grade of endomyocardial fibroelastosis, z-score of the aortic valve and left ventricular length, ascending aorta diameter, and the presence of significant tricuspid regurgitation. Underscoring the significance of appropriate initial therapy is data suggesting invariably worse outcomes when “crossover” between strategies is required (Rhodes et al. 1991).


Fig. 23.2
Management options in neonates with critical left ventricular outflow tract obstruction (Alsoufi et al. 2007)
For those neonates with isolated critical AS and adequate left heart structures, initial therapy entails either balloon or surgical valvuloplasty. No prospective randomized trials have compared outcomes between these techniques, and retrospective studies have yielded mixed data (McCrindle et al. 2001; Rehnstrom et al. 2007; Siddiqui et al. 2013). Confounding comparisons has been the evolution of both catheter-based and surgical techniques. Whereas surgical repair was once limited to rigid aortic valve dilation or blade commissurotomy, current techniques are more refined and include resection of nodular dysplasia and reconstruction of the aortic valve leaflets (Fig. 23.3) (Siddiqui et al. 2013). Such repairs, however, require cardiopulmonary bypass. Similar advances have been made in catheter technology, with improvements in the balloons and wires used in valvular dilation (Stapleton 2014).
Technical considerations of note in patients undergoing balloon valvuloplasty include the site of vascular access and potential use of rapid right ventricular pacing (Stapleton 2014). Either the femoral vessels or the right common carotid is commonly accessed. If the femoral vein is used, the aortic valve is approached antegrade; this route, however, is challenging as it requires passage across the atrial septum and through a potentially small left ventricle. The arrhythmias induced by catheter manipulation may exacerbate hemodynamic instability in an already tenuous patient. If the femoral artery is used, the aortic valve is approached retrograde. The most direct and expedient course to the aortic valve is achieved through the right common carotid. Long-term patency of this vessel does not appear to be affected (Borghi et al. 2001) if cannulated. If this approach is chosen, monitoring of cerebral oxygenation with cutaneous near-infrared spectroscopy probes may be useful to detect early signs of cerebral ischemia. Given the proximity of the catheters to the airway in this scenario, airway access may also be compromised. Vigilance is thus necessary to ensure that the endotracheal tube, if used, is not displaced or kinked. Rapid ventricular pacing is applied at the discretion of the interventional cardiologist at the time of balloon dilation. This serves to decrease left ventricular stroke volume, thereby minimizing the potential of balloon displacement during inflation with the associated risk of trauma to the aortic valve leaflets (Stapleton 2014).
While some degree of aortic insufficiency is expected following balloon dilation, the introduction of severe aortic insufficiency can be catastrophic. A recent series reported a 2 % incidence of severe insufficiency immediately post-ballooning (Brown et al. 2010; Petit et al. 2012). The additional volume load imposed on the left ventricle by the regurgitant valve increases left ventricular end-diastolic pressure and, in concert with lower aortic end-diastolic pressure, may produce marked myocardial ischemia. Emergent valve repair or replacement is necessary in this circumstance to prevent myocardial infarction. Even in those who have only minimal insufficiency following initial ballooning, progression may occur over time such that one-third of patients have moderate-to-severe regurgitation at 10 years post-procedure (Stapleton 2014). As balloon valvuloplasty is a palliative therapy whose goal is not complete elimination but improvement of the stenosis, the need for reintervention is high. Over two-thirds of neonates undergoing balloon valvuloplasty require either repeat ballooning or surgical intervention at 10 years following the procedure (Brown et al. 2010; McElhinney et al. and 2005).
Rarely, aortic valve replacement is necessary in the neonate or infant with critical AS (Alsoufi et al. 2007). Indications include the presence of complex multilevel left ventricular obstruction, significant aortic regurgitation that develops during balloon valvuloplasty, or stenosis refractory to balloon valvuloplasty or surgical valvotomy. Options include the use of a mechanical valve, bioprosthetic valve, or the patient’s own pulmonary valve. All such valves have drawbacks unique to the young and growing patient. Limitations common to bioprosthetic and mechanical valves include their lack of growth potential and subsequent need for frequent reoperation. Bioprosthetic valves moreover undergo accelerated degeneration as early as 2 years after valve replacement (Gallo et al. 1988; Clarke et al. 1993; Mavroudis and Backer 2013). Mechanical valves, in contrast, are more durable but suffer from thromboembolic complications that require long-term warfarin anticoagulation (Allen 2013; Mazzitelli et al. 1998).
The Ross procedure may be offered as an alternative when the patient’s pulmonary valve is of an adequate size and without stenosis or regurgitation (Al-Halees et al. 2002; Bansal et al. 2015; Kadner et al. 2008; Nelson et al. 2015; Ohye et al. 2001; Williams et al. 2005). This operation entails removing the stenotic aortic valve and replacing it with the patient’s own pulmonary valve. A homograft is then used to reconstruct the right ventricular outflow tract. The advantage of this procedure is that the pulmonary valve maintains its potential for growth in the aortic position, is durable, and does not require anticoagulation. Concerns following the Ross procedure include (i) the need for interval replacement of the homograft used to reconstruct the right ventricular outflow tract, (ii) dilation of the neo-aortic root, and (iii) progressive neo-aortic regurgitation and stenosis (Nelson et al. 2015; Stulak et al. 2010). Due to the critically ill state in which neonates and infants who are in need of valve replacement present, mortality following this operation is high, ranging from 12 to 75 % (Brancaccio et al. 2014; Kadner et al. 2008; Kirkpatrick et al. 2008; Tan Tanny et al. 2013). The use of postoperative extracorporeal membrane oxygenation (ECMO) is occasionally needed (Mascio et al. 2014; Shinkawa et al. 2010).
Irrespective of the initial therapy chosen, principles of anesthetic management focus on supporting myocardial function, balancing systemic and pulmonary blood flow, and optimizing oxygen delivery. In the neonate presenting in cardiogenic shock, therapy consists of continuing or initiating prostaglandin E1, inotropic and/or vasopressor support, and mechanical ventilation (Affolter and Ghanayem 2014). Both epinephrine and dopamine appear to be effective inotropes in neonates (Barrington et al. 1995; Valverde et al. 2006). Vasopressor support, while useful in maintaining diastolic pressure, may also compromise splanchnic perfusion and worsen left ventricular function by increasing afterload. The benefits of such agents must be weighed against the potential for these risks. When choosing ventilatory settings, care should be taken to avoid increasing pulmonary blood flow at the expense of systemic and coronary perfusion. Increases in inspired oxygen or hyperventilation may decrease pulmonary vascular resistance, resulting in increased left-to-right shunting through the ductus in diastole. Diastolic hypotension and reduced pulmonary compliance may ensue. In the subgroup of neonates with significant lung injury, however, increased inspired oxygen and aggressive ventilation may be necessary to achieve even marginal gas exchange. The choice of anesthetic often includes a combination of opioids and benzodiazepines; most drugs can be utilized safely assuming that doses are titrated to maintain hemodynamics while providing analgesia and minimizing oxygen consumption. Given the potential for blood loss or hemodilution with intervention, blood should also be readily available.
The Child or Adolescent with Valvular Aortic Stenosis
In contrast to the neonate or infant with critical AS, older children and adolescents with valvular obstruction are commonly asymptomatic (Allen 2013). This reflects the milder degree of stenosis initially present in these individuals (Mavroudis and Backer 2013) as well as the relatively slow rate of stenotic progression (Allen 2013). On average, the transvalvular gradient increases by 1 mmHg per year (Davis et al. 2008); the exception occurs in children whose somatic growth greatly exceeds that of their stenotic aortic valve. When symptoms do occur, easy fatigability is most often reported (Ellison et al. 1976). Dyspnea on exertion, angina, and syncope can also occur as in adults, but are relatively uncommon even in those with severe stenosis (Wagner et al. 1977).
Clinical severity is assigned based on catheter-derived or Doppler-derived gradients (Table 23.1, (Maron and Zipes 2005)). Peak-to-peak pressure gradients are catheter-derived and reflect the difference between peak left ventricular and peak aortic pressures. Peak instantaneous gradients, on the other hand, are Doppler-derived and reflect the point in time in which the velocity of blood across the aortic valve is greatest. These pressure gradients thus provide different data points and should not be used interchangeably (Beekman et al. 1992).
Catheter-derived peak-to-peak gradient | Mean Doppler gradient | Peak instantaneous Doppler gradient | |
---|---|---|---|
Mild | <30 mmHg | <25 mmHg | <40 mmHg |
Moderate | 30–50 mmHg | 25–40 mmHg | 40–70 mmHg |
Severe | >50 mmHg | >40 mmHg | >70 mmHg |
Factors influencing the gradient beyond the extent of valvular stenosis include the patient’s ventricular function and hemodynamic state at the time of measurement. Depressed ventricular function serves to underestimate one’s gradient, whereas increased contractility and tachycardia may significantly increase the gradient recorded. Likewise, gradients obtained under deep sedation or anesthesia can underestimate the extent of obstruction present during normal activity. Such variables require consideration when determining the severity of valvular stenosis.
Timing of intervention is guided by an assessment of the measured gradient, ventricular function, symptoms, and the patient’s level of activity (Allen 2013; Feltes et al. 2011). Those with peak-to-peak gradients >50 mmHg have an absolute indication for intervention even if asymptomatic given the increased and progressive risk of myocardial injury and sudden cardiac death. Individuals who wish to be active, have symptoms of angina or syncope, or have ischemic changes on exercise stress testing are directed toward intervention even with peak-to-peak gradients between 40 and 50 mmHg. Intervention is not recommended in the asymptomatic patient with a peak-to-peak gradient <40 mmHg unless ventricular function is also depressed.
As with neonates and infants, management options in children and adolescents include balloon valvuloplasty and surgical valve repair or replacement. Both balloon valvuloplasty and surgical valve repair are palliative therapies whose goals include reducing ventricular afterload and delaying the need for valve replacement. When valve replacement is indicated due to either progressive regurgitation or recurrent stenosis refractory to ballooning, options include the use of a mechanical valve, bioprosthetic valve, or pulmonary valve autograft. None are ideal. Both mechanical and bioprosthetic valves commit the child to future valve replacement due to their inability to grow. Mechanical valves, moreover, require anticoagulation. The pulmonary autograft or Ross procedure, while providing a durable and functional neo-aortic valve, also requires subsequent reoperation. Compared to neonates and infants, reoperation on the neo-aortic valve is more common in children and adolescents undergoing the Ross procedure; reintervention on the right ventricular outflow tract, however, is less common (Nelson et al. 2015).
Anesthetic goals entail maintaining preload, afterload, and contractility while targeting a normal to low-normal heart rate. Extended periods without fluid intake or the use of agents that significantly diminish preload or afterload can place the patient at risk for hypotension with the induction of anesthesia. Minimizing the fasting period or placing a preoperative intravenous catheter to give fluids can help decrease this risk. In the individual with significant aortic stenosis, low diastolic pressures can quickly produce a sequence of myocardial ischemia, ventricular dysfunction, bradycardia, and cardiac arrest. Alternatively, significantly increased contractility and tachycardia due to an inadequate level of anesthesia can also cause subendocardial ischemia. Careful titration of drugs while monitoring hemodynamics is thus essential to balance myocardial oxygen demand and supply.
Case Scenario #3
A 13-month-old infant with Williams syndrome presents for repair of (i) supravalvular aortic stenosis (SVAS), (ii) supravalvular pulmonary stenosis, and (iii) bilateral branch pulmonary stenosis. Preoperative evaluation with transthoracic echocardiography demonstrates:
Severe “hourglass” narrowing of the supravalvular aortic area with a peak velocity of 4.6 m/s
Mild narrowing at the level of the transverse aortic arch and isthmus
Moderate supravalvular pulmonary stenosis
Moderate-to-severe diffuse hypoplasia of the branch pulmonary arteries
Normal biventricular systolic function with moderate biventricular hypertrophy
A cardiac computed tomography angiogram (CTA) is also obtained and reveals the additional findings of mild hooding of the coronary arteries and discrete stenosis just distal to the left coronary ostium. No evidence of renal artery stenosis was seen and no other end-organ anomalies were identified. The patient is asymptomatic. On airway evaluation, mild mandibular hypoplasia is observed.
On the evening prior to surgery, the patient is admitted so that intravenous fluids can be administered during the fasting period. Anesthetic consent, including a discussion of the high risk of cardiac arrest during the pre-bypass period, is obtained. The family asks if there were any contingency plans if such an arrest were to occur, and the possibilities of extracorporeal membrane oxygenation (ECMO) or emergent initiation of cardiopulmonary bypass are presented.
The next morning, the availability of additional anesthesia staff, the surgeon, and the perfusionist is confirmed prior to entering the operating room. Blood has been delivered and checked, and the perfusionist is in the process of priming the bypass circuit. Having ensured the presence of the necessary personnel and equipment, intravenous premedication with midazolam is given, and the patient is brought to the operating room.
Following the placement of monitors, the patient is induced with fentanyl and 2 % sevoflurane. The airway is secured after the onset of muscle relaxant. Shortly thereafter, marked ST depressions are noted on the EKG with sinus tachycardia at a rate of 160. The noninvasive blood pressure cuff measures 70/30. While confirming the presence of end-tidal CO2 and bilateral breath sounds, boluses of phenylephrine and volume are given until the ST segments normalize. An infusion of vasopressin is initiated, and as help arrives, invasive arterial and central venous lines are obtained. The nursing team preps and drapes the patient, and the surgeon prepares for incision. Within 30 min, cardiopulmonary bypass is commenced.
The operation entails a (i) Doty pericardial patch repair of the supravalvular aortic and pulmonary stenoses and (ii) homograft enlargement of the branch pulmonary arteries. On direct inspection, neither coronary hooding nor left proximal coronary stenosis is observed.
Separation from bypass occurs uneventfully on milrinone and esmolol infusions. Postoperative echocardiography reveals no residual supravalvular or branch pulmonary obstruction and hyperdynamic biventricular function. Extubation is achieved on postoperative day 1, and the patient is discharged home on postoperative day 5.
Supravalvular Aortic Stenosis
While rare in the general population, supravalvular aortic stenosis (SVAS) occurs in nearly 45–75 % of those with Williams-Beuren (Williams) syndrome (Collins 2013; Eronen et al. 2002). Other familial or sporadic forms account for the remainder of SVAS cases observed. Though the obstruction generally occurs at the level of the sinotubular junction, all areas of the aortic root can be affected, including the coronary arteries and aortic valve leaflets. Such multilevel obstruction substantially increases the risk of myocardial ischemia and cardiac arrest during anesthesia (Burch et al. 2008; Matisoff et al. 2015). Given the underlying defect in the elastin gene (Nickerson et al. 1995), other large vessels can also be involved, including the descending aorta and pulmonary, brachiocephalic, mesenteric, and renal arteries (Burch et al. 2008; Pober et al. 2008). This variability in the structures affected and the subsequent risk imparted illustrate the complexity of this disease and the challenge in managing it.
Anatomy and Pathophysiology
Supravalvular aortic stenosis is classified as either diffuse or localized (Mavoroudis and Backer 2013). The majority have stenosis localized to the sinotubular junction, resulting in the characteristic hourglass appearance of the ascending aorta. Approximately 25 % of patients, however, have diffuse stenosis that can extend to the aortic arch (Kim et al. 1999; Stamm et al. 1997). Thickening at the sinotubular junction produces a narrowed aortic outflow that results in an increased afterload on the left ventricle, hypertension in the aortic root, and limited excursion of the aortic valve leaflets in systole (Mavroudis and Backer 2013). The valve cusps, moreover, can become adherent to the enlarging ridge (Flaker et al. 1983; Peterson et al. 1965). When this occurs, blood flow into the sinuses of Valsalva, and thus coronary blood flow, can be restricted. Compounding the potential for diminished coronary blood flow are anomalies of the coronary arteries themselves. Due to the severe hypertension in the aortic root, coronary artery disease can develop, with the most profound changes occurring proximally (Kim et al. 1999; Stamm et al. 1997). Moreover, the same process that leads to thickening of the aorta can also extend to and narrow the coronary ostia, further limiting coronary flow (Meairs et al. 1984).
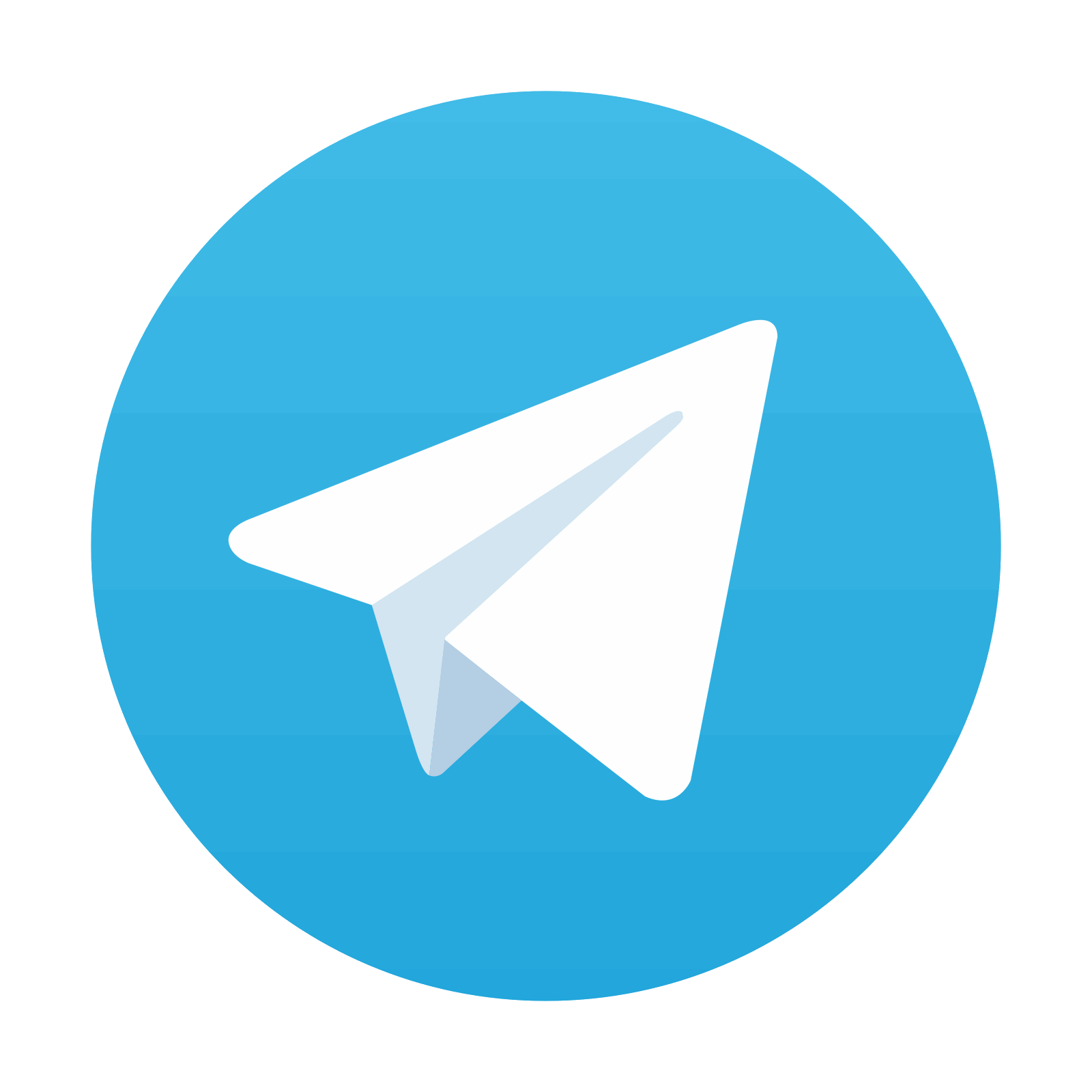
Stay updated, free articles. Join our Telegram channel

Full access? Get Clinical Tree
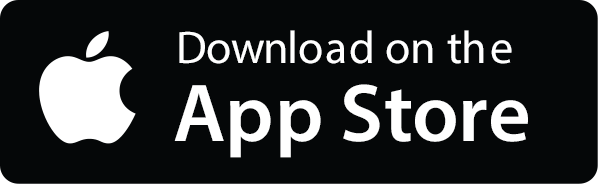
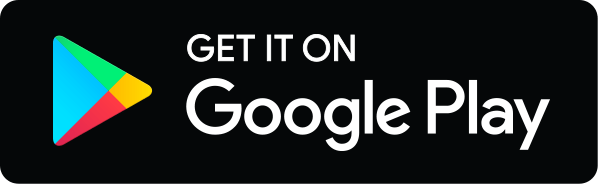