Interventions for Cardiac Arrhythmias
Haris M. Haqqani
Francis E. Marchlinski
INTRODUCTION
In the three decades since the first report of a catheter-based ablation procedure for medically refractory arrhythmias by Scheinman et al.,1 tremendous advances have been made in the field of interventional cardiac electrophysiology (EP). These advances have been predicated on a dramatic increase in our understanding of arrhythmia mechanisms, normal and abnormal cardiac anatomy, and ablation biophysics. Once these principles were established for any given arrhythmia, surgical procedures were developed to target and interrupt critical portions of the arrhythmia machinery. Following this, catheter-based procedures have been developed to recapitulate the success of the operative approach. This paradigm of progress in EP has occurred for both supraventricular and ventricular arrhythmias.
This chapter focuses on the current state of catheter-based interventions for cardiac arrhythmias, including present and evolving indications, periprocedural considerations, technical issues, outcomes, and complications.
CLASSIFICATION AND MECHANISMS OF ARRHYTHMIAS
All arrhythmias arise as a consequence of disordered electrical impulse formation or disordered impulse propagation. In terms of bradyarrhythmias, the commonest manifestation of the former mechanism is sinus node dysfunction, and of the latter, atrioventricular (AV) block. Permanent pacemaker (PPM) implantation is an adequate therapy for the majority of cases of bradycardia where intervention is required,2 and is not further addressed here. In terms of tachyarrhythmias, disorders of impulse formation result in focal tachycardias in which centripetal wavefronts emanate from a point-source. The mechanism of the disordered impulse formation may relate to either abnormal automaticity or to triggered activity. Tachyarrhythmias caused by abnormal impulse propagation result from reentry of wavefronts around anatomically and/or functionally determined circuits, and are much more common than focal tachycardias. Reentry requires the presence of longitudinal dissociation of a tissue by either functional or anatomic means such that there are two possible paths for a propagating wavefront to activate. It also requires the presence of unidirectional conduction block to allow for conduction to occur down the nonblocked path in isolation. And, crucially, it also requires the presence of significant slow conduction to allow for recovery of the blocked path by the time the wavefront returns to the original site of block.
Apart from mechanism, tachycardias are also classified into narrow- and broad-complex tachycardia based on the width of the surface 12-lead QRS complex. This in turn is determined by the presence or absence of ventricular activation exclusively over a normal conducting AV nodal His-Purkinje axis. Almost all regular narrow-complex tachycardias are due to supraventricular tachycardia (SVT) in which the tachycardia mechanism involves the atrial and/or subatrial junctional tissue, although it may involve the ventricles in addition. Also, atrial fibrillation (AF) and atrial flutter (AFL) usually present as narrow-complex tachycardia, although they are typically distinguished by marked cycle length irregularity in the former or by the presence of characteristic flutter waves in the latter. Broad-complex tachycardia can be due to ventricular tachycardia (VT), SVT with fixed or functional bundle branch block (also known as aberrant conduction), preexcited tachycardias (in which ventricular activation occurs partially or completely over a manifest bypass tract), paced rhythms, and artifacts. Invariably, the first aim of any procedural intervention for arrhythmias is to categorize and define the precise mechanism for the targeted arrhythmia.
GENERAL PRINCIPLES AND PERIPROCEDURAL CONSIDERATIONS
Most patients with clinical arrhythmias requiring procedural intervention now undergo diagnostic EP studies to define the precise arrhythmia mechanism and then subsequent catheter ablation at the same sitting. Consequently, after case selection, the most important periprocedural consideration is careful examination of all available clinical electrocardiograph (ECG) traces. In many cases, such as with atrial tachycardia, atrial flutter, Wolf-Parkinson-White (WPW) syndrome, or idiopathic VT, an intracardiac ablation target may be defined with great precision before commencing. In SVT and idiopathic VT cases, the ECG of the clinical tachycardia may help in procedural planning such as identifying the need for transseptal puncture, arterial access for left ventricular (LV) ablation, or pericardial access for epicardial ablation. Documentation and diagnosis of clinical arrhythmia events may be essential to accurately interpret the results seen at electrophysiologic testing. Detailed consideration of ECG interpretation is beyond the scope of this chapter but has been extensively reviewed elsewhere.3, 4, 5, 6
One of the reasons for the success of the surface 12-lead ECG in localization of arrhythmias is, in the absence of significant structural cardiac disease, the relatively constant anatomic relationship between the heart, various intracardiac structures, and the thoracic wall. Successful ablation of many arrhythmias requires a thorough spatial understanding of this three-dimensional (3D) geometry. In simple arrhythmias that can be dealt with by focal ablation, such as most forms of SVT, this geometry is generally predictable to the point that advanced individual preprocedural imaging is not required, although most patients undergo noninvasive transthoracic echocardiography to definitively exclude structural heart disease. In these cases, intraprocedural imaging with fluoroscopy alone is usually sufficient.
For more complex arrhythmias, a transthoracic echocardiogram is a mandatory minimum to confirm the status of valve structures and cardiac function. It will also exclude LV thrombus if catheters are to be placed in that chamber in patients with LV structural heart disease (Figure 39.1).
For patients with persistent AF a transesophageal echo is required to exclude left atrial appendage thrombus before cardioversion or AF ablation procedures. More complex arrhythmias, such as AF, atypical AFL, and scar-related VT, are often caused by various degrees of myocardial scarring, and usually require additional tools for successful ablation. Chief among these are 3D electroanatomic mapping (EAM) systems. Utilizing either magnetic or impedance-based catheter localization, these allow for the position and orientation of intracardiac mapping catheters to be registered in a virtual 3D geometry of the patient’s heart. The two most commonly used systems are CARTO XP/3 (Biosense Webster, Diamond Bar, CA) and NavX (St Jude Medical, Sylmar, CA). At each registered position of the catheter, the electrical mapping information it contains, for instance the bipolar voltage or local activation time, can be plotted on color-coded 3D maps to allow for rapid assessment of arrhythmia mechanisms, reentrant circuit locations, and substrate characteristics (Figure 39.2).
The systems allow for significantly lower ionizing radiation exposure for both the patient and the operator. For complex arrhythmias, preprocedural imaging with computed tomography (CT) or cardiac magnetic resonance (CMR) imaging has become widely used. The latter is particularly useful in view of the ability to directly visualize fibrosis by employing delayed gadolinium enhancement (DGE) techniques.7 The CT or CMR geometry may also be imported into the virtual geometry created by the EAM system, a process known as image integration. Also, real-time imaging during the procedure with intracardiac echocardiography (ICE) has become increasingly deployed. This assists with catheter manipulation, defining manipulation, obtaining transseptal or pericardial access when required, visualizing catheter contact, assessing lesion formation, and continuous online monitoring for complications such as cardiac perforation, pericardial tamponade, and pulmonary vein stenosis.8
Interventional EP procedures are generally performed via the femoral venous route, although sometimes jugular, subclavian, arterial, or percutaneous direct pericardial access may be required. The initial aims are to study the arrhythmia substrate and then to induce the clinical tachycardia so that its precise mechanism can be defined. This is accomplished by means of an EP study in which multipolar intracardiac recording catheters are placed in strategic locations such as in the coronary sinus (CS) and at the His bundle recording position.
The baseline properties of refractoriness and conduction in the atrium, ventricle, atrioventricular (AV) junction, and His-Purkinje system are then defined using a sequence of pacing maneuvers known as programmed stimulation. The clinical tachycardia is often induced during process, but sometimes beta adrenergic stimulation using isoproterenol is required before induction attempts are repeated. After arrhythmia induction, the patient is examined for evidence of hemodynamic tolerance before proceeding further. The tachycardia is first studied in its unperturbed state to attempt to reach a diagnosis. For this, the mode of induction, the surface ECG morphology and characteristics, the cycle length and any irregularity, zones of transition, the AV relationship, the atrial and ventricular activation sequence, and the mode of termination are all carefully examined. Thereafter, the response of the tachycardia to well-established pacing maneuvers is studied and a diagnosis established.
The baseline properties of refractoriness and conduction in the atrium, ventricle, atrioventricular (AV) junction, and His-Purkinje system are then defined using a sequence of pacing maneuvers known as programmed stimulation. The clinical tachycardia is often induced during process, but sometimes beta adrenergic stimulation using isoproterenol is required before induction attempts are repeated. After arrhythmia induction, the patient is examined for evidence of hemodynamic tolerance before proceeding further. The tachycardia is first studied in its unperturbed state to attempt to reach a diagnosis. For this, the mode of induction, the surface ECG morphology and characteristics, the cycle length and any irregularity, zones of transition, the AV relationship, the atrial and ventricular activation sequence, and the mode of termination are all carefully examined. Thereafter, the response of the tachycardia to well-established pacing maneuvers is studied and a diagnosis established.
Clinical management of patients during these procedures is often complex. The demands of patient tolerance and catheter stability have to be balanced with the detrimental effects of sedation and anesthesia on tachycardia inducibility and hemodynamic stability. The latter is of particular concern during scar-related VT ablation in patients with dilated ventricles and poor systolic function. Outside of the pediatric setting, the majority of adult EP procedures are carried out under conscious sedation, with one noticeable exception being AF ablation where a randomized comparison has shown better outcomes with general anaesthesia.9 While hemodynamic tolerance is not an issue for most SVT procedures, either inotropic or mechanical circulatory support is often required for scar-related VT ablation, both to allow for catheter mapping during VT or to improve the patient’s general circulatory status during what are often long and complicated procedures. This may be in the form of an intraaortic balloon pump (IABP) or a percutaneous ventricular assist device (VAD).10
While right-sided arrhythmia mechanisms are readily accessible to transvenously placed catheters, mapping and ablating arrhythmias in the left atrium or left ventricle require either a retrograde transaortic approach (usually via the common femoral artery) or a transseptal puncture. Retrograde approaches are technically more demanding for the ablation of most left-sided SVTs and hence electrophysiologists have become very comfortable with obtaining transseptal access with the assistance of one or more of (i) a His-bundle recording catheter to mark the position of the noncoronary aortic sinus of Valsalva; (ii) radiocontrast administration to stain the interatrial septum and to ensure safe advancement of the needle/sheath assembly; (iii) ICE or transesophageal (TEE) online imaging guidance; and (iv) needle tip manometry. Further technical descriptions of transseptal techniques are found in Chapter 6.
Some complex EP procedures require access to the pericardial space to enable epicardial mapping and ablation. The percutaneous approach to pericardial access was pioneered by Sosa et al.11 in the context of Chagas disease (and its associated epicardial VTs) and is now performed routinely by electrophysiologists. Under fluoroscopic guidance and after complete reversal of any anticoagulation, a 17G Tuohy needle (with an atraumatic curved tip) is advanced via the subxiphoid space into the triangle of Larry until a characteristic pop is felt as the fibrous pericardium is breached. A small volume of radiocontrast is then injected to confirm the characteristic pericardial layering of contrast and then a super-stiff, exchange-length 0.035 inch guidewire is advanced. Crucially, as during routine pericardiocentesis, guidewire placement is then confirmed in the left anterior oblique projection to ensure that it is contained within the outline of the pericardium and that it has crossed the boundaries between all four cardiac chambers, hence excluding the possibility of it being intracardiac (Figure 39.3). A sheath is then advanced and its side arm aspirated to exclude significant bleeding. If inadvertent right ventricular (RV) puncture occurs, the needle is simply withdrawn slightly and the pericardial space rewired. Apart from RV laceration, additional risks of pericardial access and epicardial ablation include intraabdominal bleeding, coronary artery injury and occlusion, phrenic nerve injury and failure of access due to prior surgery, radiation, or pericarditis. However, the risk of these complications is
low12 and the addition of this extra dimension to the interventional electrophysiologist’s toolkit has increased the number of complex arrhythmias that can be successfully ablated especially as it relates to VT.
low12 and the addition of this extra dimension to the interventional electrophysiologist’s toolkit has increased the number of complex arrhythmias that can be successfully ablated especially as it relates to VT.
The most commonly used energy source for catheter ablation is radiofrequency (RF) current.13 Radiofrequency energy delivery has the advantage of being readily generated and easily titrated to effect and its introduction to clinical practice was a huge advance over the relatively uncontrolled shocks of DC ablation. In addition, RF biophysics is relatively well understood14 and in most situations lesion size can be predicted and controlled. For most simple arrhythmias, focal lesions can be created with a 4 mm 7F solid-tip catheter using 30 to 50 W with a 50°C to 60°C catheter tip temperature cutoff. The use of large-tip and irrigated catheters has significantly increased the depth of lesions that can be delivered and this has been vital in improving the success of ablation procedures for complex scar-related arrhythmias. The basic principle behind irrigated ablation relates to the ability of surface cooling to allow for greater power delivery to deeper tissues. Not only does this increase lesion depth but it also reduces the incidence of endothelial thrombus and char formation, particularly with open irrigation.15
Apart from RF current, other ablation energy sources that have been investigated include cryoenergy, laser, microwave, and high-frequency ultrasound. Of these, cryoablation has established the clearest role in view of its increased safety and near-comparable efficacy for many ablation procedures, particularly those for SVT.16 This is an important additional option when ablation targets are located close to sensitive structures such as the AV node or the coronary arteries. Cryoballoon technology for paroxysmal AF has recently been approved in the United States to isolate pulmonary veins. Appropriate balloon sizing, placement, and pacing are required to avoid right phrenic nerve injury associated with right superior pulmonary vein isolation.17 Although not an energy source per se, there is a niche role for intracoronary ethanol in the treatment of select intramural VT substrates not accessible by either endocardial or epicardial ablation.18
SUPRAVENTRICULAR TACHYCARDIA
In adults, three main tachycardia mechanisms comprise the vast majority of SVT cases: atrioventricular nodal reentrant tachycardia (AVNRT), atrioventricular reentrant tachycardia (AVRT), and atrial tachycardia (AT). Junctional ectopic tachycardia is a rare diagnosis in adults and is seen much more frequently in the pediatric context. Recent data from a large center showed that AVNRT accounted for 56% of SVT, with AVRT seen in 27%, and AT in 17% overall, although there was a significant age and gender influence observed.19
Most patients with palpitations and SVT have a benign prognosis, particularly if there has been no history of syncope or hemodynamic compromise. This also applies to patients with WPW syndrome. These patients are given a choice of management strategies including (i) ongoing observation only; (ii) regular medication to suppress SVT such as verapamil, metoprolol, or flecainide; (iii) intermittent medication to be taken at the time of an episode (the so-called pill-in-the-pocket approach); or (iv) electrophysiologic evaluation with a view to catheter ablation if the target can be appropriately identified. Most patients who come to medical attention are symptomatic enough that they would like treatment. The very high success rate of catheter ablation in treating most underlying mechanisms of SVT and WPW20 and the low incidence of complications21 mean that the vast majority of patients prefer this approach to lifelong medication. The joint American College of Cardiology (ACC) and American Heart Association (AHA) Task Force guidelines give catheter ablation a class I indication for symptomatic SVT or WPW that is “drug-resistant or the patient is drug intolerant or does not desire long-term drug therapy.”22
Atrioventricular Nodal Reentrant Tachycardia
The exact mechanism of AVNRT remains unclear despite many decades of detailed research, and despite the existence of a routine ablation procedure that has a 98.5% long-term cure rate.23 While it is generally agreed that the AV node has functional longitudinal dissociation in patients with AVRNT, the degree of involvement of subnodal atrial tissue in the reentrant circuit is still debated. The footprint of this longitudinal dissociation during EP testing is the presence of AV
node duality in which at least one discontinuity is seen in the curve representing the output of the AV node to increasingly premature atrial extrastimulus testing. This is represented schematically as a so-called fast pathway that displays rapid conduction velocity but relatively longer refractoriness, and a slow pathway that has slower conduction but shorter refractoriness. In the basal state, conduction proceeds down both pathways but only fast pathway activation is manifest on the surface ECG. Classically, at a critical prematurity of atrial extrastimulus, unidirectional conduction block occurs in the fast pathway due to its longer refractory period and activation proceeds only down the slow pathway resulting in a long PR (or AH) interval. If the fast pathway has recovered excitability by this time, retrograde conduction to the atrium can occur over it, resulting in an “echo beat” with a very short RP interval, and sustained AVNRT if the cycle repeats itself. The very short RP interval means that atrial and ventricular activation is simultaneous and the narrow retrograde septal P wave is either difficult to discern on the surface ECG, or is seen as a small terminal inscription on the QRS complex (pseudo S-wave in lead II and R’ in V1).
node duality in which at least one discontinuity is seen in the curve representing the output of the AV node to increasingly premature atrial extrastimulus testing. This is represented schematically as a so-called fast pathway that displays rapid conduction velocity but relatively longer refractoriness, and a slow pathway that has slower conduction but shorter refractoriness. In the basal state, conduction proceeds down both pathways but only fast pathway activation is manifest on the surface ECG. Classically, at a critical prematurity of atrial extrastimulus, unidirectional conduction block occurs in the fast pathway due to its longer refractory period and activation proceeds only down the slow pathway resulting in a long PR (or AH) interval. If the fast pathway has recovered excitability by this time, retrograde conduction to the atrium can occur over it, resulting in an “echo beat” with a very short RP interval, and sustained AVNRT if the cycle repeats itself. The very short RP interval means that atrial and ventricular activation is simultaneous and the narrow retrograde septal P wave is either difficult to discern on the surface ECG, or is seen as a small terminal inscription on the QRS complex (pseudo S-wave in lead II and R’ in V1).
Catheter-based interventions for selective ablation of the AVNRT circuit without producing AV nodal block have been sought from the time Ross et al. pioneered the surgical technique of dissection in the triangle of Koch (which is formed by the CS os, the tricuspid annulus, and the tendon of Todaro) at the site of earliest atrial activation during tachycardia.24 Initial attempts targeted the fast pathway located in the anterior portion of the triangle. This was effective but resulted in an unacceptable incidence of AV block. The standard technique in use today is selective ablation or modification of the slow pathway with a procedural endpoint of tachycardia noninducibility. This was first described in 1992 by Jackman et al.25 They mapped and targeted high-frequency potentials located in the posterior region of the triangle of Koch (between the CS os and the tricuspid annuls) during sinus rhythm. Although these and other investigators found evidence linking these abnormal potentials to the tachycardia circuit, subsequent high-density array mapping of the triangle of Koch during surgical slow pathway ablation by McGuire et al. proved these potentials to be related to asynchronous muscle bundle activation around the CS os and not pathogenic for tachycardia.26,27 Currently, the most widely used method of slow pathway mapping is largely anatomic with the ablation catheter positioned just outside the CS against the septal tricuspid annulus in a position where a sharp but low-amplitude atrial electrogram is located along with a large ventricular signal. If RF applications (40 to 50 W power) here are not successful, the catheter is moved slightly superiorly in the triangle and energy delivery is repeated. At successful sites, longer and slightly faster runs of junctional rhythm are usually obtained compared to unsuccessful sites where only isolated junctional beats or slow junctional rhythm is seen.28 Very fast junctional rhythm during ablation may be a risk for impending AV block however, and applications are ceased immediately if this is seen. Ablation is also immediately discontinued if VA block is seen during junctional rhythm as this is a marker of impending antegrade heart block.28 After successful RF applications have been delivered, EP testing is repeated to assess for residual slow pathway function on and off isoproterenol. Multiple series have shown that AVNRT recurrence after slow pathway ablation (which is less than 2%) is not predicted by the presence of either residual slow pathway conduction or single echo beats.23,25 Consequently, AVNRT noninducibility remains the most important procedural endpoint. The success and safety of slow pathway RF ablation is not less favorable in the elderly either and is a first-line option across all age groups.29
Aside from more minor complications such as venous access site bleeding, the main material risk to patients undergoing slow pathway ablation for AVNRT is inadvertent AV block. Various studies initially suggested this to be less than 1% but more recent, large single-center series have shown an even lower rate of persistent AV block requiring permanent pacing of 0.07%.23 Strategies to minimize the risk of AV block with RF ablation include the use of long preformed sheaths to increase catheter stability and, in difficult cases, the use of general anesthesia to allow for reduced cardiac motion with respiratory excursion. Alternatively, and particularly in the pediatric context, cryoablation may be considered a safer, although less effective option for slow pathway ablation.30
Atrioventricular Reentrant Tachycardia and Wolff-Parkinson-White Syndrome
Tachycardias mediated by a bypass tract (BT) (also known as an accessory pathway) are grouped under the category of AVRT, and in contrast to the small circuit of AVNRT, these arrhythmias are due to large-loop reentry involving the atrium, the normal AV conduction system (AVCS), the ventricle, and the BT. When antegrade conduction is comprised of the AVCS and the BT serves as the retrograde limb, the tachycardia is called orthodromic reentrant tachycardia (ORT). This presents as a standard narrow-complex SVT but because the ventricle is part of the circuit of ORT and requires time to activate before the wavefront can return to the atrium, the RP interval on the ECG is longer with a clearly discernable P wave often present in the ST segment. In patients whose BT can only conduct in the retrograde direction from ventricle to atrium (called concealed BT), ORT is the only possible BT-mediated arrhythmia possible. If the BT can conduct in the antegrade direction from atrium to ventricle, it is known as a manifest BT as it will usually produce a short PR interval and delta wave (preexcitation) on the surface ECG since it bypasses the physiologic delay produced by decremental conduction in the AV node. If patients with such manifest BTs have symptoms of tachycardia, then WPW syndrome is present. The group of tachycardias in which antegrade conduction occurs over a manifest BT is known as preexcited tachycardias, and all
present as broad-complex tachycardias on ECGs since they do not activate the ventricle over the normal AVCS. One of these is a form of AVRT that, in contrast to ORT, displays antegrade activation down the manifest BT and through the ventricle before proceeding back to the atrium via retrograde conduction up the normal AVCS. This is known as antidromic tachycardia (ART) and presents clinically as a broad-complex tachycardia resembling some basal forms of VT. In a variant of ART, retrograde conduction may be up a second BT rather than the AVCS. Apart from ART, other forms of preexcited tachycardias involve bystander activation of the ventricle over the BT. These include preexcited AVNRT, preexcited AT, and preexcited AF. The latter rhythm in particular may be lethal if the antegrade conduction characteristics of the BT allow for extremely rapid ventricular activation that may degenerate to ventricular fibrillation. This probably accounts for the small incidence of sudden death seen with WPW, though this risk is not high enough to justify routine ablation of asymptomatic patients with preexcitation.31,32
present as broad-complex tachycardias on ECGs since they do not activate the ventricle over the normal AVCS. One of these is a form of AVRT that, in contrast to ORT, displays antegrade activation down the manifest BT and through the ventricle before proceeding back to the atrium via retrograde conduction up the normal AVCS. This is known as antidromic tachycardia (ART) and presents clinically as a broad-complex tachycardia resembling some basal forms of VT. In a variant of ART, retrograde conduction may be up a second BT rather than the AVCS. Apart from ART, other forms of preexcited tachycardias involve bystander activation of the ventricle over the BT. These include preexcited AVNRT, preexcited AT, and preexcited AF. The latter rhythm in particular may be lethal if the antegrade conduction characteristics of the BT allow for extremely rapid ventricular activation that may degenerate to ventricular fibrillation. This probably accounts for the small incidence of sudden death seen with WPW, though this risk is not high enough to justify routine ablation of asymptomatic patients with preexcitation.31,32
Adequate preprocedural planning, ECG interpretation, and anatomic understanding are important factors in catheter ablation of BTs. Due to reasons of embryogenesis, the AV valve annuli are the commonest locations of BTs, although less common sites such as the right and left atrial appendage, the aortic sinuses of Valsalva, and the coronary venous system can be involved. BTs are 5 to 10 mm in length and 0.1 to 7 mm in diameter, and generally traverse the epicardial AV groove subjacent to the overlying fat pad.33,34 The majority of BTs are related to the mitral annulus, although right-sided and septal BTs account for up to 46%.35 To eliminate a left-sided BT the operator must decide between transseptal and retrograde approaches, both of which have been shown to be equally effective.36 However, once at the mitral annulus, catheter stability is easily maintained and mapping and ablation are generally uncomplicated. In contrast, right free wall BTs, while easily accessed from the venous approach, are technically much more challenging due to the difficulties associated with stable catheter contact on the highly mobile tricuspid annulus. This is reflected in the lower acute success rate of ablation and higher recurrence risk compared to left-sided BT.37 Importantly, there is a well-described association between right-sided BTs (often multiple) and Ebstein anomaly, a situation of much greater anatomic and electrophysiologic complexity because of the shift in the anatomic location of the tricuspid valve and the ventricularization of atrial myocardium. A lower acute and chronic success rate for catheter ablation in this context is minimized by careful identification of the AV groove.38
Although surface ECG algorithms can regionalize the BT site well enough to guide and streamline the mapping process,5 precise localization requires intracardiac mapping with an EP catheter. The most commonly used technique for this relies on the determination of the site of the earliest atrial electrogram during retrograde BT conduction, or of the site of earliest ventricular activation pre-delta-wave during antegrade conduction over a manifest BT. At such sites, the relative timing of the local atrial and ventricular activation may be short or fused. A short AV interval is not used as a mapping criterion for precise localization because synchronous activation of late signals on either side of the annulus may also give rise to such short intervals away from the BT location. If mapping occurs during atrial or ventricular pacing, a degree of fusion may occur between wavefront conduction down the BT and the normal AVCS. This may not be a problem for BTs located far from the AVCS but can complicate mapping paraseptal BTs. If earliest electrograms are mapped during orthodromic tachycardia, fusion of wavefronts will not be a problem since retrograde activation occurs entirely over the BT. As sudden tachycardia termination with ablation may result in catheter movement from the site before fulguration is complete, ablation may be best performed during pacing and not SVT. The second technique for BT localization relies on recording a sharp bipolar potential between the atrial and ventricular electrograms that represents depolarization of the BT itself. Such potentials are usually obscured if the interval between atrial and ventricular signals is short. As the majority of BTs actually pursue an oblique course across the AV groove, changing the direction of pacing wavefronts can result in lengthening of the local VA or AV interval, unmasking the obscured BT potential.39
There are several special situations in BT ablation where particular difficulties and risks are encountered. Septal BTs are an obvious example where the risk of AV block can be significant. Due to their proximity to the compact AV node, this risk is probably greatest for midseptal BTs.35 Anteroseptal BTs are often located in very close proximity to the penetrating bundle of His, where they are sometimes called parahisian BTs. This is particularly the case if a His potential is seen on the ablation catheter at the successful ablation site. Although there are no conclusive data, the risk of AV block is probably less than it is with midseptal BTs as the fibrous tissue sheath in which the His bundle resides may exert a protective effect. Due to the contiguous proximity of the His bundle and the aortic root, anteroseptal BTs can be mapped and ablated from the noncoronary aortic sinus of Valsalva.40
Notwithstanding the risk of AV block with midseptal and anteroseptal BTs, it is widely considered that posteroseptal BTs are the most challenging to ablate. They account for around 25% of BTs in most series, and they cause difficulty due to the anatomic complexity of the 3D region in which they reside, the pyramidal space. This is located at the inferior crux of the heart and is a region where all four cardiac chambers (or five if the CS with its contractile muscular coat is also considered a chamber) abut each other. It contains autonomic ganglia, epicardial fat, the left and right posterior extensions of the AV node, and most critically, the branches of the distal right coronary artery including the AV nodal artery.41 Most posteroseptal BTs course obliquely through the pyramidal space from the right
atrium to the posterosuperior process of the left ventricle. Some posteroseptal BTs result from epicardial muscular connections between the coats of the coronary venous system (the CS and the middle or posterior cardiac veins) and the left ventricle.42 The Oklahoma group found that 21% of these epicardial sinoventricular connections were associated with a CS diverticulum.43 While some posteroseptal BTs may be readily ablated from the inferoseptal tricuspid annulus, below the CS os, many require extensive mapping from transseptal, retrograde, subclavian, CS, middle cardiac vein, or even percutaneous epicardial approaches. Some posteroseptal BTs have decremental conduction and may be responsible for a near incessant orthodromic tachycardia, more commonly in children, known as the permanent form of junctional reciprocating tachycardia (PJRT). This can cause a tachycardia-mediated cardiomyopathy and ablation has been well documented to reverse the depressed LV function.44
atrium to the posterosuperior process of the left ventricle. Some posteroseptal BTs result from epicardial muscular connections between the coats of the coronary venous system (the CS and the middle or posterior cardiac veins) and the left ventricle.42 The Oklahoma group found that 21% of these epicardial sinoventricular connections were associated with a CS diverticulum.43 While some posteroseptal BTs may be readily ablated from the inferoseptal tricuspid annulus, below the CS os, many require extensive mapping from transseptal, retrograde, subclavian, CS, middle cardiac vein, or even percutaneous epicardial approaches. Some posteroseptal BTs have decremental conduction and may be responsible for a near incessant orthodromic tachycardia, more commonly in children, known as the permanent form of junctional reciprocating tachycardia (PJRT). This can cause a tachycardia-mediated cardiomyopathy and ablation has been well documented to reverse the depressed LV function.44
Atriofascicular BTs account for no more than 2% to 3% of all BTs but are notable for their fascinating anatomy and physiology, best described as a duplicated accessory AV nodal conduction system located on the free wall of the tricuspid annulus.45 They are usually long fibers that extend from the posterolateral tricuspid annulus to their commonest insertion into the distal ramification of the right bundle branch. These BTs are known colloquially (and incorrectly) as Mahaim fibers. They are notable for having antegradeonly, decremental conduction, sometimes with marked longitudinal dissociation. Their very slow conduction generally means that sinus rhythm ECGs have minimal or absent signs of preexcitation as most of the ventricular mass has been depolarized by the wavefront proceeding down the normal AVCS before the atriofascicular wavefront can manifest. The absence of retrograde conduction means that orthodromic tachycardia is not possible, and the clinical arrhythmia they most commonly cause is an antidromic tachycardia in which antegrade activation is over the atriofascicular fiber and retrograde conduction is via the AVCS. This is manifest on the surface ECG as a late-transition left bundle branch block (LBBB) morphology broad-complex tachycardia. These atriofascicular pathways are mapped by searching for the large BT potential (analogous to a His potential) that they exhibit on the posterolateral tricuspid annulus, usually with the assistance of a multipolar catheter placed around the annulus.46 The main challenge in catheter ablation for atriofascicular BTs is, like all right free-wall BTs, catheter stability on the mobile tricuspid annulus that can be aided by using sheaths to support the floppier catheter tip. In addition, these BTs are superficial structures that are extremely vulnerable to catheter trauma, which can frustrate mapping attempts by causing them to transiently disappear during the procedure.
The overall success rate in catheter ablation of BTs approaches 93% to 98% long-term with a 2.2% redo rate for recurrence.20,37 However, success rates can be as low as 88% for posteroseptal BTs. The rates of major complication range from 0.6% to 1% in large, multicenter series with cardiac tamponade and heart block (parahisian BTs) being the commonest serious adverse events.
Focal Atrial Tachycardia
Focal AT accounts for 5% to 15% of SVTs in patients undergoing catheter ablation.47 The electrophysiologic mechanism can be a result of abnormal automaticity, triggered activity, or microreentry, and a variable response of the tachycardia focus to adenosine has been described as a result.48 Focal ATs do not arise randomly from all over the atria and are instead clustered around classic sites of anatomic heterogeneity. In the right atrium these include the crista terminalis,49 the tricuspid annulus,50 the CS os,51 the right atrial appendage,52 and the parahisian/perinodal regions. The latter can often be successfully and safely ablated from the noncoronary aortic sinus of Valsalva53 (Figure 39.4). In the left atrium, commonest sites of origin are the pulmonary veins,54 followed by the fossa ovalis and mitral annulus at the aortomitral continuity,55 the rest of the mitral annulus and CS, and the left atrial appendage.56
Some focal ATs can be notoriously difficult to induce, particularly those due to triggered activity and liberal doses of catecholamines may be required in addition to burst pacing or programmed stimulation. This is often the biggest challenge in successful ablation as mapping can be almost impossible with noninducible or nonsustained tachycardia, and the ablation endpoint is uncertain. On the other hand, some focal AT sites of origin, particularly the atrial appendages, are associated with incessant tachycardia and the risk of development of tachycardia-mediated cardiomyopathy.57
The most important step once focal AT has been proven by pacing maneuvers as the tachycardia mechanism is to analyze the P wave morphology. Transient AV block may need to be induced by rapid ventricular pacing if the P wave is obscured by the preceding T wave. In the absence of structural heart disease, the P wave morphology is a very reliable guide to the site of origin of focal AT.3 Also, the P wave onset must be defined relative to a fixed intracardiac fiducial reference signal (e.g., the CS os) as most successful ablation sites precede the P wave onset by 20 to 30 ms.58 Point by point activation mapping can then be targeted to the site of origin defined by the P wave, often assisted by 3D mapping systems. At successful sites the onset of RF energy delivery is often marked by an acceleration of the tachycardia, bipolar signal attenuation, and then abrupt termination. The published success rates of catheter ablation for focal AT are variable (69% to 100%) and largely reflect difficulties related to reliable induction of sustained tachycardia, catheter stability at some sites such as the tricuspid annulus or right atrial appendage, and proximity to sensitive structures such as the AV node.58
MACROREENTRANT ATRIAL TACHYCARDIAS
Nomenclature
A number of different names have been given to tachycardias whose basic mechanism consists of large loop reentry around the right or left atria, referred to here as macroreentrant atrial tachycardia (MRAT). They can be divided into two main groups, cavotricuspid isthmus (CTI)-dependent tachycardia and non-CTI-dependent. The former group accounts for the vast majority of MRAT, and consists of typical AFL, reverse typical AFL, and lower loop reentry. Broadly speaking, non-CTI-dependent MRAT is often called atypical flutter, and is due to large right or left atrial circuits related to the presence of atrial scarring. The use of the term “atrial flutter” in describing MRATs has come to describe a surface ECG atrial activation pattern of continuous undulation of the baseline.
Cavotricuspid Isthmus-Dependent Flutter
The reentrant circuit of typical AFL has been well characterized.59, 60, 61 It consists of a large loop rotating around the counterclockwise tricuspid annulus and confined entirely to the right atrium with left atrial activation being passive. The wavefront exits the narrow protected slow zone just posterior to the coronary sinus os, travels up the septum, down the free wall anterior to the crista terminalis, and then travels through the CTI before exiting toward the coronary sinus os again. This circuit determines the classic P wave morphology of typical flutter with an isoelectric/positive pattern in V1
and steep negative forces in the inferior leads. Reverse typical flutter utilizes the same circuit in the opposite clockwise direction. Since the circuit is constrained by the same anatomic barriers in all patients, in the absence of conduction-slowing medication, human AFL has remarkably constant cycle length around 200 to 240 ms.
and steep negative forces in the inferior leads. Reverse typical flutter utilizes the same circuit in the opposite clockwise direction. Since the circuit is constrained by the same anatomic barriers in all patients, in the absence of conduction-slowing medication, human AFL has remarkably constant cycle length around 200 to 240 ms.
Also known as the subeustachian isthmus and being formed by thick trabeculated muscle bundles extending from the bottom of the crista terminalis, the CTI is the narrow rim of tissue that lies between the os of the inferior vena cava (IVC) and the tricuspid annulus. This is the narrowest portion of the typical flutter circuit and the point where it is most vulnerable to interruption. Anatomic studies have shown the variability of CTI anatomy with the frequent presence of thick ridges and pouches.
Ablation of typical and reverse typical AFL (as well as a related rhythm called lower-loop reentry which rotates around the IVC) targets the CTI. Since the target is already known, and if the diagnosis has been made by the surface ECG morphology, patients do not need to be in flutter at the time of the procedure and ablation can occur in sinus or atrial paced rhythm. Using either a large tip or irrigated catheter, a linear lesion is created between the tricuspid annulus and the IVC. Flutter will usually terminate toward the end of this process if it was present at the start of the procedure. Acute termination and noninducibility of AFL is not a satisfactory endpoint however, as recurrence is common in this setting. Ablation is thus continued until conduction block is proven across the CTI from both medial and lateral to the linear lesion, the so-called bidirectional block.62 With the use of this endpoint, CTI-dependent AFL recurs in less than 4% of patients during long-term follow-up20 with an incidence of serious complications of less than 0.5%.20 This means that catheter ablation can be offered as an upfront alternative to less effective long-term suppressive drug therapy for typical AFL. Given the fact that most episodes of clinical typical AFL are induced by a burst of AF however, it should be remembered that patients with successful CTI ablation are still at risk for the development of AF over the subsequent years.63 This has implications for their long-term thromboembolic risk and thus close surveillance is warranted after AFL ablation.
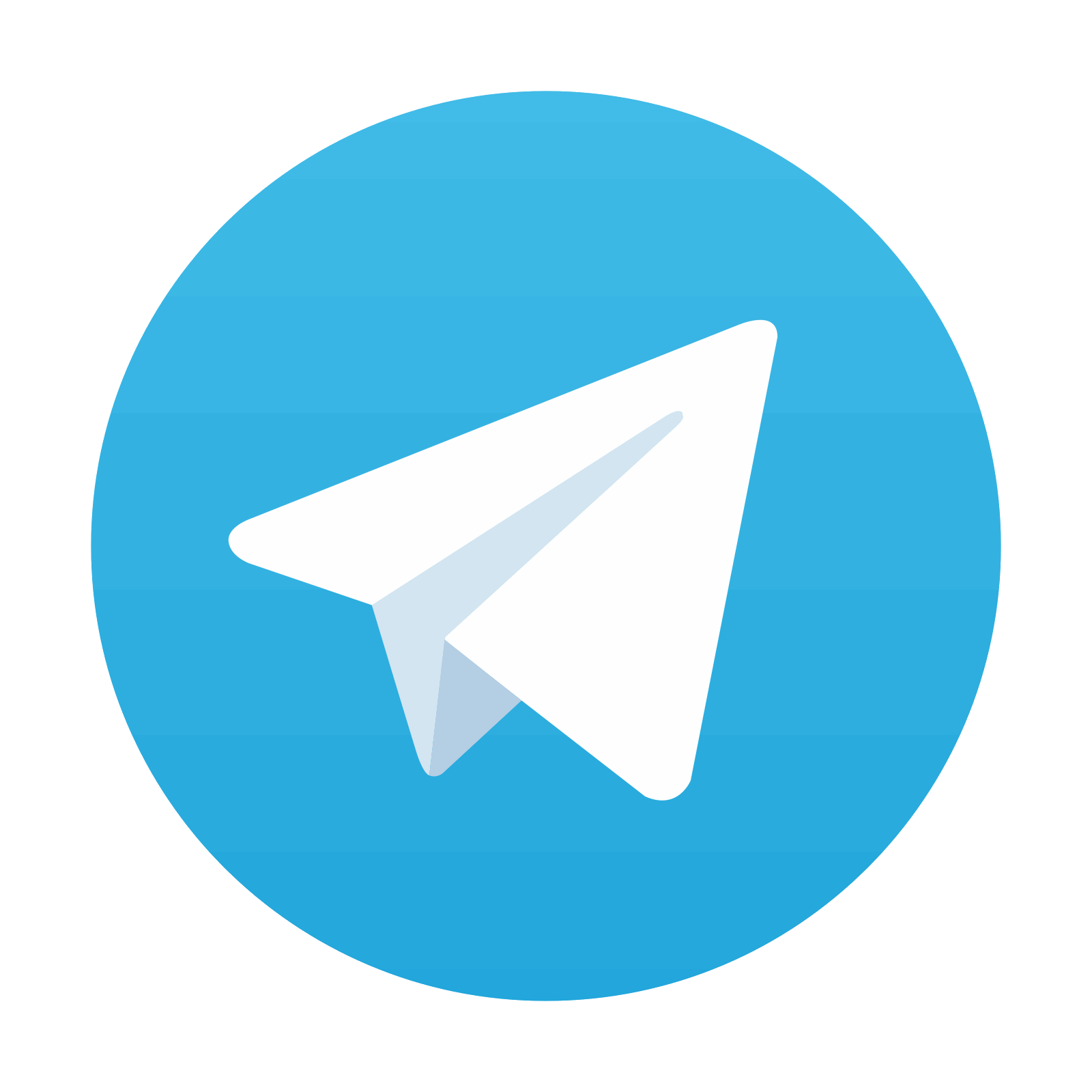
Stay updated, free articles. Join our Telegram channel

Full access? Get Clinical Tree
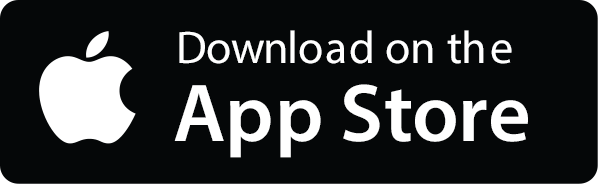
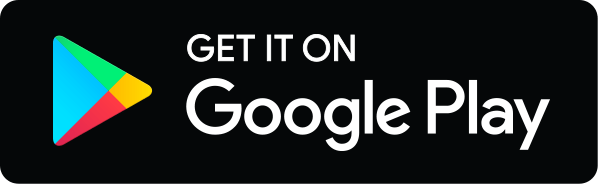