Intervention for Pediatric and Adult Congenital Heart Disease
Robert J. Sommer
The catheter-based treatment of congenital heart disease has been the traditional domain of pediatric interventional cardiologists. But the successes of both pediatric cardiac medicine and pediatric cardiac surgery over the past 40 years have resulted in a rapidly growing population of adults with corrected and palliated congenital cardiac lesions that few adult cardiologists have experience in managing. While most adult interventional cardiologists have limited training in the evaluation and transcatheter repair of these congenital lesions, pediatric interventional cardiologists who are expert in congenital heart disease, generally have little training in the commonly superimposed “adult” cardiology issues (such as pregnancy, hypertension, obesity, and coronary artery disease). This chapter focuses on a series of interventions for congenital heart disease that apply to both the pediatric and the adult population, noting procedural modifications that are required to accommodate a baby or an adult patient. Since knowledge of the physiologic and hemodynamic consequences of these lesions is at least as critical as knowing the steps of the procedures, a brief review of the underlying pathophysiology is also included in each section.
THE CONGENITAL CATHETERIZATION LABORATORY
Catheterization laboratories (cath labs) that perform procedures on patients with congenital heart disease differ from those treating mostly coronary disease. Most congenital procedures are best done in a biplane cath lab, as finding septal defects and complex surgically constructed pathways requires three-dimensional imaging. Case times are usually far longer than those of coronary cases, and the spectrum of equipment required for congenital cases is quite different. Because of the wide variety of congenital lesions and patient sizes, as well as the uncertainty as to when a particular catheter or device might be needed, a large number of items must be stocked. The laboratory administration thus must be willing to accept having some equipment expire unused rather than risk not having the critical catheter or wire available when it is needed.
In contrast to the early days of congenital catheterization, it is currently uncommon to perform purely diagnostic procedures. Echocardiography (transthoracic in children, transesophageal in adults), and now cardiac magnetic resonance imaging (MRI) are powerful noninvasive imaging modalities that allow the definition of the anatomy and assessment of both simple and complex physiology (including shunts, valvar dysfunction, and obstructions within the circulatory pathways). Instead, cardiac catheterization has become the preferred and minimally invasive way to perform repairs and palliations of straightforward congenital heart defects whose correction was previously limited to the operating room.
CONGENITAL OBSTRUCTIVE LESIONS
Obstructive Lesions of the Right Ventricular Outflow Tract
Obstruction to pulmonary blood flow is one of the most common and important abnormalities associated with congenital heart disease, and congenital interventions frequently involve relief of this problem. Congenital obstruction of the right ventricular outflow tract can occur in the muscular right ventricular outflow tract (subvalvar obstruction), at the valvar level, in the main pulmonary artery (MPA) (supravalvar obstruction), or at the level of the branch pulmonary arteries.
Although the level of obstruction can usually be determined noninvasively, each lesion has a unique hemodynamic pattern and angiographic appearance (see below). They have in common, however, increased afterload on the right ventricle (RV) myocardium, resulting in right ventricular hypertrophy and reduced diastolic compliance of the ventricle as a receiving chamber with a corresponding increase in right atrial filling pressures. The clinical symptoms associated with these lesions depend both on the degree of obstruction and the age of the patient.
Although the level of obstruction can usually be determined noninvasively, each lesion has a unique hemodynamic pattern and angiographic appearance (see below). They have in common, however, increased afterload on the right ventricle (RV) myocardium, resulting in right ventricular hypertrophy and reduced diastolic compliance of the ventricle as a receiving chamber with a corresponding increase in right atrial filling pressures. The clinical symptoms associated with these lesions depend both on the degree of obstruction and the age of the patient.
Subvalvar Pulmonary Stenosis
Subvalvar obstruction is usually muscular in nature and is not generally amenable to catheter intervention (Figure 35.1A). Residual or recurrent “infundibular” obstruction is most commonly found in patients who have undergone repair of tetralogy of Fallot. Double-chambered right ventricle (DCRV) also features hypertrophy of the infundibular muscle, causing it to closely appose the free wall of the RV in systole and narrow the outflow tract below the pulmonary valve. In children, this lesion may be associated with small membranous ventricular septal defects (VSDs) or membranous subaortic obstruction. Over time, the membranous ventricular septal defect may close spontaneously as the infundibular obstruction progresses, so that DCRV may present in the adult as an apparently isolated subvalvar obstruction.
Pulmonary valve annular hypoplasia may also present in the setting of significant right ventricular outflow obstruction and can be found in patients as residua of surgically corrected congenital heart disease (CHD). This lesion is not generally amenable to transcatheter intervention owing to the fibrous ring of the hypoplastic annulus that resists balloon dilation.
Supravalvar Pulmonary Stenosis
Except when scarring from previous surgical interventions produces narrowing of the MPA (i.e., following arterial reconstruction in neonatal transposition of the great arteries, or tetralogy of Fallot), supravalvar obstruction generally occurs at the sinotubular junction and is congenital. Sinotubular junction stenosis can be confused echocardiographically with valvar disease, because the normal leaflets are limited in their forward motion by the distal ridge of muscular tissue (Figure 35.1B), appear to dome, and exhibit turbulent flow at the leaflet tips on Doppler, just as is seen in valvar pulmonary stenosis (PS). Balloon dilation of supravalvar obstructions is largely unsuccessful owing to the muscular/elastic nature of the arterial wall in the MPA, and has been associated with MPA rupture. Stents have been placed successfully to relieve obstruction in the supravalvar region, but risk stenting open the pulmonary valve and causing severe insufficiency, most often a poor trade-off for moderate PS.
Valvar Pulmonary Stenosis
Valvar PS is a common congenital lesion. Similar in nature to congenital aortic stenosis (AS), it is typically caused by commissural fusion that precludes the leaflets separating fully (Figure 35.1C). The result is a diminished valve orifice/valve area resulting in increased RV afterload.
The natural history studies of isolated valvar PS in children indicate that a pressure gradient >50 mmHg is associated with poor long-term outcomes, including RV myocardial dysfunction, ventricular arrhythmia, and sudden death,1, 2, 3 whereas pressure gradients <30 mmHg are not associated with symptoms, changes in lifestyle, or life expectancy. As treatment shifted from surgical to catheter-based therapy in the 1980s, the indications for intervention have changed. Currently, any patient with a peak-to-peak valvar gradient ≥40 mmHg should be considered for balloon valvuloplasty.
Neonatal Critical Pulmonary Stenosis
Neonates with critical valvar PS, or with valvar pulmonary atresia, present with cyanosis. Desaturated blood returning to the right atrium (RA) can either enter the severely hypertrophied, sometimes diminutive RV chamber or flow right to left across the patent foramen ovale (PFO), as it did throughout fetal life. This shunt adds desaturated blood to the pulmonary venous return on the left side of the heart, accounting for the systemic hypoxemia. If the atrial level shunt is large enough, and resulting antegrade pulmonary flow is small enough, the patient will be dependent on the coexistence of a patent ductus arteriosus (PDA) to provide pulmonary blood flow. When the PDA begins to close (12 to 48 hours), the child becomes progressively hypoxemic, requiring prostaglandin E1 (PGE1) to reopen and to maintain ductal patency until an appropriate intervention can be performed.
Even after successful balloon intervention in the neonate (see below), the hypertrophied RV continues to present a diastolic impediment to forward flow, fostering a continued right to left shunt at the PFO, and ongoing systemic desaturation. When PGE1 is discontinued, and the PDA begins to close, pulmonary blood flow will be reduced, and systemic oxygen saturations will fall. As long as the infant maintains oxygen saturations greater than 70% and does not develop a systemic metabolic acidosis, PGE1 does not need to be restarted. Over a course of days to weeks, after elimination of the right ventricular afterload, the right ventricular myocardium thins and becomes more compliant. It becomes easier to fill the RV, right-to-left shunting diminishes, and the patient’s oxygen saturation normalizes. Rarely, ongoing RV noncompliance, often in combination with right ventricular and tricuspid valve (TV) hypoplasia, can result in persistent desaturation in the absence of significant residual PS, and may require closure of the PFO/atrial septal defect (ASD) to eliminate the right-to-left shunt.
Pulmonary Stenosis after the Neonatal Period
In most children, PS presents as an asymptomatic heart murmur. Gradients are followed noninvasively with Doppler echocardiography. It is important to note that the peak instantaneous echo gradient usually overestimates the peak-to-peak gradient measured in the cath lab (Figure 35.2) when considering the timing of the intervention in a child. Severe obstruction is associated with surprisingly few symptoms in young children. Rarely, children with severe PS may maintain patency of the PFO allowing right-to-left shunt at times of peak exercise (exercise-induced cyanosis). In patients who are cyanotic at rest, it is important to recognize that neither the echo nor catheter estimates of valve gradient accurately reflect the degree of obstruction, since only part of the cardiac output is traversing the valve.
Adolescent/Adult Patients
Valvar PS rarely presents de novo in the adolescent and adult population. The harsh systolic murmur invariably brings these patients to attention early in childhood. However, the lack of symptoms, and lesser gradients, in the pediatric age group, may delay early intervention. Mild degrees of obstruction are tolerated without issues for many decades but can occasionally be associated with new onset symptoms of exercise intolerance, breathlessness, and fatigue in the older adult. Classic signs of right heart failure—peripheral edema, jugular venous distension, and ascites—are rare. But the excessive right ventricular afterload limits RV systolic performance, thereby diminishing left ventricle (LV) preload and cardiac output during exertion. Adult PS patients tend to be more symptomatic than children with similar gradients, but we have seen patients in their eighth and ninth decades of life with very high gradients (>100 mmHg) and few symptoms.
Valvar PS in the adult may also be acquired as part of carcinoid heart disease.4 These valves become thickened and dense and more closely resemble dysplastic neonatal valves, whose resistance to flow is not related to valve leaflet fusion, but rather to the force required to push open the thickened leaflets. As is the case in the newborn disease, these thickened valves are generally not responsive to balloon valvuloplasty.
Pulmonary Valve Stenosis with Significant Regurgitation
When pulmonary valve stenosis or obstruction within a surgically placed RV to pulmonary artery (PA) conduit coexists with significant pulmonary regurgitation, the result is both a volume overload and afterload increase on the RV. In this setting, relief of the obstruction alone, may not alleviate the most severe hemodynamic derangement, the regurgitant flow, and may actually make it worse. Until recently, surgical intervention with valve replacement was the only alternative available to the patient.
Over the past decade however, beginning with the work of Bonhoeffer’s group in Europe,5,6 the development and subsequent clinical use of an implantable pulmonary valve has changed not only the interventional management of patients with residual postsurgical pulmonary valve disease, but also is reshaping many of the surgical paradigms for earlier management. With an excellent intervention available for replacement of the pulmonary valve inside of a surgical conduit, or inside the annular ring of a preexisting replacement tissue valve, surgeons are moving away from mechanical valves, and from complex RV outflow tract reconstructions, when a valved conduit can be more simply placed from the RV to the MPA. This is now done with the knowledge that the conduit placement may represent the last surgical intervention for some patients.
Since so many complex congenital malformations, including pulmonary valve atresia, tetralogy of Fallot, doubleoutlet RV, and some of the aortic valve malformations, require intervention for, or reuse of the native pulmonary valve, the need for later valve replacement is growing. This is particularly true in light of the growing recognition of the deleterious long-term effect of pulmonary valve insufficiency on the RV myocardium. The Melody Valve (Medtronics) is approved in the United States, and the Edwards-Sapien Valve, approved for aortic valve replacement7 is in clinical trials. Today, patients with tetralogy of Fallot who have had valved conduits or prosthetic valves placed, and patients who underwent the Ross procedure, where the pulmonary valve is autotransplanted to replace the diseased aortic valve and a valved conduit is used between the RV and the lungs, represent the two largest patient populations who are receiving these valves.
Careful evaluation of the underlying coronary anatomy is critical in determining the appropriateness of the intervention. When the conduit is placed on the anterior surface of the heart, coronary branches may pass directly beneath it, and may
be potentially compressed by placement of the stented valve and distension of the conduit. Evaluation and pretreatment of the valve landing site is critical for optimal valve longevity. Because the conduits sit directly beneath the sternum, there is often an element of compression of the conduit which is dynamic, and can lead to stent fracture (Figures 35.3 and 35.4). Prestenting the landing site of the new valve, potentially with several stents layered inside each other, has significantly improved the survival of the implant, minimizing stent fracture, which affected 23% of the initially reported series.8,9
be potentially compressed by placement of the stented valve and distension of the conduit. Evaluation and pretreatment of the valve landing site is critical for optimal valve longevity. Because the conduits sit directly beneath the sternum, there is often an element of compression of the conduit which is dynamic, and can lead to stent fracture (Figures 35.3 and 35.4). Prestenting the landing site of the new valve, potentially with several stents layered inside each other, has significantly improved the survival of the implant, minimizing stent fracture, which affected 23% of the initially reported series.8,9
Branch Pulmonary Artery Stenosis
Branch PA stenosis or hypoplasia may be acquired (e.g., either at sites of prior surgery, or from extrinsic compression) or may be congenital (i.e., tetralogy of Fallot). Numerous systemic congenital syndromes are also associated with branch PA stenosis/hypoplasia (Williams syndrome, congenital rubella, Alagille syndrome, and others). Branch PA stenosis is typically a hemodynamic burden that must be dealt with in children, but can be seen in adults as residue of earlier
congenital heart surgery or rarely as an isolated congenital lesion.10 Anatomy ranges from single stenotic areas, to multiple stenoses, to diffuse hypoplasia of the vessel. In contrast with other right-sided obstructive lesions, the branch PA stenosis not only increases RV afterload, but also results in maldistribution of the pulmonary blood flow with hypoperfusion of selected lung segments or of an entire lung, and overcirculation to others because of the parallel pathways available to the pulmonary blood flow. Indications for angioplasty include elevated right ventricular systolic pressure, hypertension in unaffected portions of the vascular bed, marked decrease in flow to an affected portion, and/or symptoms. In children, growth of the distal vessels depends on the blood flow to those segments. Segmental hypoperfusion in childhood is associated with poor growth of the affected PA, and is itself an indication for intervention.
congenital heart surgery or rarely as an isolated congenital lesion.10 Anatomy ranges from single stenotic areas, to multiple stenoses, to diffuse hypoplasia of the vessel. In contrast with other right-sided obstructive lesions, the branch PA stenosis not only increases RV afterload, but also results in maldistribution of the pulmonary blood flow with hypoperfusion of selected lung segments or of an entire lung, and overcirculation to others because of the parallel pathways available to the pulmonary blood flow. Indications for angioplasty include elevated right ventricular systolic pressure, hypertension in unaffected portions of the vascular bed, marked decrease in flow to an affected portion, and/or symptoms. In children, growth of the distal vessels depends on the blood flow to those segments. Segmental hypoperfusion in childhood is associated with poor growth of the affected PA, and is itself an indication for intervention.
Quantitative lung perfusion scans, and more recently MRI/MRA, have been extremely useful in the ongoing assessment of these patients. Flow to each individual lobe can be quantified, yielding information about relative severity of individual stenotic lesions. This can help direct therapy prior to arrival in the cath lab and avoid unnecessary catheter/wire manipulation during what are often lengthy procedures.
Percutaneous Balloon Pulmonary Valvuloplasty
Percutaneous pulmonary valvuloplasty in children was first reported by Semb et al.11 in 1979. However, the static balloon technique, reported by Kan et al.12 in 1982, was the first to be applied widely. Results have demonstrated the safety and effectiveness13, 14, 15, 16 of this technique and have established it as the treatment of choice for children and adults with isolated pulmonary valve stenosis.
Pediatric Technique
Echocardiographic evaluation is critical to successful outcome prior to intervention. It defines both the degree of obstruction as well as the valve morphology, allows extremely accurate measurement of the pulmonary valve annulus, and can rule out any associated defects. Balloon dilation catheters are now available on catheter shafts as small as 3F, allowing pulmonary balloon valvuloplasty even in premature infants under 2 kg.
After administration of appropriate anesthesia, femoral venous access is obtained and a balloon-tipped angiography or end-hole catheter is used to perform the right heart catheterization. In the absence of associated defects, the PA saturation is used to estimate predilation cardiac index (Fick calculation). In most cases, femoral arterial access is not required, as complications from arterial access probably far outweigh the complications of the valvuloplasty especially in small children under 5 kg. The need for heparin administration (50 to 100 U/kg), in a purely right-sided catheter procedure is unclear. An endhole catheter is advanced across the valve to a branch PA and a pressure pullback is performed (Figure 35.1C). The peak-to-peak, and mean gradients across the pulmonary outflow are measured. Right ventricular angiography is then performed using both lateral and cranial-angled AP projections to localize the valve and confirm the echocardiographic annulus size by measuring the valve diameter at the level of the valve hinge points. A balloon diameter 1.2 to 1.4 times that of the annulus is selected, since the use of oversized balloons in pulmonary valvuloplasty has been shown to produce optimal results in children. In larger patients, with an annulus size >20 mm, modifications in technique are required (see Section “Adult Patients”). A balloon length of 2 to 3 cm, depending on patient size, is adequate in most children and avoids some of the potential complications of right ventricular outflow tract trauma and injury to the TV.
A balloon end-hole catheter can be floated to the distal right or left PA (angled, torquable catheters may also be used), and a stiff exchange-length guidewire is placed in the branch PA, taking care not to injure the small distal branches of the PA. The sheath and catheter are removed, and the desired valvuloplasty balloon is introduced over the guidewire. Although some have advocated the introduction of a “naked” balloon through the groin, we prefer the use of a short venous sheath large enough to accommodate the appropriately sized balloon. Once the balloon catheter is centered on the pulmonary valve, the position can be adjusted quickly using a series of very-low-pressure partial inflations to look for the valve “waist.” When in the appropriate position, the balloon is inflated rapidly, with mild traction to prevent forward motion, until the waist disappears most often with a “popping” sensation. The balloon is then immediately deflated. The pop of the valve corresponds to the tearing of the stenotic valve commissures. We like to record the fluoroscopic images of this inflation, so that the result can be immediately reviewed, especially if there is any question of the effectiveness of the dilation.
After successful opening of the valve, the balloon will usually jump forward with forward flow, which must be distinguished from the forward “squirting” of a balloon that has been positioned too far into the PA, since the latter indicates that the valve has not been effectively dilated. In patients with dysplastic or thickened pulmonary valves, there will be no pop as the balloon is inflated to full pressure, but only a gradual resolution of the waist with increasing balloon pressure and a return of the waist as balloon pressures fall. As mentioned, these valves are rarely amenable to balloon valvuloplasty.
If a suboptimal result is obtained, repositioning and immediate reinflation may help. A monorail-type angiographic catheter can be advanced over the wire to assess the residual gradient, without removing the guidewire. Alternatively, a larger-lumen end-hole catheter (i.e., a guide catheter) with a Y adapter (Tuohy-Borst valve) can be advanced over the wire to perform a pullback, measuring pressure from the side port of the Tuohy-Borst as the catheter is withdrawn across the valve. In this way, residual pressure gradients can be measured and accurately localized to either the valvar or infundibular level (see Section “Complications”). Residual valvar gradients of more than 20 to 30 mmHg are unusual and suggest suboptimal balloon size or position and warrant repeat dilation with advancement of a potentially larger dilation catheter over the guidewire that is still in place in the PA.
Age-Related Modifications of the Procedure
In neonates, access for the procedure can be obtained at the umbilical vein, provided the ductus venosus in the liver has not closed. This route is readily accessible and avoids injury/obstruction of the femoral vein. In older patients, internal jugular and subclavian approaches are also acceptable for the procedure. Percutaneous transhepatic access has also been used.
With severe PS, with a closed PDA, the catheter across the tiny valve lumen may completely or virtually completely obstruct flow. In this setting, we prefer to perform the RV angiogram prior to crossing the valve to minimize the potential for hemodynamic compromise. Once the valve is crossed,
the catheter is removed quickly, leaving only the guidewire (to minimize obstruction to flow). In neonates, it is almost never possible to pass a balloon-tipped catheter through the valve, making our catheter of choice a torquable 4F or 5F end-hole catheter with a multipurpose or Berenstein curve. This catheter is manipulated through the TV and flipped up into the RV outflow tract, and either a 0.014-inch torque-control guidewire or a small hydrophilic guidewire can be used to probe for the valve orifice. Once the valve is crossed, the wire is positioned either in a branch PA or through the ductus arteriosus into the descending aorta where it could be snared from the descending aorta to stabilize the position further, if needed.17 Most often, in this setting, we will first use a smaller balloon on a smaller catheter shaft to predilate the valve, followed by an oversized balloon to finish the procedure.
the catheter is removed quickly, leaving only the guidewire (to minimize obstruction to flow). In neonates, it is almost never possible to pass a balloon-tipped catheter through the valve, making our catheter of choice a torquable 4F or 5F end-hole catheter with a multipurpose or Berenstein curve. This catheter is manipulated through the TV and flipped up into the RV outflow tract, and either a 0.014-inch torque-control guidewire or a small hydrophilic guidewire can be used to probe for the valve orifice. Once the valve is crossed, the wire is positioned either in a branch PA or through the ductus arteriosus into the descending aorta where it could be snared from the descending aorta to stabilize the position further, if needed.17 Most often, in this setting, we will first use a smaller balloon on a smaller catheter shaft to predilate the valve, followed by an oversized balloon to finish the procedure.
Valvar pulmonary atresia also presents with cyanosis in the neonate. In these babies, patency of the ductus arteriosus is required to provide pulmonary blood flow. In some cases, the size of the pulmonary valve annulus, the RV chamber volume, and the TV annulus may be sufficient to allow handling of the normal pulmonary blood flow. In these patients, several techniques have been used to perforate the atretic valve, including stiff guidewires, transseptal needles, or radiofrequency ablation—the most common choice today.18, 19, 20 Once the valve is perforated and a wire advanced into one of the branches or down the descending aorta (through the PDA), balloon dilation proceeds as if for critical neonatal PS. In some cases, ductal stenting can also be performed to maintain adequate pulmonary flow, as the RV becomes more compliant over time.
Adolescent/Adult Technique
Percutaneous balloon valvuloplasty in adults is similar to what is described above for younger children. The principle difference is that the valve annulus is larger, and owing to the need for balloon diameter oversizing to 120% to 140% of the valve annulus, balloons of 25 mm or larger are often required. There are three solutions to this size issue:
1. Custom balloons are available in sizes greater than 30 mm. With a balloon of this size, no real differences are required in the technique, but the balloons have longer inflation times, longer deflation times, lower burst pressures, and require larger sheath sizes.
2. Double-balloon technique. A second venous access is obtained and a balloon-tipped end-hole catheter is passed to the distal PA. A second stiff exchange-length guidewire is placed across the valve. The perimeter of the combined balloons is selected to be 20% to 40% larger than the measured annulus,21 and the balloons are inflated simultaneously. The double-balloon technique allows the use of two smaller sheaths but requires additional venous access and additional personnel; it is also more technically challenging, requiring accurate positioning of two balloons during simultaneous inflation.
3. In these cases, we prefer the use of an Inoue balloon for adolescent/adult pulmonary valvuloplasty.22 After hemodynamic evaluation and RV angiography, a 14F sheath is exchanged over a wire and a 0.032-inch stiff exchange guidewire is positioned in the branch PA. An Inoue balloon is selected with a diameter 1.2 times the valve annulus. The balloon is stretched/slenderized, and passed over the wire to the RA. At that point, the balloon is softened by retraction of the metal support rod, and the distal portion of the balloon is inflated slightly to help float the catheter through the valve. If there is difficulty in manipulating the Inoue balloon through the RV to the PA, a long 14F Mullins sheath can be passed over a wire into the PA, and the Inoue can be advanced through the long sheath (the sheath may need to be cut shorter to accommodate the Inoue length). Once positioned in the main PA, the valvuloplasty is performed exactly as a mitral valvuloplasty. A Y adapter can be attached directly to the back of the Inoue, and pressure can be measured from the sideport during pullback, over the wire. Advantages of the Inoue technique include the ability to upgrade the balloon size without having to change out the catheter, and minimizing the risk of TV or branch PA injury with the short length and positional accuracy of the balloon. Principle disadvantages are the large sheath size required and the higher cost of the Inoue compared with other balloon dilation catheters.
Complications
Acute Subvalvar (Infundibular) Obstruction
With severe valvar PS, particularly in older children and adults, concentric right ventricular hypertrophy is present universally. When the afterload at the valve is acutely removed, a hypercontractile RV outflow tract may create a dynamic subvalvar muscular obstruction, which has been termed the “suicide right ventricle.” The total gradient across the outflow tract may actually be higher after dilation than it was prior to the balloon dilation. It is critical to recognize the difference between residual valvar obstruction and resulting subvalvar reactive obstruction, so as not to perform unnecessary additional valve dilations. A careful pullback pressure recording performed over a wire (as outlined earlier) is the best way to determine the level of residual obstruction. With an intact atrial septum, if the subvalvar obstruction is severe enough, cardiac output may fall acutely. In patients with the potential for right to left shunt at the atrial level, hypoxemia will ensue. Acute treatment of these patients is much like that with left-sided hypertrophic obstructive cardiomyopathy. Volume loading should be combined with beta and/or calcium channel blockers to reduce myocardial contractility. Over the course of several weeks to months, as the hypertrophy of the outflow tract muscle recedes with reduction of the RV afterload, subvalvar obstruction will resolve.23
Other complications are rare and include TV injury, as a result of wire tension on the TV leaflet during inflation or in pulling the partially deflated balloon back through the TV.
Pulmonary insufficiency can be seen after successful dilation, but is usually well tolerated acutely. Long-standing valvar regurgitation, however, may lead to RV dilation and dysfunction in adults. In children, long balloons may rarely injure/rupture the curved RV outflow tract as the balloon straightens, and a long balloon advanced or propelled too far into one of the branch PAs may cause injury at that site. Finally, the wire tracking through the TV can pinch or damage the atrioventricular node, resulting in high-degree atrioventricular block. In our experience, however, more than 94% of pulmonary valve procedures in all age groups were uncomplicated, with most complications clustered in the smaller infants.
Pulmonary insufficiency can be seen after successful dilation, but is usually well tolerated acutely. Long-standing valvar regurgitation, however, may lead to RV dilation and dysfunction in adults. In children, long balloons may rarely injure/rupture the curved RV outflow tract as the balloon straightens, and a long balloon advanced or propelled too far into one of the branch PAs may cause injury at that site. Finally, the wire tracking through the TV can pinch or damage the atrioventricular node, resulting in high-degree atrioventricular block. In our experience, however, more than 94% of pulmonary valve procedures in all age groups were uncomplicated, with most complications clustered in the smaller infants.
Over more than two decades, pulmonary balloon valvuloplasty has been shown to be an extremely safe and effective therapy, in all age groups. Acute and long-term gradient reductions are comparable to surgical interventions, whereas complications, including pulmonary regurgitation, are less prevalent than after surgery.24 Balloon valvuloplasty is a curative procedure for most patients and should be considered the procedure of choice for valvar PS with a transvalvar gradient of >40 mmHg at any age, in symptomatic adults with gradients >30 mmHg, and in neonates with critical PS.
Balloon Angioplasty for Branch Pulmonary Artery Stenosis
Balloon angioplasty for hemodynamically important branch PA lesions can be accomplished with small peripheral vascular balloon catheters. Specially designed high-pressure balloon catheters or bladed cutting balloons may be used for more resistant lesions. Placement of intravascular stents has become the treatment of choice in older children and adults who have completed or nearly completed their growth. Particularly in the proximal, more muscular branches of the pulmonary tree, stents more reliably improve vessel size, overcoming the recoil of these elastic vessels, and reduce the need for oversized balloons. With increased experience demonstrating that later stent reexpansion is possible/safe, stents are now being used in even the youngest patients in critical situations.
Technique
Branch PAs may be dilated from any venous access, depending on the catheter course required. Often, access to the left PA can best be achieved from the femoral approach, while a superior approach may be the easiest to get to the right PA. Following a Glenn shunt, the only access to the branch PAs would be from above. In the case of an aortic to PA shunt, the PAs would need to be accessed from the femoral artery.
After heparinization, and the placement of a small arterial catheter for blood pressure monitoring, right heart hemodynamics are measured, and the magnitude and location of gradients in the PAs are determined. In patients with symptoms of severe right ventricular failure, creation of an ASD (to maintain cardiac output) prior to dilation may decrease morbidity and mortality, by maintaining cardiac output (LV preload) even with the balloon inflated in the lungs. Pulmonary angiograms should include selective biplane injections in each lung and in affected lobes or segments. Selective catheterization of the lung segments is best accomplished using a torquable end-hole catheter and a floppy-tipped torque wire. Steerable/deflectable catheters, used frequently by electrophysiologists for ablation procedures, are also available but may be too short in larger patients. Once satisfactory wire position is attained, an angiographic monorail catheter is the tool of choice to image and to assess the severity of the stenosis. Alternatively, a larger-lumen angiographic catheter may be passed over the wire, with a Y adaptor at its end, to allow angiography and pressure measurement from the sideport. Either technique allows pressure measurements, angiograms, and dilations to be performed without losing wire position. Lower-volume, selective injections in the affected lobes almost always yield superior images compared to injections in the central PA. Prior to dilation, a stiffer exchangelength wire should be passed to the largest vessel distal to the stenosis to support balloon positioning. Use of the largest vessel also minimizes the risk of rupture/vessel injury of small distal vessels with balloon inflation.
The ideal balloon has a low profile, a short distal tip, and a high maximal inflation pressure. The balloon diameter is chosen to be two to four times the diameter of the lesion but not more than two times the diameter of the normal vessel on either side.25, 26, 27 The balloon is inflated until the waist disappears or until maximum inflation pressure is reached. Inflation times range from 10 to 60 seconds, depending on the response of the waist and how well the cardiac output is maintained. Unlike dilation of the semilunar valves, in which all output from the ventricle is eliminated during inflation, perfusion of the other lung/lobes will maintain cardiac output and will allow longer inflation times. Like with coronary angioplasty, successful dilation generally results in tearing of the intima and media.28
Following dilation, the balloon catheter is exchanged again over the guidewire for a monorail catheter, and the lesion is reassessed hemodynamically and angiographically. Successful dilation is often accompanied by an increase in pressure distal to the angioplasty site, and results in both a decrease in proximal pressures and a decrease in the gradient across the area. Angiograms are repeated to measure the diameter of the stenosis and to look carefully for tears and aneurysms that may preclude further balloon dilation. For this reason, distal lesions are generally dilated prior to proximal lesions, and more severe stenoses are dilated prior to milder ones.
Results
The criteria for successful dilation have been arbitrarily defined as an increase in diameter of >50%, an increase in flow in the affected segment of >20%, and/or a decrease of >20% in the systolic right ventricular-to-aortic pressure ratio. Using these criteria, the early success rate using low-pressure
balloons was approximately 60%.27,28 The use of cutting balloons and high-pressure balloons with inflation pressures greater than 20 atmospheres increased success rates to 75%.29 The success rate for postoperative stenoses is higher than for congenital stenoses, which tend to be much more resistant to dilation. There is an incidence of subsequent restenosis following balloon angioplasty of approximately 15%.29 Complications have included death in approximately 1% of patients secondary to PA rupture or reperfusion pulmonary edema. Aneurysms occur in 3% of dilations and are most common in small vessels distal to the stenosis. Although the success rate using low-pressure balloons has changed little over the years, the complication rate has decreased owing primarily to improved technique. The use of high-pressure balloons does not seem to have significantly altered the complication rate. Balloon angioplasty at surgical sites should be used with caution in patients less than 4 to 6 weeks after surgery to minimize the risk of vessel rupture.
balloons was approximately 60%.27,28 The use of cutting balloons and high-pressure balloons with inflation pressures greater than 20 atmospheres increased success rates to 75%.29 The success rate for postoperative stenoses is higher than for congenital stenoses, which tend to be much more resistant to dilation. There is an incidence of subsequent restenosis following balloon angioplasty of approximately 15%.29 Complications have included death in approximately 1% of patients secondary to PA rupture or reperfusion pulmonary edema. Aneurysms occur in 3% of dilations and are most common in small vessels distal to the stenosis. Although the success rate using low-pressure balloons has changed little over the years, the complication rate has decreased owing primarily to improved technique. The use of high-pressure balloons does not seem to have significantly altered the complication rate. Balloon angioplasty at surgical sites should be used with caution in patients less than 4 to 6 weeks after surgery to minimize the risk of vessel rupture.
Use of Bladed Cutting Balloons
Later experience with bladed cutting balloon catheters has shown improved outcomes for the treatment of what were previously considered undilatable lesions. Small studies, under compassionate use protocols, have shown significant improvement in vessel size using oversized cutting balloons that cut through intimal and medial layers.30,31 After cutting-balloon inflation, subsequent use of high-pressure balloons and/or intravascular stents has further improved outcome with success rates reported in up to 92% of vessels.32
Use of Intravascular Stents
Intravascular stents were first used for elastic/resistant branch PAs in 198926, 27 and are now the most commonly used in CHD. Although balloon angioplasty remains the treatment of choice in peripheral lesions and in infants/small children (growth issues), stent implantation in the PA bed has become first-line therapy for proximal obstructions, lesions owing to surgical distortion, external compression, inadequate results of balloon angioplasty, and obstructive intimal flaps following balloon angioplasty. Infants who undergo surgical repairs such as the arterial switch for transposition of the great arteries, repair of tetralogy of Fallot with branch PA plasty, and palliations such as shunts or PA banding may have acute postoperative obstructions that create hemodynamic instability or place the obstructed lung at risk for long-term hypoperfusion. Stent usage in the immediate postoperative period, as an alternative to repeat surgical intervention, can be lifesaving and is more reliable than balloon angioplasty alone. Since early stent use in the branch PAs, technology has improved both in the stents themselves and in the delivery balloons. The use of stents has improved the success rate of branch PA interventions to >90%.
Most experience with stent implantation in branch PAs is with the balloon expandable Palmaz and Genesis stents (Johnson & Johnson, New Brunswick, NJ), though newer stent designs may have significant advantages including trackability, conformability to vessel course, reduction in balloon and vessel damage owing to smoother device edges, diminished stent shortening with expansion, and improved visibility with alternative materials. Innovations in balloon design include a marked reduction in catheter shaft diameter and slip-/scratch-resistant balloon surfaces (to reduce the incidence of the stent slipping off or puncturing the balloon). The BIB (balloon-in-balloon) balloon dilation catheter system (Nu-Med, Hopkinton, NY) is being used for many indications and helps minimize the risks of stent malposition.
Heparinization and prophylactic antibiotic use is recommended in these typically long procedures. Predilation with a balloon is optional but is not necessary for most branch PA lesions. Stent and balloon sizes are selected for the individual lesion. The stent is crimped onto the balloon and can be delivered using one of two techniques. With a standard approach, an oversized long sheath or guiding catheter is passed over a stiff exchange-length guidewire (with a short floppy tip), such that the sheath tip is distal to the lesion to be stented. The balloon/stent combination is then advanced over the guidewire through the sheath to the implantation site. Alternatively, the front-loading technique involves passing the balloon catheter fully through the sheath outside the body, crimping the stent onto the balloon, and pulling it back into the front of the sheath before introducing this assembly over the wire. Combined techniques are also used in which the long sheath is advanced over the wire to the inferior vena cava (IVC), the stent then mounted on the balloon, and advanced over the wire to the tip of the sheath prior to advancing the entire system over the wire to the target lesion. The stent is deployed as described in Chapter 31. Antiplatelet therapy is the norm, for a period of 3 to 6 months to prevent in-stent thrombosis. For patients with normal pulsatile flow in the PAs, aspirin alone should be sufficient. For patients with nonpulsatile flow (bidirectional Glenn shunt or Fontan), dual antiplatelet therapy or warfarin is warranted, though there is little data available on which to base such a decision. There is a very low rate of restenosis in the branch PAs except for the relative stenosis that develops as the patient grows.32
Stents in Extracardiac Conduits
Stents may also be used to extend the life of surgical conduits inserted between the heart and the branch PAs. With rapid growth of the infant, such conduits may kink and develop intraluminal obstruction. Balloon angioplasty alone in this setting has been largely unsuccessful because the conduit will reassume its kinked course on balloon deflation. In several patients, we have been able to hold off conduit replacement for several years using this approach, removing the stent with the explanted conduit at later surgery. This may allow for placement of an adult-sized conduit at the next surgery, and future placement of transcatheter valve implants (see above). For patients with obstructions in full-sized conduits, a pulmonary valve implant would be the current procedure of choice.
OBSTRUCTION OF THE LEFT VENTRICULAR OUTFLOW TRACT
Anatomy/Physiology
Similar to obstructions of the right ventricular outflow tract, congenital obstruction of the left ventricular outflow tract can occur at the subvalvar level, at the valvar level, at the supravalvar level, or in the aortic arch itself (coarctation of the aorta). The increased afterload on the LV myocardium results in concentric left ventricular hypertrophy and reduced diastolic compliance of the ventricle as a receiving chamber with a corresponding increase in left atrial and pulmonary venous pressures. The severity of patient symptoms is proportional to the elevation of left ventricular filling pressures. Patients may present with dyspnea on exertion when symptoms are mild and/or with orthopnea, syncope, or sudden death with ventricular dysfunction. Most children tolerate large degrees of left ventricular outflow tract obstruction without symptoms, presenting with a murmur alone, but symptoms become more common in the older child and adult.
Transcatheter Therapy for Left-Sided Obstruction
Subaortic stenosis encompasses a spectrum of disorders ranging from simple membranous obstructions of the subaortic area, to fibromuscular tunnel obstructions, to the more familiar hypertrophic cardiomyopathy. Like subvalvar lesions of the right side, subaortic obstructions are primarily a surgical issue. Membranous obstructions of the left ventricular outflow were treated with balloon angioplasty early in the interventional era,33,34 with limited success and with routine recurrence of obstruction.
Fibromuscular tunnel obstructions are generally not amenable to catheter intervention. Stents have been used in a few patients with critical postoperative obstructions, with very limited success, high morbidity, and a significant incidence of stent failure.
For the more common hypertrophic cardiomyopathy with muscular subaortic obstruction, alcohol ablation of septal tissue in adults has been widely adopted as an alternative to surgical muscle resection. There is no significant experience with this technique in children and adolescents, because septal hypertrophy is rarely clinically apparent before puberty and continues to develop during adolescence and young adulthood. Dual-chamber pacing has been reported to alleviate the degree of obstruction in younger patients, but with comparative studies is less effective in adults than either surgery or alcohol ablation.35
Supravalvar AS typically occurs at the sinotubular junction, as a congenital lesion. This is the pathognomonic lesion for Williams syndrome, a genetic deletion syndrome associated with developmental delay, abnormalities of calcium metabolism, and a diffuse arteriopathy. This lesion should also be treated surgically because the elastic properties of the tissue at that site make balloon angioplasty ineffective, and the use of a stent in this position risks entrapping aortic valve leaflets with resulting aortic regurgitation.
Valvar Aortic Stenosis
Balloon aortic valvuloplasty for congenital AS in a child was first reported in 1983.36 It has been performed subsequently in large numbers of patients with both congenital and acquired valvar stenoses.36, 37, 38, 39, 40, 41, 42 In acquired calcific valve stenosis, acute relief of gradient is possible. However, the rapid rate of restenosis, the risk of cerebral embolization, the risk of disrupting the valve leaflets and causing severe valvar insufficiency, along with unpredictable and suboptimal degrees of obstruction relief has left balloon valvuloplasty for calcified valves as an emergency option only, when surgical valve replacement is not an option. The recent successes of transcatheter aortic valve replacement7,43 are changing the paradigms for treatment of older adults with severe aortic valve disease.
In children, adolescents, and young adults with congenital valvar AS, however, balloon valvuloplasty remains an excellent alternative to surgical valvotomy or to valve replacement, since the pathology involves more commissural fusion and less leaflet rigidity seen in adult patients with senescent and densely calcified aortic valves.
Neonatal Critical Aortic Stenosis
Physiologically, critical AS is a different entity from severe AS in older children, since the valve may be virtually atretic and the left ventricular cavity may be moderately to severely hypoplastic. Unlike hypoplasia of the right ventricle in critical PS (in which right atrial blood may cross the PFO to maintain LV preload and cardiac output), the size of the left ventricle has a direct impact on survival. LV hypoplasia, reduced LV compliance, and reduced emptying in the face of extraordinary afterload lead to increased left atrial and pulmonary venous pressures. While the PFO (or ASD) may act as a pop-off valve for the left atrium (LA), reducing pulmonary venous pressures and congestion, any blood that shunts from the LA to the RA, reduces LV preload, and as a result further reduces left ventricular output. Low cardiac output and pulmonary venous congestion may be incompatible with postnatal life. If LV hypoplasia is a concern, the alternative is to assign these patients to a stage I palliation for hypoplastic left heart syndrome. A retrospective analysis of a group of patients with critical AS undergoing surgical valvotomy or balloon dilation at Boston Children’s Hospital led to a scoring system (based on echocardiographic measurement of left-sided structures) that can be used to triage such patients.44
The neonate with critical AS may be asymptomatic immediately after birth because of the normal remnants of the fetal circulation. Left atrial return crosses the PFO to the RA, rather than entering the LV. The volume-loaded RV ejects blood into the MPA, where flow is distributed between the lungs and the systemic circulation via the ductus arteriosus, based on the relative resistance of each pathway. If a normal
blood volume reaches the aorta, there are no clinical problems. However, as pulmonary resistance falls and as the ductus arteriosus starts to close, RV blood preferentially flows to the lungs. A corresponding fall in systemic blood flow results in diminished tissue oxygen delivery, anaerobic respiration at the cellular level, and profound metabolic acidosis. Reestablishing and maximizing systemic flow is the key to resuscitating the acidotic newborn. Prostaglandin is required to reopen the ductus, and elevation of pulmonary vascular resistance is desirable (minimize FiO2, allow pCO2 to rise).
blood volume reaches the aorta, there are no clinical problems. However, as pulmonary resistance falls and as the ductus arteriosus starts to close, RV blood preferentially flows to the lungs. A corresponding fall in systemic blood flow results in diminished tissue oxygen delivery, anaerobic respiration at the cellular level, and profound metabolic acidosis. Reestablishing and maximizing systemic flow is the key to resuscitating the acidotic newborn. Prostaglandin is required to reopen the ductus, and elevation of pulmonary vascular resistance is desirable (minimize FiO2, allow pCO2 to rise).
When most of the systemic flow arises from the ductus arteriosus, rather than the LV, the measured valvar pressure gradient across the stenotic valve is not a meaningful number, as the flow across the valve can approach zero. Prior to ductal closure, critical AS is manifested by right-to-left systolic flow at the ductus arteriosus with equal systemic arterial desaturation in all four limbs.
Pediatric Technique
Using routine sedation, a femoral vein and artery are entered percutaneously and the patient is heparinized. The venous catheter is used to measure right heart pressures and cardiac output (when no shunts are present) before and after dilation. In older patients, aortic valves can be dilated from the femoral vein using a transseptal approach, but this risks entrapping/stenting open/injuring the mitral valve, and the retrograde approach from the femoral artery remains the most common technique. The typical method for crossing the stenotic aortic valve from the aorta is to advance the soft end of a straight wire out of a steerable catheter (multipurpose or Judkins Right curve) and use it to probe for the valve orifice. We often use a hydrophilic guidewire, as the reduced friction of its surface allows for more rapid in and out movements, but probing must be done gently to avoid perforating a cusp or damaging the coronary arteries. When the LV is entered, a transvalvar gradient is measured by simultaneously recording pressure from the catheter and from the femoral sheath (one French size larger than the catheter). An aortogram may be done to define the anatomic landmarks if the time for crossing becomes prolonged. We do not routinely do a baseline aortic angiogram, as the amount of aortic regurgitation should have been documented by echo as part of the precatheterization evaluation. A pigtail catheter is exchanged over a wire, a left ventriculogram may be performed and the aortic annulus can be measured at the hinge points of the valve.
In contrast to the pulmonary valvuloplasty, the balloon diameter is chosen to be only 75% to 90% of the annulus diameter. Animal and clinical studies demonstrate that aortic valvuloplasty with a balloon/annulus ratio greater than 1.0 is more likely to be associated with aortic regurgitation.45,46 A double-balloon technique was used in the past to allow for the use of smaller sheaths, when the annulus was larger, and there was concern for femoral artery injury in small children. However with balloons on shafts as small as 3F and 4F today, there is little indication for such an approach. The pigtail catheter is exchanged for the balloon dilation catheter, which is centered across the valve, inflated and deflated rapidly, and pulled back to the descending aorta. The gradient and the cardiac output are remeasured following dilation, and an aortogram is performed to look for aortic regurgitation. If the residual gradient is >55 mmHg and an aortogram shows no or only mild regurgitation, a larger balloon may be used. It is far better, however, to leave a residual gradient than to cause significant aortic insufficiency in the small child, as surgical backout options are limited (Ross procedure).
It can often be difficult to keep the balloon positioned in the valve during inflation, against the force of left ventricular ejection. A stiff catheter shaft, long balloon, and extra stiff exchange wire will help stabilize the position, or the balloon can be advanced so that it lies along the top of the aortic arch rather than around the underside of the arch. An increasingly common technique, suggested by Cribier,47 involves the use of a transvenous pacing catheter positioned in the RV to rapidly pace the ventricle during balloon inflation to reduce ventricular ejection and provide a more stable balloon position. This technique is a critical component of the transcatheter aortic valve implantation procedure.
Technique for Critical Neonatal Aortic Stenosis
Before the catheterization begins, the baby’s hemodynamic status must be optimized. We perform this catheter procedure with the baby intubated and paralyzed and receiving a prostaglandin infusion. The FiO2 is turned down to 21%, and pCO2 is allowed to rise to the mid-40s to increase pulmonary vascular resistance and maximize systemic flow across the PDA. Body temperature is carefully monitored.
The umbilical artery can usually be used in the first week of life. Catheter manipulation is more difficult from the umbilical artery owing to the inferoposterior loop in its course before it enters the descending aorta, but its use avoids damage to the femoral artery in these very small infants. Surgical cutdown at the carotid artery for catheter access has also been employed. This approach simplifies crossing the valve48 because of the straight path to the valve from the neck. As with older patients, a transseptal approach can be used from either the femoral or umbilical vein, with no puncture required since all neonates have a PFO. But this approach is rarely used since it carries a much higher risk of mitral valve injury than in the older population.
Results/Complications
Balloon valvuloplasty for congenital AS has been an effective palliation in the pediatric population with excellent gradient reduction and increase in valve area. But the procedure is complicated by the development of significant aortic regurgitation in 10% to 30% either immediately or within weeks of the procedure (inversely related to balloon/annulus ratio); femoral arterial complications in 30% to 40% (inversely related to the size of the patient), and restenosis.49, 50, 51 Survival with
freedom from reintervention was seen in only 50% of patients at 14.4 years in one cohort of 269 patients51 and in 60% at 12 years in a group of 74 patients.50 Transient left bundle branch block occurred in 15% and ventricular arrhythmias requiring cardioversion in 3%.51 In a 25-year review of balloon aortic valvuloplasty at Texas Children’s Hospital,42 death or transplant was significantly correlated with poor baseline LV systolic function, and the need for recurrent intervention was significantly higher in neonates than in older patients (34% versus 9%).
freedom from reintervention was seen in only 50% of patients at 14.4 years in one cohort of 269 patients51 and in 60% at 12 years in a group of 74 patients.50 Transient left bundle branch block occurred in 15% and ventricular arrhythmias requiring cardioversion in 3%.51 In a 25-year review of balloon aortic valvuloplasty at Texas Children’s Hospital,42 death or transplant was significantly correlated with poor baseline LV systolic function, and the need for recurrent intervention was significantly higher in neonates than in older patients (34% versus 9%).
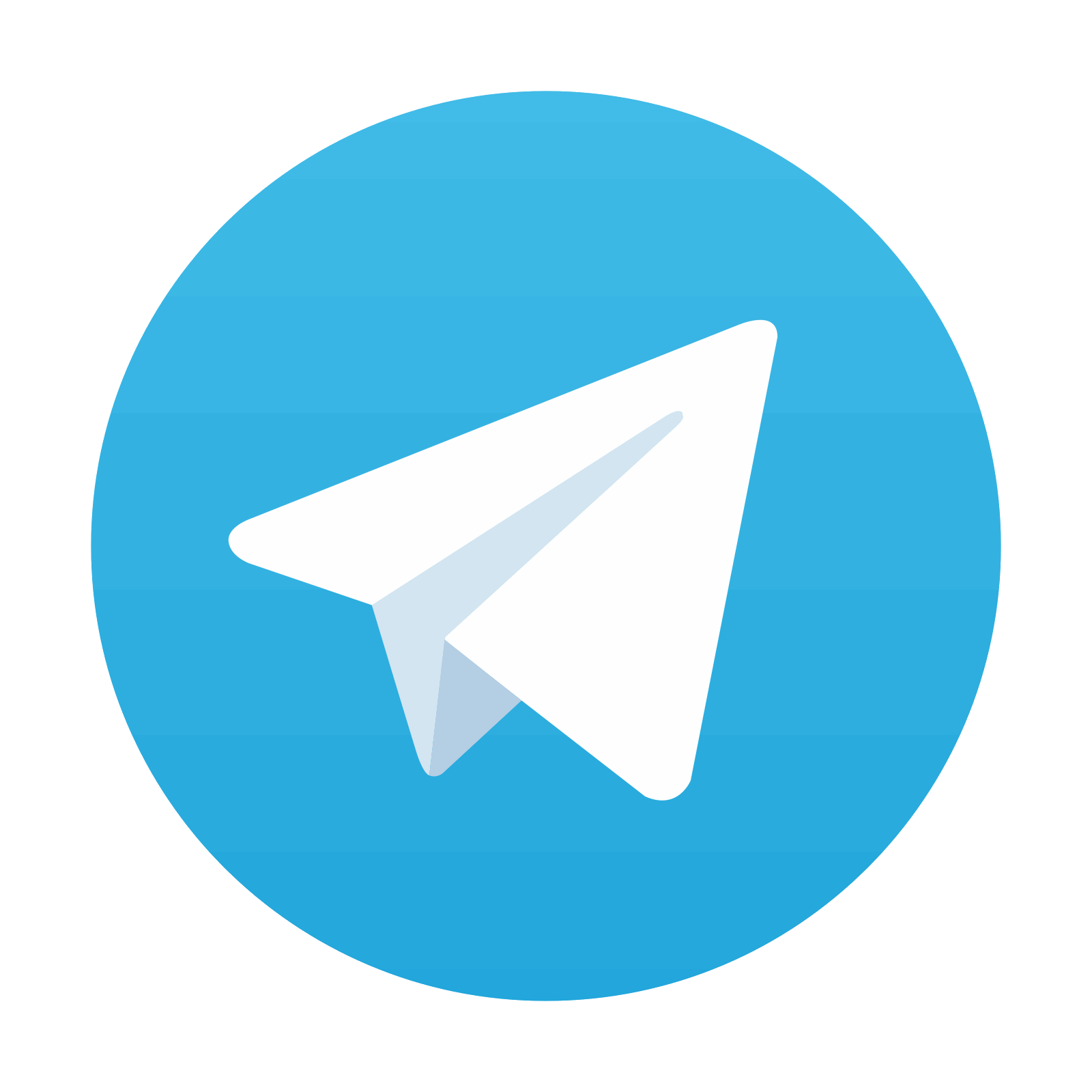
Stay updated, free articles. Join our Telegram channel

Full access? Get Clinical Tree
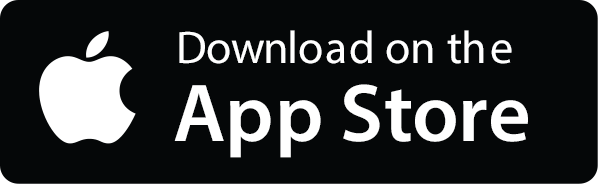
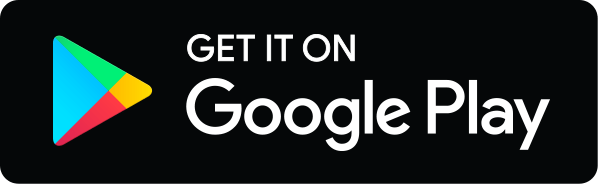
