Chapter 22 Imaging in the Electrophysiology Laboratory
Intracardiac Echocardiography
Comparison of Mechanical and Phased-Array Intracardiac Echocardiographic Transducers and Systems
Phased-array systems use either a linear array of crystals (Viewmate, St Jude-EP Med Systems, Berlin, NJ; AcuNav, Acuson Division, Biosense Webster, Diamond Bar, CA) parallel to the catheter body or radially arranged crystals at the catheter tip (JOMED). The first two use 64 to 128 crystal elements and operate at frequencies ranging from 5 to 10 MHz. These can operate at variable (Acuson) or four different frequencies (Viewmate) to produce a sector scan parallel to the long axis of the catheter. The catheter body ranges from 7 to 10 Fr and is typically deflectable over an arc of 90 degrees. These systems produce two-dimensional and M-mode images with high resolution over depths up to 12 cm. This permits imaging of the left-side chambers from the right heart without trans-septal puncture. In general, these systems have become more popular in clinical electrophysiological procedures. More detailed features of each of these systems are shown in Figure 22-1. Platforms can provide more enhanced imaging features such as harmonic imaging for producing improved gray-scale image presentation, adaptive color Doppler to select the optimal frequency for improved resolution, and tissue Doppler imaging to assess direction and timing of myocardial function and pulsed-wave tissue Doppler for velocity mapping during cardiac and vascular imaging. Fusion of two-dimensional ICE images into a static electroanatomic map using the CARTO system (Biosense Webster) is now commercially available (discussed later and elsewhere in this text).
Three-dimensional reconstruction has now been performed with offline and real-time reconstruction of serial images and by overlay of electrical activation sequences on the images. Simon et al initially demonstrated electroanatomic mapping by using serial sections from a rotational ICE transducer and electrical recordings from a basket catheter in the right atrium in patients with atrial flutter, which were processed offline (Figure 22-2, A).1 With this approach, they could demonstrate macro–re-entrant activation sequences in common atrial flutter on a three-dimensional anatomic ICE image. Smith et al used a phased-array system to reconstruct a real-time three-dimensional anatomic ICE image with up to 60 volumetric scans per minute and superimposed activation maps on these images. In a sheep model, they demonstrated right and left atrial intracardiac structures, including pulmonary veins and the right ventricle, and pacing sequences could be accurately visualized in the reconstructed anatomy.2 Pulmonary veins, antral regions, and right atrial structures have been reconstructed in early three-dimensional image efforts (see Figure 22-2, A).3 Knackstedt and colleagues reported a close to real-time technique using an AcuNav ICE catheter with a motorized sector scan and reconstruction of serial two-dimensional images. Animal and clinical testing validated the visualization of anatomic structures.4 Okumura and colleagues applied online imaging and offline reconstruction of ICE imaging of pulmonary veins by using a pullback technique during ablation procedures in 29 patients. The images were computer reconstructed to visualize the entire length of pulmonary veins with a three-dimensional full motion image being developed. Radiofrequency (RF) lesions could be visualized and the extent of ablation between extensive and segmental ablation procedures assessed. The superior pulmonary veins were visualized in virtually all patients; the ablation sites were identified, pulmonary vein stenosis excluded, and comparable lesions sizes noted with both approaches.5
Baseline Image Acquisition Using Intracardiac Echocardiography
Passage of the ICE catheter into the right atrium allows the initiation of baseline imaging of cardiac structures.6 Baseline imaging requires visualization of the right atrium, the tricuspid valve, and the right ventricular inflow tract in the first image set. This is usually accomplished from a low to mid-right atrial placement of the phased-array transducer with the catheter body parallel to the spine. Tricuspid valve motion and trabeculation of the right ventricle and its inflow tract are identified. The coronary sinus ostium, the triangle of Koch, and the crista terminalis can also be imaged by sweeping and torquing the transducer viewing sector. The transducer can then be deflected and retroflexed to a septal imaging view in this location. On occasion, the transducer may need to be advanced or withdrawn to achieve good septal imaging. The fossa ovalis and its muscular margins are clearly seen. Aortic root and valve imaging is usually best achieved in a more superior right atrial location with retroflexion of the ICE transducer. Transducer imaging depth may be adjusted to achieve imaging of the left atrial pulmonary vein and LAA as well. Left atrial wall thickness and change in tissue image may be used to assess the extent of ablation, as can microbubble formation. The transducer may have to be deflected to visualize the ostia of the pulmonary veins and the appendage. It is important to torque the ICE catheter body to fully scan the left atrium from the superior to the posterior aspect to the inferiorly located mitral valve to look for thrombus, intracavitary echoes or “smoke,” and septation such as in cor triatriatum. Pulmonary vein antra and ostia require care and imaging depth adjustment for optimal visualization from the right atrium. In contrast, rotational ICE catheter systems require a systematic scan from the superior to inferior right atrium for right atrial structure identification. Trans-septal puncture is not required for phased-array systems, but rotational ICE catheters need to be placed in the left atrium for adequate imaging, and the phased-array catheter may need trans-septal placement for mitral valve or left ventricular interventions. Right ventricular placement is useful for left ventricular imaging as well as in ventricular wall motion analysis, optimization of cardiac resynchronization therapy, and smoke or thrombus identification.
Anatomic accuracy has been judged by comparing ICE imaging to computed tomographic (CT) angiography. Jongbloed et al noted a high degree of concordance between the two methods for left atrial structural anatomy such as pulmonary vein anatomy and ostial and antral configurations and dimensions.7 One prospective clinical trial has assessed ICE imaging compared with transesophageal echocardiography (TEE).8 In unpaired analyses, an atrioseptal aneurysm was detected by TEE in 4 (9%) of 45 patients with TEE and in 5 (15%) of 34 patients with ICE. In paired analyses, there was no atrioseptal defect identified by either technique. The percentage concordance in paired analysis for the presence of a patent foramen ovale was 100% and 96% for atrioseptal aneurysms with the two techniques, respectively. Ascending aortic plaques were more often visualized by TEE, but descending aortic plaques were more often visualized by ICE. However, concordance between the two methods was quite modest, especially when plaque size was considered. Dense smoke, thrombus, or aortic plaque can potentially influence interventional procedures and should be carefully examined.
Use of Intracardiac Echocardiography to Guide Electrophysiological Procedures
Evaluation of the Left Atrium and Left Atrial Appendage
In the Intra-Cardiac Echocardiography–Guided Cardioversion to Help Interventional Procedures (ICE-CHIP) study, comparison with TEE showed no significant difference in the presence of spontaneous echo contrast between ICE and TEE during left atrial imaging, but there was a significantly greater incidence of spontaneous echo contrast in the appendage detected with TEE compared with ICE (P = .005).8 Percentage concordance for the presence of spontaneous echo contrast was 65% for the two techniques for the left atrium and 60% for the two techniques of the LAA. Dense contrast was seen in the left atrium in 12.5% of ICE studies and 16.9% of TEE studies. In the LAA, dense contrast was less common with ICE (ICE, 5.3% vs. TEE, 15.7%). The LAA is visualized with both techniques, and the presence of spontaneous echo contrast in both the left atrium and the LAA is clearly seen. Intracardiac thrombus was uncommonly seen with both techniques (TEE, 6.9% vs. ICE, 5.2%) with a percentage concordance for the presence or absence of thrombus of 97% in the left atrium and 92% in the LAA. Probable thrombus in the left atrium was actually detected more frequently by ICE, but the presence of thrombi in the appendage was more frequently detected or reported with TEE. In view of this, ICE should be complemented with TEE for LAA thrombus, particularly if limited views and poor-quality images are obtained. Other approaches, such as coronary sinus placement of the ICE probe, have been suggested to improve appendage imaging, particularly if ablation or cardioversion of AF is contemplated.9
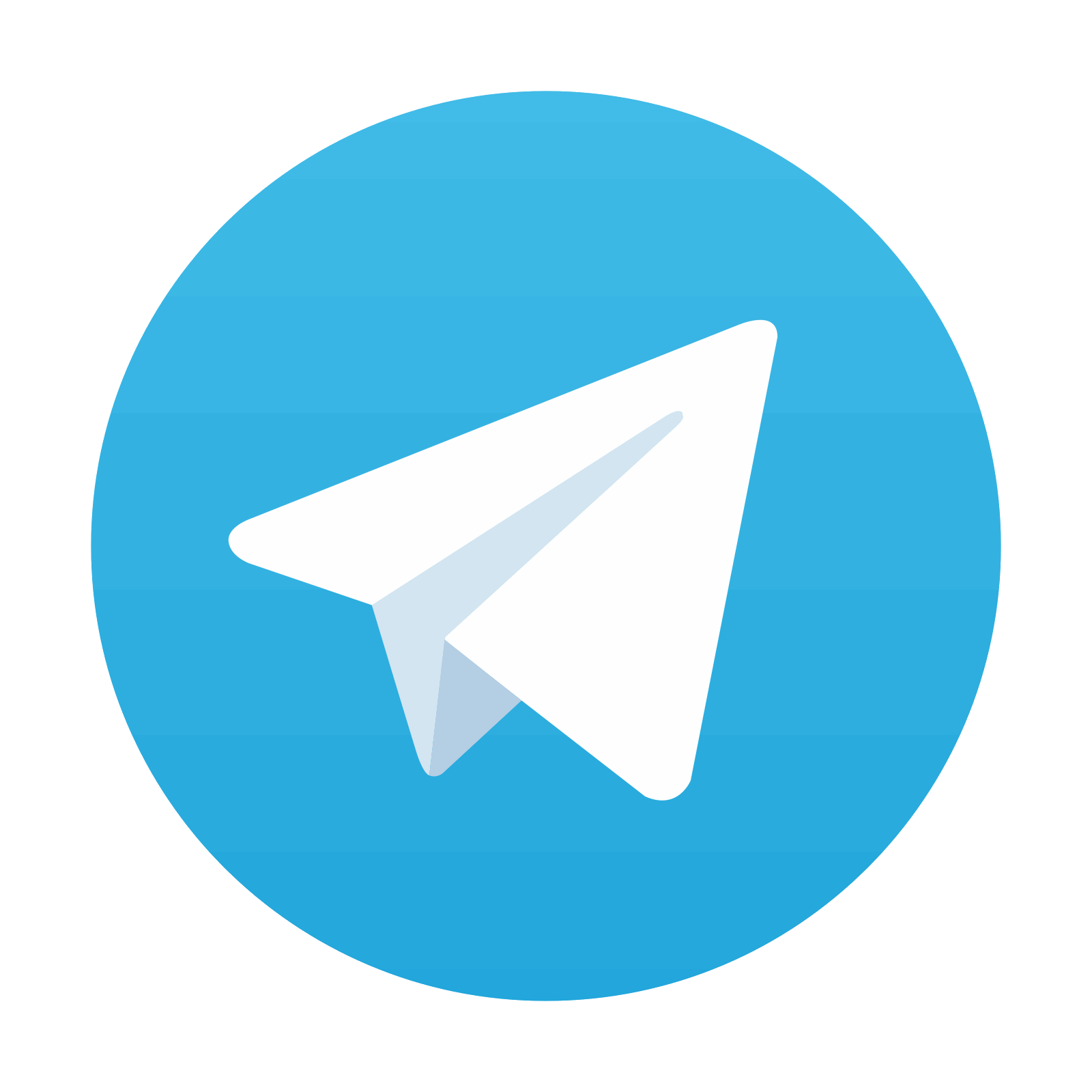
Stay updated, free articles. Join our Telegram channel

Full access? Get Clinical Tree
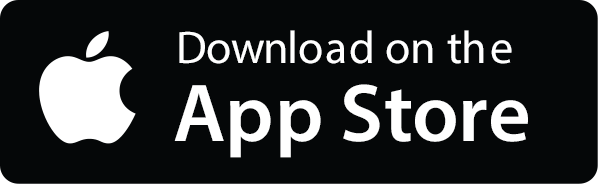
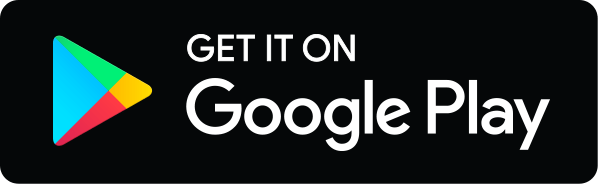