Chapter 35 Hemodynamic Aspects of Cardiac Pacing
Heart Rate: Restoration of Normal Chronotropic Function
Cardiovascular Physiology
Cardiac output (CO) is the product of heart rate (HR) and stroke volume (SV), calculated as the difference between left ventricular (LV) end-diastolic (ED) and end-systolic (ES) volumes. HR is mainly under the influence of the autonomic nervous system and circulating catecholamines. LVED volume depends on the (1) circulating volume, (2) cardiac filling pressures, (3) contributions of atrial systole, and (4) ventricular relaxation, and LVES volume depends on (1) cardiac afterload and (2) ventricular contractility (Figure 35-1). The Frank-Starling law correlates the ventricular filling pressures with ventricular systolic ejection volumes as a function of the LV systolic performance (Figure 35-2).1 The respective contributions of these variables to cardiovascular performance are influenced by age; physical fitness; the presence, type, and severity of underlying heart disease; and the prescription of pharmaceuticals. In healthy individuals, during exercise, up to a 300% increase in CO is mostly contributed by an increase in HR and more modestly to an increase in stroke volume (SV).2 On the one hand, the contribution of the increase in SV is more important in endurance athletes. In the presence of LV systolic dysfunction, on the other hand, the increase in SV during exercise is limited by a reduced contractile reserve, and the increase in CO is even more dependent on variations in HR.
Chronotropic Dysfunction
Chronotropic Incompetence
The term chronotropic incompetence (CI) describes an insufficient HR relative to the metabolic needs of the organism, during exercise or under stress caused by emotional disturbance, pain, and so on. The definition of CI has not been standardized. It has been defined as the inability to reach, during an exercise test, a maximal HR more than 75% to 80% of the theoretical maximal HR calculated by Astrand’s formula (220 – Age). Others consider that the absolute peak HR during exercise must be more than 100 or 120 beats/min.3 Wilkoff determined the HR response to exercise in a mathematical model, which includes age, resting HR, intensity of exercise, and functional capacity (FC) in the equation:
The incidence of CI varies with the definition applied, though it may reach 60% among permanently paced patients. Its origin is atrial or ventricular. Sinus node dysfunction (SND) is the most frequent cause of atrial CI, observed in 25% to 40% of cases, and the incidence increases with age.4 A few patients presenting with permanent atrial fibrillation maintain a normal chronotropic function during exercise. However, they have inappropriate tachycardia, bradycardia, or both in alternans. The severity of CI in patients presenting with high-degree AV block increases with age and with the increasingly distal location of a block along the His-Purkinje system. Rarely dissociated from disorders of impulse formation or propagation, CI may be associated with autonomic dysfunction, as well as with myocardial ischemia.5 Further investigation is warranted if myocardial ischemia is suggested by clinical presentation, particularly since its incidence is threefold higher among patients with coronary artery disease.
Optimal Heart Rate
Lower Ventricular Pacing Rate
In the early days of cardiac pacing, Sowton et al studied the optimal lowest pacing rate by measuring CO and left and right filling pressures in patients presenting with complete AV block.6 The highest CO and lowest filling pressures were associated with pacing rates between 55 and 90 beats/min and a mean of 71 beats/min. CO/ventricular pacing rate curves showed roughly two distinct profiles. Flat curves (Figure 35-3, left) were most often associated with normal myocardial contractility and a stable CO as HR increased. Peaking curves (see Figure 35-3, right) were often observed in the presence of LV dysfunction, showing greater variations in CO with changes in HR. At the fastest HR, CO levels fell, reflecting the shortened diastolic filling time and alteration in LV compliance.
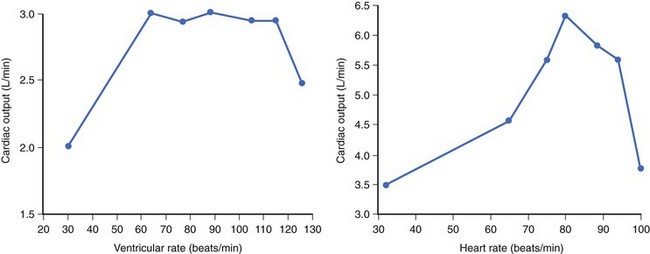
Figure 35-3 Left, Flat curve. Right, Peaked curve.
(From Sowton E: Haemodynamic studies in patients with artificial pacemakers, Br Heart J 26;737–746, 1964.)
Maximal Ventricular Pacing Rate
The upper ventricular pacing rate, whether atrial tracking driven or sensor driven, must also be tailored on the basis of the patient’s habitual activities and underlying cardiovascular status. An excessively slow upper pacing rate causes exercise-induced chronotropic insufficiency, and an excessively rapid pacing rate may be deleterious. Kindermann et al ascertained the theoretical, optimal, maximal pacing rate corresponding to the increase in maximum VO2 (oxygen rate) measured during cardiorespiratory exercise testing in patients with a normal or less than 45% LV ejection fraction (EF), the majority of whom were paced in DDD mode.7 The mean optimal pacing rate was 86% of the theoretical maximal HR in patients with a normal LVEF, and 75% in patients with a depressed LVEF. It is noteworthy that programming of the upper pacing rate was excessively high in the majority of patients. Furthermore, in most patients, maximal VO2 rapidly reached a plateau during exercise testing, regardless of systolic LV function. According to Fick’s principle, a VO2 that remains stable despite an increase in HR (in presence of a stable arteriovenous O2 difference) indicates a decrease in SV.
Preserving Atrial Function and Atrioventricular Synchrony
Atrial Function
The hemodynamic importance of atrial function varies highly among individuals. In healthy subjects, the contribution of atrial systole to ventricular SV is approximately 20% at rest and 30% during exercise. The contribution of atrial systole to CO during vigorous effort is gradually supplanted by HR. However, the relative importance of atrial systole increases with age, with abnormal ventricular diastolic function, and within certain limits, with the deterioration of LV systolic function.8
Atrioventricular Synchrony
A proper AV synchronization is key for the efficacious hemodynamic contribution of atrial systole. Conversely, complete AV dissociation is hemodynamically detrimental. Atrial systole occurring when the mitral valve is closed activates (1) atrial and pulmonary neurohumoral stretch receptors, (2) vagal activity, and (3) the production of atrial natriuretic peptide. These effects may cause a fall in systemic arterial pressure and a variety of manifestations known as pacemaker syndrome when occurring in permanently paced patients. It has been described in approximately 20% of patients paced in the VVI/VVIR mode, whose atrial activity is sinus, though it may occur in any pacing mode in the presence of AV dyssynchronization. AV synchronization is electrical and mechanical, left and right. While electrical and mechanical dyssynchronies usually coexist, their correlation is not systematic (see Atrial Conduction Delay). “Electrical” AV dyssynchrony manifests as an abnormal PR interval or AV dissociation on surface ECG, and “mechanical” AV dyssynchrony is defined as a LV diastolic filling time less than 40% of the cardiac cycle, accompanied by an abnormal transmitral Doppler profile. In the case of cardiac pacing, left AV mechanical synchrony is hemodynamically the most important and is preserved by programming an appropriate AV delay. The main determinants of the AV delay are AV conduction time, intra-atrial and inter-atrial conduction times, and intraventricular and interventricular (VV) conduction times.
Atrial Conduction Delay
The intra-atrial and inter-atrial conduction delays are measured from the onset of the P wave to (1) the para-Hisian atrial ECG (normally 30 to 60 ms), and (2) to the distal coronary sinus atrial ECG (normal 60 to 90 ms), respectively. Prolonged atrial conduction delays may cause left AV dyssynchrony with a foreshortened mechanical AV delay, apparent on mitral Doppler as a short LV filling period and truncated A wave. The main causes of prolonged atrial conduction delays—apparent on surface ECG as a more than 120-ms P-wave duration, notching of the P wave, and a foreshortened segment between the end of the P wave and the onset of QRS (Figure 35-4)—are (1) slowing of intra-atrial conduction due to heart disease, and (2) a latency of paced atrial capture. The latter is responsible for greater conduction delays than is apparent during sinus rhythm and mandates the routine programming of a 30 ms to 40-ms longer paced than sensed AV delay. The latency of atrial capture can be considerably amplified by several factors, including type and location of the pacing lead and its interface with the myocardium, pacing rate, abnormal electrolyte or metabolic status, the effect of medications, and myocardial disease. Heart diseases most often associated with anomalous atrial conduction are valvular (rheumatic mitral disease, in particular), hypertrophic, ischemic, and hypertensive. Programmers allow a choice of separate AV intervals for sensed P waves versus paced P waves in order to offset this phenomenon. While left atrial dilation is often associated with prolonged atrial conduction time, this correlation is low, except in the case of rheumatic mitral valve disease.9
Prevention and Management of Atrial Conduction Delay
Late Atrial Sensing and Cardiac Resynchronization Therapy
The clinical effects of fused spontaneous and paced QRS in recipients of CRT systems remain uncertain. Indirect arguments suggest that fusion complexes are not necessarily adverse.11 In limited studies, at least a similar hemodynamic benefit was observed in patients at rest in whom resynchronization was achieved by LV stimulation alone, with fusion via the right bundle branch, or by biventricular stimulation.12 Furthermore, the absence of first-degree AV block predicts a response to CRT. Either the presence of AV block is a prognostic factor associated with more severe heart disease or its absence is associated with a higher likelihood of fusion or “concealed resynchronization.”13 Nevertheless, pending further studies, the current recommendation is to avoid or limit biventricular simulation with fusion to the extent possible.
Atrioventricular Synchrony During Exercise
During exercise, sympathetic activity facilitates AV nodal conduction, and a shortening of AV delay that is inversely proportional to the HR is observed. Implantable pacemakers are able to reproduce this phenomenon in patients with AV block.14 The rate-adaptive AV delay shortens AV delay, usually linearly with acceleration of the HR, up to the shortest programmable value.15 This offers several benefits: (1) improvements in hemodynamic function and cardiopulmonary performance while preserving proper AV synchrony during exercise16; (2) ability to increase the maximal synchronous HR during exercise by shortening TARP; and (3) subjective improvement in the quality of life by the adaptation of the AV delay during exercise.17
Conflicting data have been reported regarding the programming of hemodynamically optimal AV delays in recipients of CRT systems during effort, probably reflecting heterogeneous patient characteristics, including degree and type of dyssynchrony, lead positions, and underlying heart disease.18 The current recommendation is to deactivate the rate-adaptive AV delay in the absence of AV conduction disorder.
Ventricular Conduction Delay and Atrioventricular Synchrony
An additional delay is inserted between left atrial and LV activation in cases of marked slowing of intraventricular or VV conduction with left bundle branch block morphology, most often observed in the context of advanced heart disease. Despite the absence of PR interval abnormality of surface ECG (Figure 35-7), unequivocal left AV mechanical dyssynchrony is present on ultrasound Doppler examination (Figure 35-8). Although conventional DDD pacing with a short AV delay can correct LV filling (transmitral Doppler), it should not be programmed in patients with HF because of the potential adverse effects of RV pacing. Moreover, this pacing increases only left heart filling, whereas biventricular stimulation restores AV synchrony on both sides of the heart (see Figure 35-17) as well as intraventricular synchrony and improves clinical outcomes.
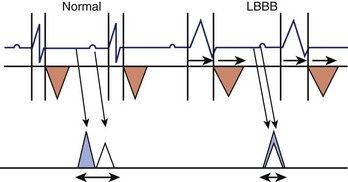
Figure 35-8 Effect of left bundle branch block (LBBB) on AV synchrony. Left ventricular outflow tract (orange triangles) and mitral Doppler (blue triangle, E wave; white triangle, A wave) are shown during the cardiac cycle. LBBB is responsible for an increased systolic and decreased diastolic interval (see also Figure 35-15) with fusion of A and E waves. The P-R interval is unchanged.
(Modified from Cazeau S, Gras D, Lazarus A, et al: Multisite stimulation for correction of cardiac asynchrony, Heart 84:579–581, 2000.)
Atrioventricular Optimization in DDD/DDDR Pacing
The purpose of an optimal AV delay is to guarantee (1) the maximal contribution of the atrium to the diastolic LV filling, (2) a long diastolic filling period, (3) a short isovolumetric contraction period, and (4) the maximal SV. Empirical programming is suboptimal because of the wide variability among patients in optimal AV delay, caused by marked interindividual differences in electrical and electromechanical intervals in the atria (intra-atrial and inter-atrial conduction times) and ventricles (VV conduction delay, in particular). In case of an excessively long AV delay, LV contraction occurs well after atrial systole, while the mitral valve is passively semi-closed, causing (1) fusion of E and A waves of the filling transmitral Doppler flow and (2) diastolic mitral regurgitation (Figure 35-9). An excessively short AV delay causes the LV contraction to close the mitral valve before the end of ventricular filling (truncated A wave). AV delay is optimized mostly in recipients of CRT systems, whereas in dual-chamber pacing, it is reserved for the rare patients presenting with symptoms attributed to AV dyssynchrony. Several methods are applicable, depending on the choices and practices of the various centers (Table 35-1). Echocardiography and programming algorithms are most often used because of their wide availability, ease of use, and low cost. Other methods are mostly investigative and will not be discussed in detail in this chapter (see Table 35-1).
Table 35-1 AV Optimization Methods
Methods | Description |
---|---|
ECHOCARDIOGRAPHY | |
Optimization of Left Ventricular Filling | |
Start with long AV, then shortening by 20 ms until truncated A wave, then lengthening every 10 ms to avoid truncated A wave | |
Optimization of Left Ventricular Systole | |
OTHERS | |
Vopt, Optimal AV delay; AV, AV delay; long QA, interval between Q of the electrocardiogram to end of mitral Doppler A wave with long AV delay; short QA, interval between Q of the electrocardiogram to end of mitral Doppler A wave with short AV delay; VTI, velocity-time integral.
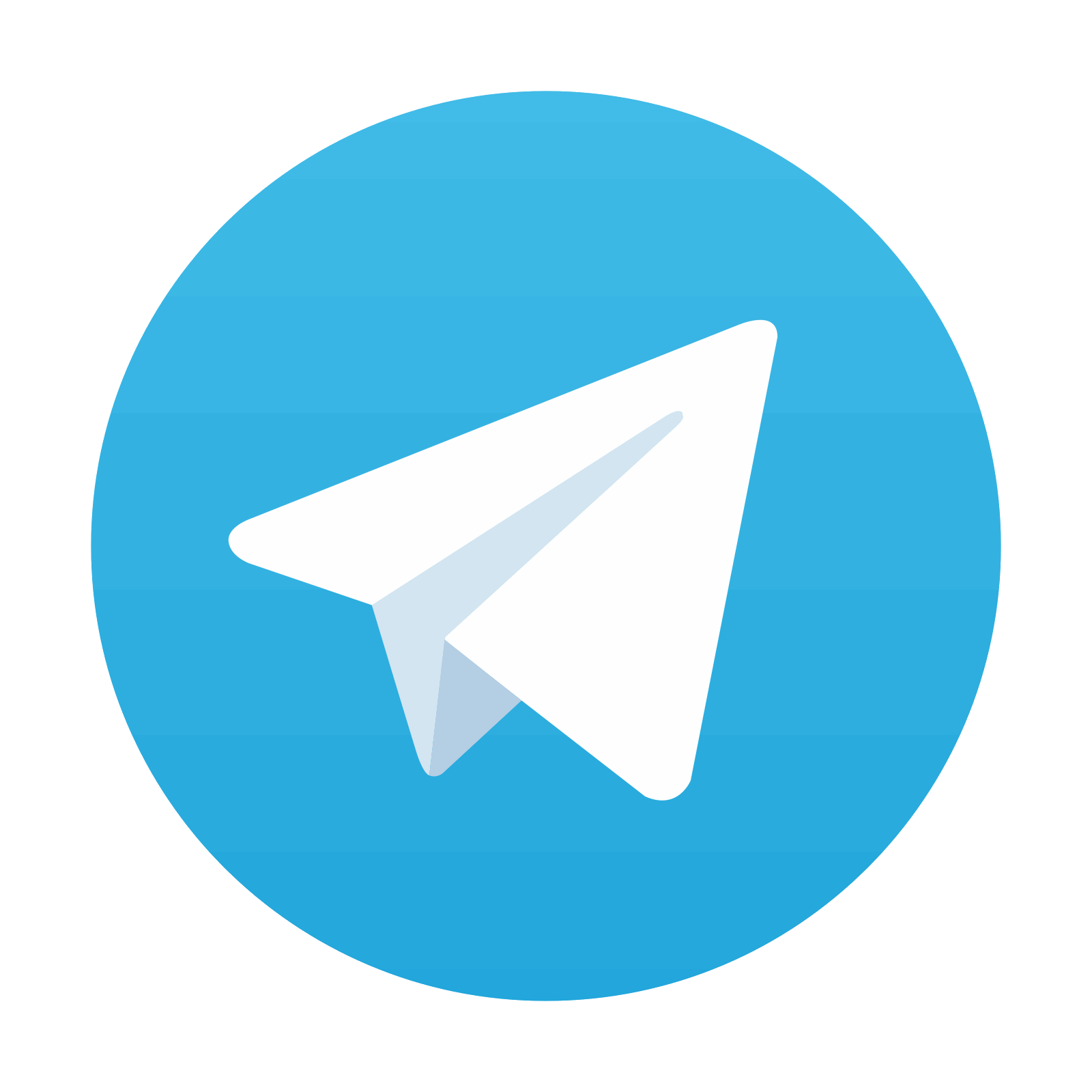
Stay updated, free articles. Join our Telegram channel

Full access? Get Clinical Tree
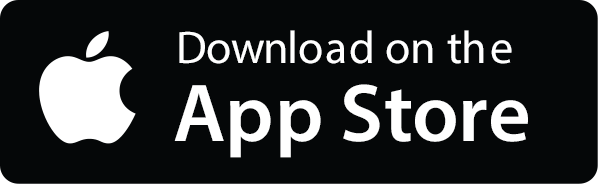
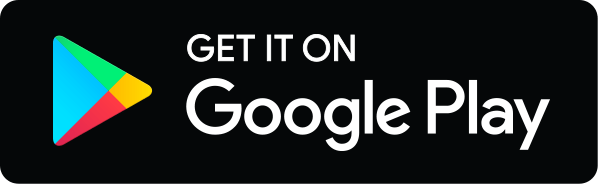