Chapter 66 Heart Rate Variability and Heart Rate Turbulence
Heart Rate Variability
Heart rate variability (HRV) refers to beat-to-beat changes in R-R intervals and is believed to reflect the continuous interplay between sympathetic and vagal tones.1–4 Under normal physiological conditions, a sinus node plays a role of a heart’s pacemaker, possessing its own intrinsic activity and being under the influence of the autonomic nervous system. A variety of internal and external stimuli are known to change the balance between sympathetic and vagal tones. Consequent changes in heart rate may therefore occur in response to mental or physical stresses, cardiac or noncardiac disease conditions, or medications. Autonomic nervous system imbalance, which is generally defined as an increased sympathetic tone and decreased vagal tone, has been proven to be associated with increased risk of cardiac mortality. Therefore, HRV has become an important tool in identifying patients at risk of cardiovascular death.1–7
Heart Rate Variability Measurements
Assessment of HRV is performed by several methods, including time-domain and frequency-domain analyses as well as nonlinear techniques.1–7 The analysis is usually based on long-term (at least 18-hour) Holter electrocardiogram (ECG) recordings. Short-term analyses could be performed on recordings of as short as 5 minutes’ duration, which usually are obtained under controlled, standardized conditions to avoid the influence of external stimuli that may affect the autonomic nervous tone.
Time-Domain Heart Rate Variability Analysis
Time-domain HRV parameters can be derived from direct measurements of N-N intervals or from the differences between N-N intervals.1–35 The simplest, and most commonly used HRV parameter is the standard deviation of all N-N intervals (SDNN) calculated over a long-term period (Figure 66-1). rMSSD and pNN50 (defined in Table 66-1, along with other parameters derived from the time-domain analysis) are considered as measurements predominantly reflecting parasympathetic modulation of the heart.1,2 SDNN, SDANN, and SDNNIX (also defined in Table 66-1) reflect the overall variability as well as the variability with a predominant parasympathetic influence. Among time-domain HRV parameters, no parameter could be considered to represent predominantly sympathetic modulation of the heart. Values of time-domain HRV parameters are frequently decreased in patients with cardiac (post-infarction status, cardiomyopathies) and noncardiac conditions (diabetes) and may indicate increased risk of mortality.1–5
Table 66-1 Time-Domain and Frequency-Domain Heart Rate Variability Parameters
VARIABLE | UNITS | DESCRIPTION |
---|---|---|
TIME-DOMAIN | ||
SDNN | ms | Standard deviation of all N-N intervals |
SDANN | ms | Standard deviation of the averages of N-N intervals in all 5-minute segments of the entire recording |
SDNNIX | ms | Mean of all the 5-minute standard deviations of N-N during the entire recording intervals |
rMSSD | ms | Square root of the mean of the sum of the squares of differences between adjacent N-N intervals |
pNN50 | Percent of difference between adjacent N-N intervals >50 ms | |
FREQUENCY-DOMAIN | ||
Total power | ms2 | Variance of all N-N intervals <0.4 Hz |
ULF | ms2 | Ultra-low frequency <0.003 Hz |
VLF | ms2 | Very low frequency <0.003 to 0.04 Hz |
LF | ms2 | Low frequency power 0.04 to 0.15 Hz |
HF | ms2 | High frequency power 0.15 to 0.4 Hz |
LF/HF | Ratio | Ratio of low-/high-frequency power |
Geometric methods convert a series of R-R intervals into geometric patterns such as sample density distribution of N-N intervals or the Lorenz plot of N-N intervals.5,8 Geometric methods create histograms of various interval frequencies and are believed to be less affected by noise and artifacts during computerized processing of ECG recordings. The HRV triangular index is determined by dividing the total number of N-N intervals by the maximum of the density distribution.8 This index represents overall HRV, and lower values are associated with increased mortality. In the case of Lorenz or Poincaré plots, subjects with preserved HRV are characterized by a fan-shaped plot, whereas those with depressed HRV are characterized by narrow plots.1–3 Heart rate asymmetry is a recently discovered physiological phenomenon that reflects the larger contribution of heart rate decelerations compared with accelerations to short-term heart rate variability. (Figure 66-2).9 An abnormal structure of heart rate asymmetry has been shown to be associated with increased risk of all-cause mortality in patients with previous myocardial infarction (MI).
It is recommended that for time-domain measurement over the long term, at least 18-hour ECG recordings encompassing morning and night hours should be used. It is also emphasized that time-domain measurements of HRV, obtained from periods of different durations, should not be compared because the length of recording significantly influences the overall variability values.1,10,11 The task force on HRV analysis recommends the following measures for time-domain assessment: SDNN, HRV triangular index, SDANN, and rMSSD.1 It is assumed that SDNN and HRV triangular index estimate the overall HRV, SDANN reflects the long-term component of HRV, and rMSSD and pNN50 estimate predominantly parasympathetic modulation.
Frequency-Domain Heart Rate Variability Analysis
Spectral analysis provides information on how the power of HRV is distributed as a function of frequency and is usually performed on the basis of short-term recordings (5 minutes), even though long-term recordings may also be used.1,6 Such analysis aims to separate the different frequency components of an entire R-R interval modulation (Figure 66-3). The most frequently used approach to compute spectral indexes is based on the fast Fourier transformation. The total power of R-R interval variability is the total variance and corresponds to the sum of the four spectral bands: low frequency (LF), high frequency (HF), very low frequency (VLF), and ultra-low frequency (ULF) (see Table 66-1). Spectral analysis obtained from short-term recordings is characterized by three major components: (1) the HF component, (2) the LF component, and (3) the VLF component. Although vagal tone is considered a major contributor to the HF component, LF is believed to reflect both sympathetic and vagal influences. Sympathovagal balance is frequently expressed by an LF/HF ratio. In a healthy subject during resting, controlled conditions, a slight predominance of LF over HF is seen; therefore, the LF/HF ratio is usually between 1 and 2. VLF represents the numerous influences on the heart, including thermoregulation, the renin-angiotensin system, and endothelial factors, but it is also considered a measure of sympathetic activity. The ULF spectral component represents very low oscillations and might reflect circadian and neuroendocrine rhythms. The power of the spectral components may be expressed in absolute (ms2) and normalized units (nu). Normalized units are obtained as follows:
For frequency-domain methods, at least a 1-minute recording is needed to assess the HF component, whereas 2 minutes are required for LF analysis. Nevertheless, for standardization, 5-minute recordings in controlled conditions are recommended.1,10
Despite different techniques of analysis, time and frequency parameters are strongly correlated to each other. Time-domain methods are preferred for long-term recording analysis. Currently, the majority of commercial Holter devices provide an automatic measurement of HRV parameters. It should be considered, however, that independent of the method applied, careful editing of R-R intervals is needed. The task force on HRV recommends that for standardization of physiological and clinical studies, two types of recordings should be used: (1) short-term 5- minute recordings in stable conditions with frequency domain analysis; and (2) 24-hour recordings with time-domain analysis. Spectral analysis could also be performed on the entire 24-hour period, from 5-minute segments yielding LF and HF values and averaged over the entire 24-hour period. The methodology of HRV measurement was standardized in a special report of the Task Force of the European Society of Cardiology/Heart Rhythm Society (ESC/HRS) in 1999 and in the American College of Cardiology/American Heart Association (ACC/AHA) guidelines for ambulatory electrocardiography.1,10
Nonlinear Heart Rate Variability Analysis
The last decade brought an increasing interest in nonlinear methods to analyze heart rate variability. Nonlinear analysis is believed to be less dependent on the pre-processing edition of beats and to express a more complex nature of R-R interval variability. Even though the exact pathophysiological background of nonlinear indices has not been fully clarified, evidence exists that a more complicated mathematical approach may be superior to conventional HRV parameters in risk stratification. Methods that have been proven to provide prognostic information are detrended fluctuation analysis, power law relationship analysis, and approximate entropy.7,11–13
Clinical Covariates of Heart Rate Variability
Numerous conditions affect sympathovagal status and influence heart rate and its variability. Age has been reported as a major determinant of HRV with constant decline in time-domain and frequency-domain parameters over the course of a person’s life.14 Approximately, a 15% decline in LF and HF power was reported for every 10 years, with an early fall in the HF component.15 Women tend to have lower SDNN and LF but higher HF values compared with men. Nevertheless, these differences weaken with age and gender; the diminishing of differences around menopausal age suggests a potential hormonal influence on the autonomic nervous system.16
Decrease in HRV has consistently been observed in patients after MI and is reported to contribute to both structural changes of the left ventricle and to a decrease in vagal activity or to blunted response of a sinus node to autonomic regulation.17 Consequently, it has been postulated that such a shift toward increased sympathetic activity and loss of vagal protection may contribute to enhanced arrhythmogenesis and subsequent sudden cardiac death (SCD) caused by lethal arrhythmias. Patients with previous MI are therefore characterized by a significant reduction in SDNN, which is correlated with the degree of left ventricular dysfunction and parallel decrease in spectral components.18,19 Decreased HRV parameters have been observed not only in patients after MI but also in nonischemic cardiomyopathy and in a steadily growing population of patients with heart failure, including those with preserved left ventricular function. The extent of HRV reduction correlates with the advancement of heart failure expressed by measurements of ejection fraction (EF), New York Heart Association (NYHA) class, or B-type natriuretic peptide (BNP) levels.20–23
HRV is known to be modified by a variety of drugs, reperfusion strategies, regular exercise training, and cardiac resynchronization. The initial impact of thrombolysis on HRV in patients with acute MI was confirmed more recently by studies involving patients undergoing invasive reperfusion procedures with percutaneous coronary angioplasty.24 Recent years have brought additional information that in patients with heart failure changes in HRV after cardiac resynchronization therapy (CRT), implantation may identify CRT responders. Low HRV values before implantation and no increase during postprocedural follow-up identify patients at risk of progression to heart failure.25,26
Clinical Applications of Heart Rate Variability
The association between abnormal HRV, impaired autonomic nervous tone, and cardiovascular mortality is well documented (Table 66-2). Even though experimental data indicated an association between impaired HRV and the preponderance toward ventricular arrhythmias, data on the association between decreased HRV and SCD are conflicting. Most post-infarction and heart failure studies indicate that depressed HRV mainly identifies patients at risk of overall mortality, with less evidence for the association with arrhythmic death. Evidence linking depressed HRV with implantable cardioverter-defibrillator (ICD)–documented ventricular tachycardia (VT) or ventricular fibrillation (VF) is limited.1–7
Patients with Previous Myocardial Infarction
The clinical relevance of HRV as a prognostic tool was first appreciated in 1965 by Hon and Lee.27 Nevertheless, its history in cardiac risk stratification came about in the late 1980s when the first publications on HRV as a risk stratifier in patients with previous MI were published.28,29 In 1987, Kleiger et al, of the Multicenter Post-Infarction Project (MPIP), reported than an SDNN of less than 50 ms was associated with more than a fivefold higher risk of mortality during a 31-month follow-up of more than 800 survivors of MI, compared with those with SDNN greater than 100 ms.29 Low HRV remained a significant predictor of mortality after adjustment for other significant stratifiers such as EF, Killip Kimball class, and ventricular premature beats (VPBs). However, a combination of reduced SDNN with any of a group of risk markers (≥10 VPBs per hour, left ventricular ejection fraction [LVEF] <30%, failure to perform exercise test, or heart rate >80 beats/min) identified patients with a mortality rate of 50% during a follow-up.
Multiple publications from the 1990s consistently confirmed the predictive value of HRV parameters in patients with previous MI.30–32 The Grupo Italiano per 10 Studio della Sopravvivenza nell’Infarto miocardico (GISSI) study in 567 males treated with thrombolysis revealed that SDNN, pNN50, and rMSSD were independently associated with all-cause mortality during a 3-year follow-up and thus confirmed the predictive value of HRV in the thrombolytic era.30 The Autonomic Tone and Reflexes After Myocardial Infarction (ATRAMI) study enrolled low-risk patients with previous MI and demonstrated that SDNN less than 70 ms was associated with a threefold higher mortality rate (relative risk [RR], 3.2).31 Both SDNN less than 70 ms as well as low baroreflex sensitivity (BRS; <3 ms/RR) were significantly associated with an adverse outcome, and a combination of having both decreased SDNN and decreased BRS identified patients with a 17% mortality rate compared with 2% in patients with both parameters within higher value.31 Predictive accuracy of SDNN was further significantly improved when analyzed together with low EF. In the placebo arm of the Azimilide Post-Infarct Survival Evaluation (ALIVE) trial of patients with previous MI and depressed LVEF, low HRV triangular index (<20 U) was found to identify patients at higher risk for death at 1 year compared with those with HRV index greater than 20 U (15 vs. 9.5%, P < .005).32 Nevertheless, no significant relationship with arrhythmic events was observed. The majority of studies focused on time-domain parameters assessed from 24-hour Holter recordings; however, other reports have provided information on the prognostic role of spectral HRV measurements.33–35 Bigger et al found that 5-minute time-domain and frequency-domain parameters calculated on the basis of randomly chosen 5-minute periods, provided significant, although weaker, prognostic information compared with the same parameters calculated over a 24-hour period in patients from MPIP.33
Acute coronary syndromes are currently managed with the wide use of primary angioplasty and β-blockers. Makikallio et al addressed the prognostic role of Holter-derived risk parameters in the stratification of patients with previous MI treated according to current guidelines.36 On the basis of a study of 2130 patients, of whom 70% underwent invasive coronary revascularization and 94% were treated with a β-blocker, the authors documented that all HRV parameters except HF (SDNN <70 ms, lnVLF <5.3, lnLF <3.85, and degree of frequency analysis [DFA, α1]) were significant univariate predictors of SCD and non-SCD deaths, but only LF and α1 predicted non-SCD mortality when adjusted for significant clinical covariates. Only reduced α1 was able to independently identify patients at risk of SCD during a median of 1012 days of follow-up (hazard ratio [HR], 1.9; 95% confidence interval [CI], 1.0 to 3.6; P = .0444). Of note, HRV parameters (α1 and VLF) were able to predict SCD among patients with previous MI who had an LVEF greater than 35%. Nevertheless, it should be stressed that in concordance with recent observations on lower incidence of SCD in patients with previous MI undergoing aggressive reperfusion, only 52 SCDs occurred in a studied population (2.5%), with the majority in patients with LVEF greater than 35%. The Risk Estimation Following Infarction Non-invasive Evaluation (REFINE) study failed to demonstrate the usefulness of decreased SDNN in predicting cardiac death or resuscitated cardiac arrest in 322 patients with an acute infarction and LVEF less than 50%.37
The recently published Clopidogrel for High Atherothrombotic Risk and Ischemic Stabilization, Management, and Avoidance (CARISMA) study is the first study to evaluate a variety of ECG markers in predicting arrhythmic events (VF or VT) documented by the implantable loop recorder.38 In patients evaluated 6 weeks after an acute MI, SDNN, VLF, LF, and the fractal scaling component analyzed as continuous variables were independently associated with all-cause mortality as well as primary arrhythmic endpoint documented by the implantable loop recorder. It is worth emphasizing that only α1 was associated with arrhythmic events when risk stratifiers were evaluated in an early post-infarction period (1 week). When categorized values were used, a decreased VLF component (VLF ≤5.7) had the highest HR (7.0, P < .001), followed by HF less than 3.5 (HR, 6.2; P = .023), SDNN less than 70 ms (HR, 4.6; P = .006), and α1 less than 0.75 (HR, 3.5; P = .019) (Figure 66-4).
Patients with Heart Failure
Decreased HRV has been considered for years to be an independent and strong marker of risk for all-cause mortality or death caused by heart failure, whereas data on predicting SCD in this population are limited.38–44 Early reports on the predictive value of HRV showed that reduced HRV parameters were related to a 20-fold increased risk of death in patients awaiting heart transplantation.39 In the UK-Heart Study SDNN, less than 50 ms was associated with death from progressive heart failure but failed to predict SCD.40 SDNN is the most extensively studied HRV risk stratifier, but no consensus on the cut-off has been achieved so far. The other studies indicated SDNN less than 100 ms, 67 ms, or 65.3 ms as predictors of death in patients with heart failure.41,42 Despite different cut-offs, a constant trend is seen in all the published studies toward the high prognostic value of depressed HRV in predicting death from heart failure and all-cause mortality.
More controversies exist in terms of frequency-domain components. The findings of these studies are difficult to compare, mainly because of different methodologic approaches. Decreased LF and VLF components are the most frequently reported HRV spectral measures related with poor outcome in patients with heart failure.43–47 La Rovere et al reported that low-frequency power measured from short-term recordings during controlled breathing was a powerful predictor of SCD in 202 patients with moderate to severe congestive heart failure.43 It should also be emphasized that different components of spectral analysis were documented to be related to different types of death. In a group of 330 patients with congestive heart failure in NYHA class I to III, decreased nighttime VLF was related to progressive heart failure, whereas decreased nighttime LF values were associated with SCD.47 Nonlinear HRV measures were also reported to provide prognostic information on mortality in patients with CHF.11–13 Maestri et al, who aimed to compare several nonlinear HRV methods in predicting mortality in patients with congestive heart failure, demonstrated that despite differences in prognostic values, assessment of nonlinear indexes provides important prognostic information in addition to clinical data.13
The prognostic role of HRV in the prediction of SCD in patients with congestive heart failr remains controversial. Most studies support the prognostic value of HRV in predicting the progression of heart failure. Only a study of Fauchier et al showed that reduced SDNN (less than 100 ms) was an independent risk predictor of SCD and arrhythmic events in patients with dilated cardiomyopathy.44 Rashba et al in the Defibrillators in Non-ischemic Cardiomyopathy Treatment Evaluation (DEFINITE) trial demonstrated that SDNN greater than 113 ms identified patients with 0% mortality during a 3-year follow-up.48 Preserved SDNN also identified patients with 0% SCD and ICD shocks rates (Figure 66-5). It is therefore plausible that preserved function of the autonomic nervous system may serve to identify low-risk patients with previous MI. It cannot be excluded that the paucity of clear evidence for the association between depressed HRV parameters and SCD might be associated with difficulty in categorizing the sudden or arrhythmic nature of death. The autonomic nervous system operates differently in various patients, depending on the disease as well as the advancement of the disease process. HRV parameters successfully predict worsening of the congestive heart failure and total mortality in patients with congestive heart failure, indicating that autonomic dysfunction is part of the overall clinical picture in such patients, but these parameters seem to have little or no prognostic significance for predicting arrhythmic events in these patients.
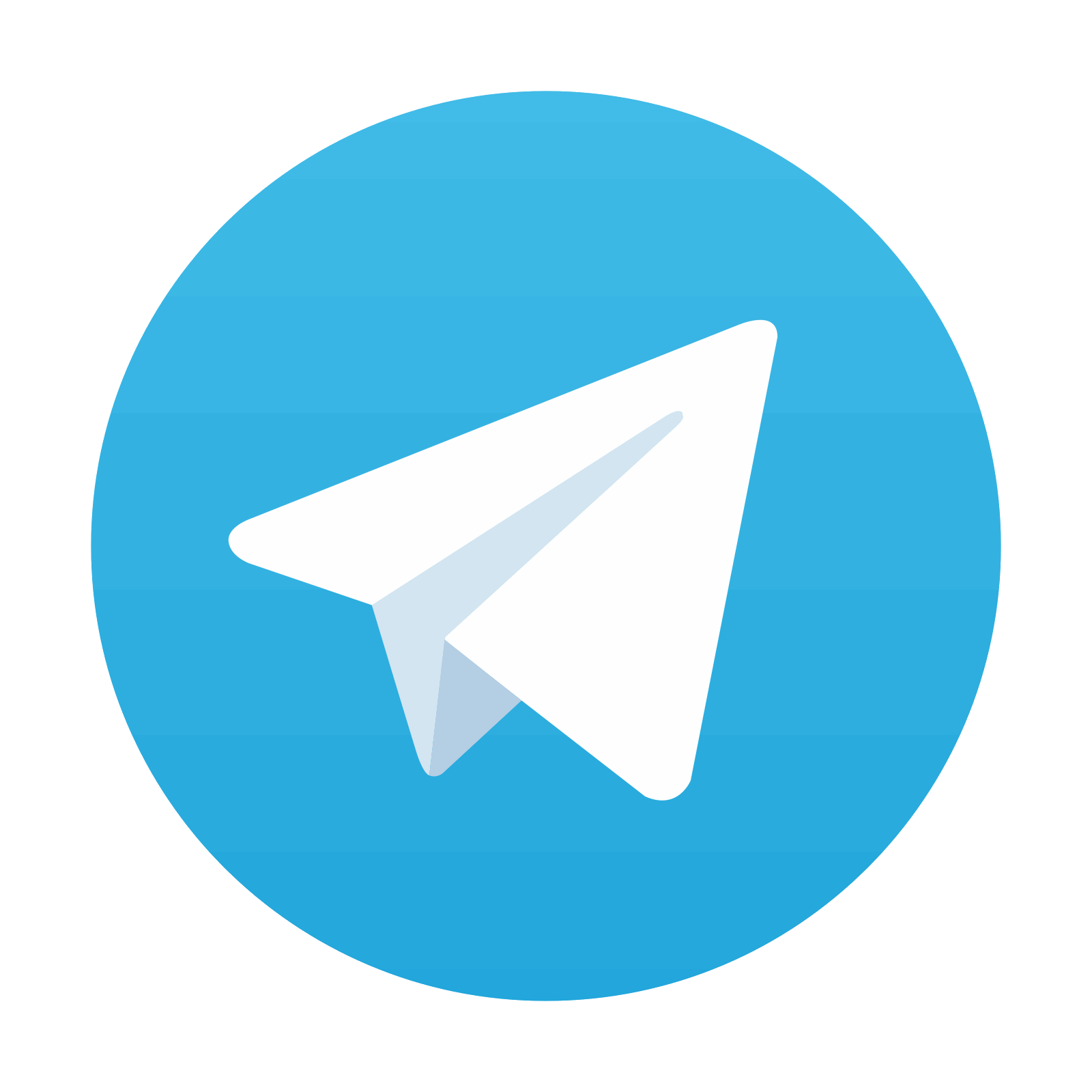
Stay updated, free articles. Join our Telegram channel

Full access? Get Clinical Tree
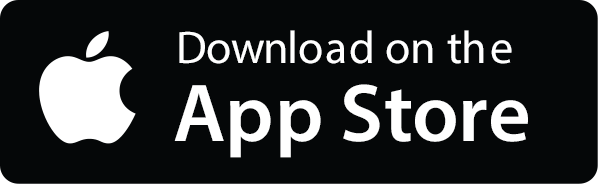
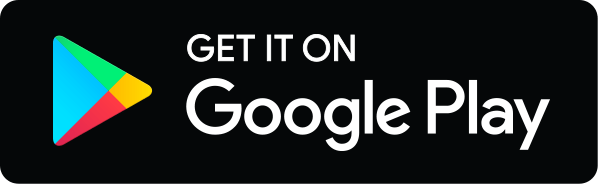