CHAPTER 100 Heart–Lung Transplantation
The clinical realization of heart–lung transplantation evolved through much earlier experimental developments in the laboratory. Alexis Carrel1 (Fig. 100-1), and later Demikhov2 and Marcus and associates,3 performed experiments that explored the possibility of heart–lung transplantation to replace diseased organs. When heart transplantation began in 1968, Cooley, Lillehei, and Barnard all performed human heart–lung transplants, with short survival. Progress in the laboratory translated to the clinical application of the first successful human heart–lung transplantation performed at Stanford in 1981.4 Since these initial clinical transplantations, the field of heart–lung transplantation and lung transplantation alone has undergone dramatic changes in all aspects, including indications, operative technique, and postoperative management. This chapter reviews the state of the art and current issues in the field of heart–lung transplantation.
INDICATIONS FOR HEART–LUNG TRANSPLANTATION
Recipient Diagnosis
The diagnostic profile of heart–lung transplant recipients reported to the Registry of the International Society for Heart and Lung Transplantation (ISHLT) through 2007 is depicted in Figure 100-2.5 Pulmonary hypertension secondary to congenital heart disease is the most frequent diagnosis, found in 33.9% of individuals who require heart–lung transplantation.5 Cardiac lesions that can result in secondary pulmonary hypertension include atrial and ventricular septal defects, patent ductus arteriosus, truncus arteriosus, and other complex congenital anomalies, including univentricular heart with pulmonary atresia and hypoplastic left heart syndrome. Pulmonary hypertension secondary to congenital heart disease varies prognostically when compared with primary pulmonary hypertension (PPH). Despite comparable levels of pulmonary arterial pressures, individuals with Eisenmenger’s syndrome have lower right atrial pressures, better cardiac index, and an overall better prognosis than patients with pulmonary hypertension without congenital heart disease.6 Therefore, hemodynamic variables are less reliable in this group than the presence of progressive symptoms as an indicator for transplantation (see later). Patients with significant hemodynamic derangements may be effectively managed with medical therapy, thus delaying transplantation.
Secondary pulmonary hypertension with complex, irreparable cardiac defects must be treated with heart–lung transplantation. In a recent study, patients with Eisenmenger’s syndrome caused by a ventricular septal defect (VSD) or multiple congenital anomalies had a highly significant survival advantage when a heart–lung transplantation was performed as opposed to cardiac repair combined with lung transplantation.7 However, patients with simple cardiac defects, such as atrial septal defects, should be considered for repair of the cardiac defect combined with single or bilateral lung transplantation. Lung transplantation with intracardiac repair has evolved as a viable alternative to heart–lung transplantation for several reasons. First, right ventricular function improves after lung transplantation as a result of normalization of pulmonary vascular resistance.8 Also, there is an organ donor shortage, and furthermore the denervation and graft coronary artery disease associated with cardiac transplantation is avoided.
PPH associated with irreversible right ventricular failure is the second most common indication for heart–lung transplantation, with 24% of recipients carrying this diagnosis.5 In contrast to secondary pulmonary hypertension from congenital heart disease, abnormal hemodynamic parameters are closely related to increased mortality.9 Therefore, patients with PPH should be promptly referred to a transplant pulmonologist for initiation of intravenous pulmonary vasodilators. If severe right ventricular failure persists despite aggressive medical therapy, heart–lung transplantation may be indicated.
The role of heart–lung transplantation in the setting of PPH has also evolved over the past several years. Individuals with pulmonary vascular disease and improved right ventricular function after initiation of medical therapy may also be treated effectively with either single-lung transplantation (SLT) or double-lung transplantation (DLT). In the setting of PPH, DLT results in both greater hemodynamic improvement and a shorter duration of postoperative mechanical ventilation than SLT.10 It is the preferred operation in most transplantation centers. Furthermore, comparable survivals were noted after heart–lung transplantation and DLT in patients with PPH.11 This has led to the recent trend in favor of DLT for patients with PPH because it decreases the use of scarce donor hearts. This trend has resulted in a decrease in the number of heart–lung transplants for PPH over the past 10 years. The subsets of patients who have fixed pulmonary vascular resistance not responsive to vasodilators and who have irreversible right-sided heart dysfunction are still candidates for heart–lung transplantation.
The introduction of the “domino” transplantation procedure renewed interest in heart–lung transplantation for septic lung disease. In this procedure, the recipient of a heart–lung transplant served as a heart donor for a second recipient, thus maintaining donor organ allocation.12,13 Recent studies have demonstrated equivalent survival rates in recipients of domino heart grafts and in recipients of cadaveric heart grafts. However, this procedure is rarely performed, and bilateral lung transplantation remains the procedure of choice for most septic or parenchymal lung disease.
The distribution of preoperative diagnoses managed with heart–lung transplantation at Stanford from 1981 to the present has been as follows: Eisenmenger’s syndrome, 44%; PPH, 23%; cystic fibrosis, 12%; complex congenital heart disease, 12%; α1-antitrypsin deficiency, 2%; cardiomyopathy with pulmonary hypertension, 2%; failure of SLT, 1%; congenital bronchiectasis, <1%; pulmonary lymphangioleiomyomatosis, <1%; and other diagnoses, 3%.14
Recipient Selection Criteria
Recipients of heart–lung transplants generally are younger than heart transplant recipients, with 44% being between the ages of 35 and 49 years, and only 15% being older than 60.5 It must be determined that the patient and the family are emotionally prepared for transplantation. Each patient must be assessed for the presence of adequate emotional and psychosocial support to withstand the rigorous and intrusive medical regimen that will continue throughout life. Heart–lung transplantation has become more common as a therapeutic option for complex congenital heart disease in infants and children.15,16 These recipients also must be carefully evaluated, and they should have an extremely strong family support network to cope with the complex regimen and the frequent medical follow-ups.
The risk for mortality on the waitlist must be balanced with the 1-year mortality risk after transplantation to determine the ideal time to activate someone on the transplant waitlist. The individual’s disease process should be severe enough to warrant heart–lung transplantation, yet the patient must be well enough to survive the long wait until the transplantation occurs, and well enough to survive the surgical procedure itself.17 The underlying disease determines the appropriate window. Survival with or without transplantation varies by disease process and by the patient’s overall physical condition. Identification of a critical turning point in a patient’s disease that signals an accelerated decline is the key to appropriate referral for transplantation. Pulmonary and cardiac clinical signs that can be used to gauge the appropriateness of listing include changes in the rate of decline in exercise capacity or pulmonary function, increasing frequency of infectious complications, increasing number and duration of hospitalizations, and increasing supplemental oxygen requirements. Other signs include increasing requirements for diuretics and increased hepatomegaly and bilirubin levels. Hyperbilirubinemia has been shown to be a marker for poor postoperative outcome in patients with pulmonary hypertension and right ventricular dysfunction. Patients with chronic bilirubin levels greater than 2.1 mg/dL who are undergoing heart–lung transplantation have been demonstrated to have significantly worse postoperative mortality (58%).18 The rate of perioperative complications, including coagulopathy, hepatic encephalopathy, poor wound healing, and impaired clearance of cyclosporine with resultant nephrotoxicity, were also significantly increased after heart–lung transplantation in such patients.19
Heart–lung transplantation should be undertaken before multisystem organ failure and severe malnutrition ensue. Many patients, while they await a suitable donor, become increasingly debilitated. Every opportunity to optimize their physical strength and nutritional status should be aggressively pursued.20 Good physical performance before transplantation has been associated with improved postoperative recovery. Therefore, patients should be referred for physical therapy early in an effort to improve their functional status and optimize their recovery potential.
In the past, most centers considered conditions associated with extensive adhesions, such as previous thoracotomy or sternotomy, septic lung disease, and previous chemical or surgical pleurodesis, an absolute contraindication to heart–lung transplantation. Historically, these patients had an increased morbidity and short-term mortality, resulting primarily from excessive blood loss and pulmonary issues associated with massive transfusions of blood products. The introduction of antifibrinolytic therapy (aprotinin) in thoracic organ transplantation has provided improved outcomes for this group of patients.21 However, with increasing evidence that this drug is associated with worse survival and a higher risk for renal and neurologic complications after isolated coronary artery bypass surgery, its use in North American surgical programs has diminished markedly. Heart–lung transplantation in patients with previous surgery may remain one indication for its continued use.22 We now routinely accept patients with previous cardiac or thoracic surgery for heart–lung transplantation, and they represent a significant proportion of the current waiting list.
In patients selected for transplantation, routine pretransplantation screening is performed for ABO blood group, the presence of preformed antibodies, human leukocyte antigen (HLA) and reaction of degeneration (DR) tissue types, and titers of antibodies against cytomegalovirus, herpes simplex virus, and toxoplasmosis. ABO compatibilities are strictly adhered to because isolated episodes of hyperacute rejection have been observed in transplantations performed across ABO barriers. In addition to ABO compatibility, donor–recipient matching also is based on body size. In practice, matching donor and recipient height seems to be the most reproducible method for selecting the appropriate donor lung size. In general, the dimensions of the donor lungs should not be greater than 4 cm greater than those in the recipient. Other investigators have proposed that the simplest method of matching donor to recipient lung size is to use their respective predicted total lung capacity (TLC) values.23,24
Once an appropriate donor–recipient match is made, the recipient is screened for preformed antibodies against a panel of 50 random donors. A percent reactive antibody (PRA) level greater than 25% prompts a prospective specific crossmatch between the donor and recipient. A positive crossmatch indicates the presence of antidonor circulating antibodies in the recipient, and the organ cannot be accepted for transplantation. Despite several retrospective studies that reveal improved graft survival with HLA matching,25–27 prospective HLA matching is not feasible given the short ischemic times tolerated. Furthermore, in a recent multicenter study, HLA matching for lung transplantation did not have a large effect on clinical outcome, including the development of obliterative bronchiolitis.28
Recent data suggest that the presence of an elevated PRA is associated with both early and late mortality.29 Additionally, elevated PRA levels have been shown to be associated with an increased incidence of acute rejection in both lung and heart transplantation patients, with an increased incidence of graft vascular disease in heart transplantation patients, and with an increased occurrence of accelerated bronchiolitis in lung transplant recipients.30,31 Methods to decrease the patient’s PRA and to avoid antibody production by virtual, or, when time allows, prospective cross-matching, have decreased the negative effects of high baseline PRA on late survival.32
Many heart–lung transplant patients, especially in the pediatric population, have undergone previous cardiac surgery and are extremely sensitized when they present for heart–lung transplantation. Specific protocols using immunoglobulin, plasmapheresis, and rituximab have been shown to decrease the PRA in potential organ recipients. Additionally, post-transplant treatment initiated in the operating room with plasma exchange, plasmapheresis, weekly immunoglobulin injections, and monitoring for the development of donor-specific antibodies has minimized the risk for hyperacute rejection in this population.33 The current desensitization protocol being used at Stanford is shown in Box 100-1.
TREATMENT OF PATIENTS AWAITING TRANSPLANTATION
The average waiting period for a heart–lung block is greater than 18 months, so treatment of recipients after listing is a critical aspect of the preoperative plan. Routine medical care, including clinical surveillance, optimization of cardiopulmonary function, and management of disease-specific and age-specific issues, should be addressed during this time. General considerations in the pretransplantation period include appropriate surveillance protocols and screening for malignancies such as prostate, breast, and colon cancer. Other issues that should be addressed include appropriate prophylaxis for thromboembolism with warfarin,34 and aggressive maximization of bone density, because osteoporosis is increasingly recognized as a clinical problem for patients on chronic steroids.35 Nutrition and high-intensity pulmonary rehabilitation should be optimized. Finally, psychosocial issues need to be addressed, with referral for supportive counseling, support groups, and other training means to help each patient cope with the upcoming challenges.
Disease-specific issues in patients awaiting heart–lung transplantation need to be addressed. These patients require optimal treatment of heart failure with standard therapeutic measures such as sodium restriction, aggressive diuresis, and vasodilators. Afterload reduction with nitrates, hydralazine, and angiotensin-converting enzyme inhibitors prolong survival while awaiting transplantation.36 In patients with PPH, supplemental oxygen is recommended to avoid hypoxic pulmonary vasoconstriction. Indications for outpatient oxygen therapy include an arterial partial pressure of oxygen (PaO2) of 55 mm Hg or less (or arterial oxygen saturation [SaO2] less than 88% on room air) at rest, during exertion, or while asleep.37 The prognosis of patients with PPH is determined by hemodynamic variables, and the following parameters are associated with a poor outcome: increased mean pulmonary artery pressure (mPAP > 85 mm Hg; median survival, 12 months), increased mean right atrial pressure (RAP > 20 mm Hg; median survival, 1 month), and decreased cardiac index (CI < 2.0 L/min/m2; median survival, 17 months).9 In addition to supplemental oxygen therapy, initiation of pulmonary vasodilator therapy improves the hemodynamics and survival while awaiting transplantation. Epoprostenol is a short-acting prostaglandin, released normally by the endothelium, that vasodilates the pulmonary vascular bed and inhibits platelet aggregation. Continuous infusion of epoprostenol (Flolan) has been demonstrated to significantly improve pulmonary hemodynamics, exercise capacity, and survival in patients with NYHA III and IV heart failure.38,39 Patients may then wait longer for transplantation. Despite improvements in pulmonary hemodynamics and survival, diseases often progress, or the patients develop tachyphylaxis. Therefore, patients need to be followed closely and returned to the active list if any clinical deterioration is noted. In patients with right ventricular failure awaiting transplantation, creation of an interatrial shunt to decompress the pressure-overloaded ventricle may need to be considered.40
Patients with cystic fibrosis awaiting heart–lung transplantation have special needs. Pulmonary hygiene is of critical importance, as more than 80% of patients are chronically colonized with Pseudomonas aeruginosa.41 Pulmonary clearance techniques, institution of bronchodilators, and initiation of antibiotics in the setting of acute infection are lifelong requirements. Prophylactic antibiotics are not routinely given because of the development of multiresistant organisms.42 Sinus disease and lower respiratory tract infections are risks in most patients with cystic fibrosis. Bilateral maxillary sinus antrostomies performed in the pretransplantation period have resulted in fewer infectious complications in the post-transplant period.43 In addition to infectious issues, other associated risk factors such as malnutrition secondary to malabsorption, pancreatic insufficiency, and diabetes mellitus must be properly addressed.
SELECTION AND MANAGEMENT OF DONORS
The satisfactory criteria for heart–lung blocks are similar to those for hearts and lungs separately (Box 100-2). The lungs are the most delicate solid organ, with frequent parenchymal damage and neurogenic edema occurring after brain death, as well as the increased risk for aspiration. These factors result in only 20% to 30% of multiorgan donors having lungs suitable for donation. Preliminary donor evaluation includes a detailed history, review of electrocardiogram and echocardiogram, chest film, and arterial blood gas analysis. A donor age of less than 40 is preferred. However, potential donors aged 40 to 50 years are considered at most transplant centers, provided they have normal cardiopulmonary functioning and a normal coronary arteriogram. Acceptance criteria also include no significant lung contusion or history of aspiration or sepsis. There should be no history of prior cardiac or pulmonary surgery. A donor chest film must be clear with no signs of pathology. An arterial blood gas analysis should reveal an arterial oxygen tension level of greater than 350 mm Hg on a fractional inspired oxygen concentration (FiO2) of 100%, and 100 mm Hg on an FiO2 of 30%. Lung compliance is estimated by measuring peak inspiratory pressures, which should not exceed 30 cm H2O. Tracheobronchial infection must be excluded with chest radiography, Gram stain of sputum, and bronchoscopy.
Box 100–2 Heart–Lung Donor Selection Criteria
Because of growing waiting lists and increased mortality before a transplant is received, most centers have expanded their donor acceptance criteria with increased use of so-called marginal donors.44 Thoracic trauma with a resultant pneumothorax is not a contraindication, provided there is not a continuous air leak. Furthermore, lungs that are mildly contused also can be used, provided ongoing bleeding is not noted on bronchoscopy and/or there is no major atelectasis. Patients with prolonged intubation and ventilation (>70 hours), once thought to be a contraindication, also should be considered. As noted, older donors also are being considered, provided pulmonary functional parameters are normal and there is no significant coronary artery disease. Infection with hepatitis C virus is an evolving area in cardiopulmonary transplantation. Hepatitis C–negative recipients have an increased risk for acquiring hepatitis, but the effect on patient survival remains unclear.45 Absolute contraindications include severe coronary or structural heart disease, prolonged cardiac arrest, active malignancy (sometimes excluding primary brain cancers such as astrocytoma and skin cancers), positive smoking history of greater than five pack-years, and positive human immunodeficiency virus (HIV) status.
Donor management after a declaration of brain death is a critical area, with an opportunity for significantly improving the number and quality of potential donors. Aggressive management strategies implemented by organ procurement organizations make “unacceptable” lungs, defined as a PaO2-to-FiO2 ratio of less than 150, acceptable for transplant.46 These unacceptable donors were treated with invasive monitoring, methylprednisolone, fluid restriction, inotropic agents, bronchoscopy, and diuresis. The resultant “acceptable” organs were successfully transplanted without compromise of either 30-day or 1-year graft survival.46 The major goal in the initial management of these donors is the maintenance of hemodynamic stability and pulmonary function. Patients who have suffered an acute brain injury are usually hypovolemic, and appropriate fluid resuscitation is the initial step. Complicating factors include the development of diabetes insipidus, which should be treated with a vasopressin infusion (0.1 to 0.4 U/hr).47 Fluid administration is guided by the central venous pressure (CVP), keeping in mind that a CVP of greater than 8 mm Hg has been demonstrated to be an independent risk factor for the development of lung dysfunction.48 Inotropic agents such as dopamine or phenylephrine are instituted to maintain perfusion pressure after intravascular volume has been repleted. The donor is not hyperventilated after brain death is declared, and a pH of 7.40 is the goal, thus avoiding pulmonary vasoconstriction. Positive end-expiratory pressure of 5 is maintained to prevent atelectasis, and higher levels are avoided because of their deleterious effect on cardiac output.
The pathophysiology of brain death and its deleterious effect on the cardiovascular system have been well described.49 The resultant endocrine and metabolic derangements are a potential area of intervention in the marginal donor with hemodynamic instability. Hormonal therapy, including triiodothyronine (T3), cortisol, insulin, and vasopressin, has been demonstrated to have beneficial effects on the hemodynamic profile of marginal donors.50 Physiologic resuscitation, guided by a better understanding of the pathophysiology of brain death, ultimately leads to increased number and quality of heart–lung donors.
HEART–LUNG PRESERVATION
Minimization of IR injury ultimately results in optimization of allograft function in the post-transplantation period. Nonspecific graft failure, which most likely represents IR injury, remains a significant cause of perioperative morbidity and possibly mortality.51 Reperfusion injury may predispose to an increased risk for acute rejection secondary to the upregulation of histocompatibility class II antigens.52 Understanding the pathophysiology of IR injury allows the implementation of strategies aimed at preserving function, minimizing injury, and prolonging allograft and patient survival.
The result of inadequate preservation is activation of the inflammatory cascade, resulting in pulmonary endothelial injury and increased capillary permeability. The resulting pulmonary interstitial and alveolar edema leads to diminished airway compliance, poor gas exchange, and increased pulmonary vascular resistance. Numerous recent studies have elucidated the role of the neutrophil and its interaction with the pulmonary endothelium in the pathogenesis of IR injury.53–55 Reperfusion injury is caused in part by a complex interplay between leukocyte activation and subsequent release of inflammatory mediators and cytokines, with a final common pathway of pulmonary endothelial injury.
Since the early 1980s, various methods were developed to provide adequate preservation. These have varied from simple graft excision and cold storage to sophisticated autoperfusion with donor blood and a working heart–lung preparation. Today, only two methods are actively employed: (1) perfusion with pulmonoplegia solutions and cold storage and (2) perfusion with cold donor blood, with both methods using pulmonary vasodilators. Pulmonary artery flush, the most widely adopted method of allograft preservation,56 allows rapid cooling of both lungs, with a significant improvement in allograft function noted after both the infusion note (4 minutes) and total volume (60 mL/kg) were increased.57 To counteract the reflex pulmonary vasoconstriction arising from preservation solutions, donors are pretreated with prostaglandins. These selective pulmonary vasodilators, alprostadil in North America and epoprostenol in Europe, improve the distribution of the pulmonary arterial flush and thus improve lung preservation. The role of retrograde flush through the left atrium has been advanced recently, because of the improved distribution of the flush in a porcine model.58,59
The two types of crystalloid pulmonary artery flush solutions, intracellular and extracellular, are both designed to counteract the cellular swelling that results from graft ischemia and hypothermia. The intracellular solutions, Euro-Collins (EC) and University of Wisconsin, are composed of electrolytes that mimic the intracellular environment. The extracellular solutions include the Wallwork solution, which uses donor blood, and low-potassium dextran (Perfadex). The most commonly used preservation solution is EC crystalloid solution, which has been modified with magnesium sulfate and 50% dextrose. A recent study has demonstrated that lung procurement with low-potassium dextran resulted in a significantly decreased incidence and severity of reperfusion injury with improved allograft function, as well as improved survival, when compared with EC.60 The addition of a retrograde flush for isolated lung transplantation has resulted in improved post-transplant lung function.61 This retrograde flush technique can be safely used in combined heart–lung transplantation by making a small left atrial incision, flushing the preservation solution retrograde via the veins, and collecting the effluent in the proximal pulmonary artery, where a separate small incision is made. In contrast, Celsior solution, when used as a lung-preservation solution, resulted in lethal post-transplant outcomes in a porcine model of lung transplantation.62
Recent investigations in the laboratory and clinic have demonstrated improvements in allograft preservation by modification of the pulmonary arterial flush solutions. The focus has been to intervene at the level of the leukocyte–endothelial interaction in an effort to preserve the function of the pulmonary endothelial cell. The impact of toxic inflammatory mediators elaborated by the leukocyte has been reduced by techniques such as leukocyte filtration,63 introduction of monoclonal antibodies directed against anti-intercellular adhesion molecules and selectins,53,55,64 and vascular immunotargeting strategies.54 Furthermore, treatments aimed at preserving pulmonary endothelial function by restoring endothelium-derived mediators such as nitric oxide and prostacyclin have been demonstrated to improve allograft pulmonary function.65–68 Instead of focusing on the preservation solutions or additives, others have focused their attention of the development of continuous organ perfusion systems. The TransMedics Organ Care System is an organ perfusion system that preserves organs by using a warm blood perfusion of the organ during storage. The system has been approved for use in Europe for donor heart procurement, and in late-stage clinical trial protocol use in the United States.
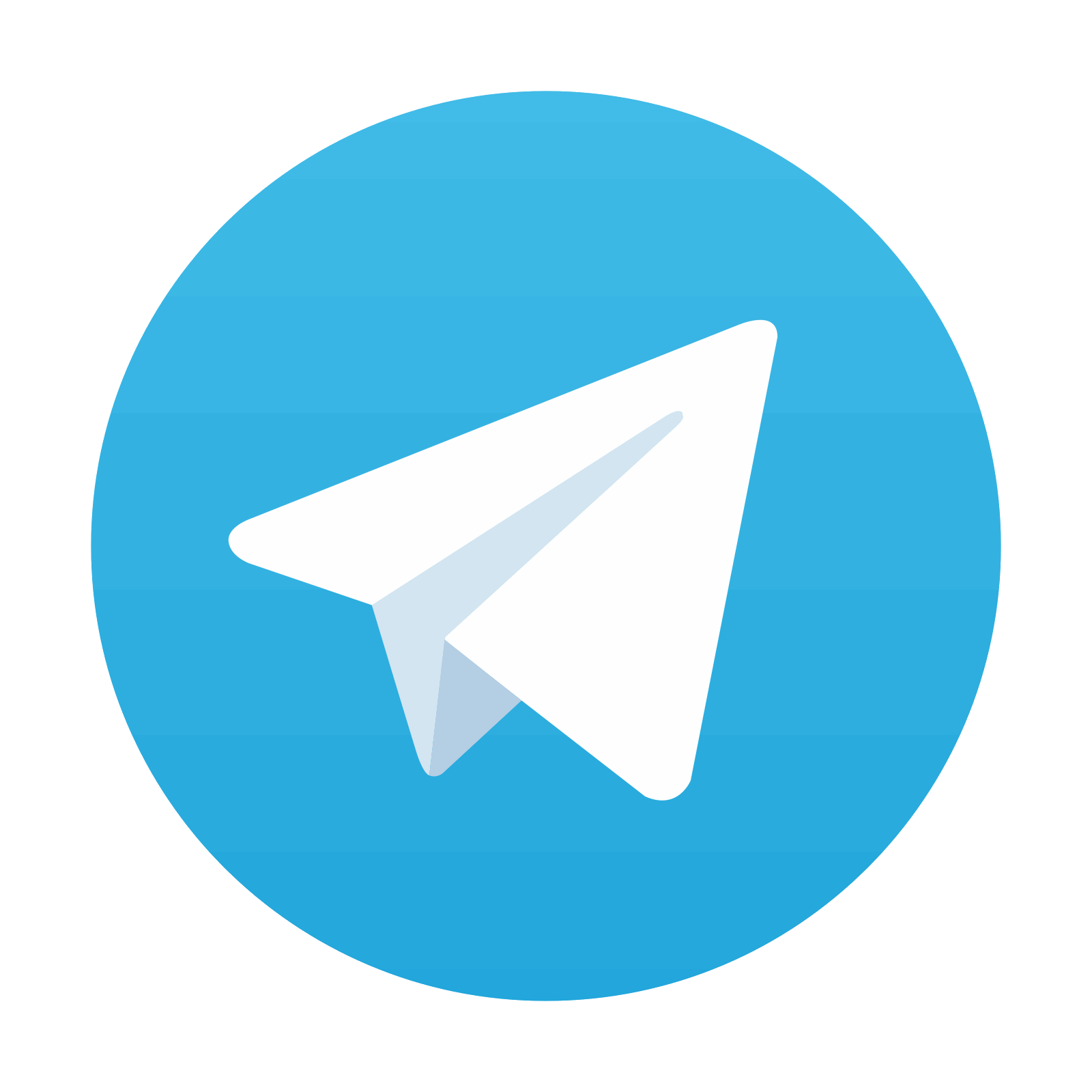