ECMO nomenclature is controversial, as there are authors who disagree with a definition that seems comprehensive, but not specific. As such, the term extracorporeal life support (ECLS) has been proposed and used, however in practice and in the literature these terms are used interchangeably. There are proponents of further taxonomic distinctions based on the indications for device use, such that care for respiratory- and cardiac-failure patients should be performed by different specialists, and in different intensive care units rather than aggregated due to technical convenience.
It is important to understand that despite technical advances in the field, the ability to make decisions for such complex cases requires a thorough understanding of how the device functions (and dysfunctions!), as well as communication with the various stakeholders involved. This can vary among institutions, but often includes intensivists, surgeons, cardiologists, pulmonologists, perfusionists, respiratory therapists, and nurses.
The Extracorporeal Life Support Organization (ELSO) is an international consortium of health care professionals and scientists. ELSO’s goal is to gather data regarding the use of ECMO around the world and to provide support to institutions that are dedicated to using ECMO as a method of resuscitation for their patients. ELSO aims to foster innovation, provide guidelines, expertise, clinical support, and aid with forming a community of practitioners with interest in the field.
Indications and contraindications
ECMO can be used for both respiratory, as well as cardiac, failure depending on its configuration (see below). It is commonly used in neonatal pathology such as meconium aspiration, respiratory distress syndrome, congenital diaphragmatic hernia, persistent pulmonary hypertension, as well as cardiac pathology that will necessitate surgical correction. For adults, indications and contraindications are differentiated based on the primary organ failure as outlined in Table 14.1.
Absolute indications | |
---|---|
Respiratory indications | Cardiac indications |
|
|
Relative contraindications |
---|
|
Absolute contraindications |
---|
|
Configuration
ECMO evolved from CPB technology, with similar features that include:
The main differences between ECMO and CPB are that ECMO does not have a venous reservoir or an arterial filter and the level of anticoagulation required for ECMO circuits is lower than that used for conventional CPB. The other major difference relates to the intended duration and setting of use: ECMO is mainly used in the ICU for days to weeks, as compared to CPB, which is typically used in the operating room for a matter of hours.
Broadly speaking, ECMO is a circuit that allows blood to be drained from a large vein and pumped back to the patient, via either a large vein or an artery, after passing through a gas exchanger for oxygenation and carbon dioxide removal and a heat exchanger for temperature regulation. Cannulae with the biggest possible diameter are preferred, and are usually placed percutaneously using the Seldinger technique and imaging guidance.
ECMO has two possible configurations, namely veno-venous (VV) used mainly for respiratory support, and veno-arterial (VA) that is employed for cardiac support. These basic configurations are shown in Figure 14.2 and 14.3.
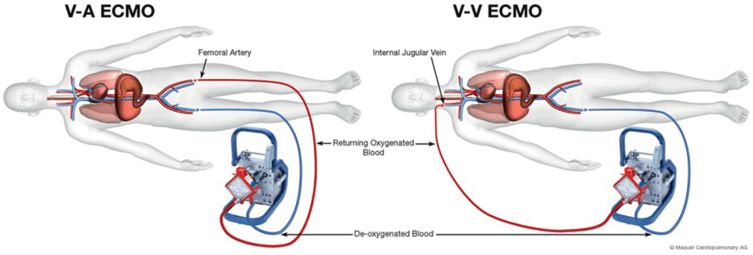
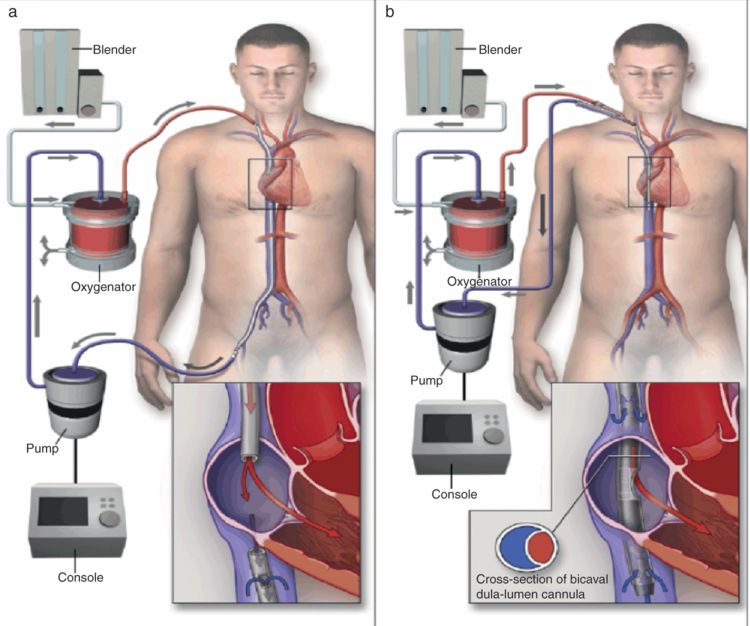
Using VV ECMO, the gas exchange membrane is in series with the intrinsic lung, and is therefore able to provide gas exchange, and ultimately respiratory support. In VA ECMO, the gas exchange membrane is in parallel with the intrinsic lung, and the ECMO system provides replacement for both cardiac and pulmonary function. Both configurations provide respiratory support, but only the VA allows for hemodynamic support.
VV ECMO
Common cannulation sites for VV ECMO are the femoral vein (extending into the inferior vena cava) for drainage and jugular vein (extended into the right atrium) for return (see Figure 14.3). Some oxygenated blood, immediately after being reinfused through the jugular vein cannula, is immediately withdrawn directly through the femoral cannula without reaching the systemic circulation. This phenomenon is known as recirculation. Currently, there is a double-lumen cannula available for single site cannulation with drainage and infusion ports manufactured specifically in order to minimize recirculation. However, in this configuration only up to 5 l/minute flow can be supplied. In patients who require higher flows (e.g. in sepsis) a second cannula may be required in order to optimize gas exchange. If the lungs are not contributing to the gas exchange, blood flows of 3.0–6.0 l/minute may be required to achieve an oxygen saturation (SaO2) between 86% and 92%, since some mixing of the oxygenated and deoxygenated blood occurs.
VA ECMO
VA ECMO is instituted by either cannulating a femoral vein (commonly) or the right atrium for drainage, and the femoral artery or ascending aorta (for central cannulation, see below) for blood return. One of the adverse effects associated with femoral artery cannulation is distal limb ischemia, which can be improved, if not totally resolved, by using an additional arterial cannula distal to the femoral artery to create a shunt to the distal extremity. Peripheral cannulation rather than central cannulation of the atrium is preferred, mainly due to the fact that it can be instituted rapidly at the bedside, with minimal or no sedation required. The disadvantage of peripheral cannulation is that the proximal aortic arch vessels, including the coronaries, will be perfused by retrograde blood flow from the ECMO circuit that is mixed with blood originating from the patient’s intrinsic cardiac output. If the patient has coexisting respiratory dysfunction, it is highly likely that the oxygen concentration of this mixed blood varies substantially from that of the ECMO output, at the location of the arterial cannula, which is beyond the distal aortic arch. Therefore it is important to monitor oxygenation from the right radial artery in order to more accurately assess oxygenation provided to the heart and brain.
Central cannulation is an acceptable alternative, and is convenient in cardiac surgery patients who are being converted to ECMO from CPB. In this situation, the arch vessels as well as the rest of the body are perfused in an antegrade fashion. An intra-aortic balloon pump (IABP) can also be incorporated to aid with left ventricular output, prevent ventricular distention, and possibly ameliorate myocardial stunning. Disadvantages of central cannulation are increased risk of bleeding and infection due to an open chest. Tunneled cannulae that allow for the chest to be completely closed have recently become available; however these do come at an increased cost.
One of the most pressing issues during VA ECMO is the need for close monitoring of left ventricular function, either by observing the arterial waveform for pulsatility or with frequent echocardiographic examinations. Inadequate emptying of the left side of the heart due to poor left ventricular function can lead to dangerous left ventricular distention by blood flowing from the right ventricle and bronchial circulation into the left ventricle. Inotropes and IABP counterpulsation (only in conjunction with central arterial cannulation) can be used to reduce distention. In refractory cases the left ventricle may need to be “vented,” similar to what is done during on-pump cardiac surgery, in order to prevent further myocardial damage.
Components of the ECMO circuit
There are several components of the circuitry that are essential for this system to function, including the console, the pump, the membrane oxygenator, and the blender. The circuit has a drainage limb that takes the blood into the pump, a limb that circulates blood from the pump to the membrane oxygenator, and the return portion that circulates the oxygenated blood past the oxygenator to the body. The pump generates a negative pressure to withdraw blood from the body and a positive pressure to aid with blood return towards the body.
The pump
Since ECMO evolved from the CPB circuitry, the pumps initially utilized were roller pumps. Nowadays most institutions use centrifugal pumps for the ECMO circuitry (see Figure 14.4). The advantages of centrifugal pumps are purported to be:
small size,
low priming volume (32 ml),
fast spinning, up to 5000 revolutions per minute,
less blood trauma,
less platelet activation, and
less pronounced inflammatory response.
Roller pumps are afterload independent and may cause microemboli of tubing debris. Centrifugal pumps are both preload and afterload dependent, so a flow sensor must be introduced into the circuit, since the correlation between the pump speed and the blood flow is not constant. Hemolysis is a real concern, but with evolving pumps, it seems to be less of an issue.
The membrane oxygenator
Several types of membranes have been used for ECMO: silicone, microporous hollow fiber, and poly-methylpentene (PMP) hollow fiber membranes. The disadvantages with the first two include poor efficiency, pulmonary injury, higher transfusion requirement, and plasma leak. The PMP oxygenator is commonly used today, and is a true hollow fiber in which blood and gas are totally separated by the membrane (see Figure 14.5). The advantage is a longer duration of use, and lower incidence of hemolysis. Its role is to allow deoxygenated blood with a high CO2 concentration to enter through one side and to let the CO2 diffuse from blood into the gas phase and O2 to diffuse into the blood, so that oxygenated blood with a lower CO2 concentration exits through the other side (see Figure 14.6). A constant flow of fresh gas (known as sweep gas) is administered into the oxygenator to facilitate O2 and CO2 exchange. The concentration and flow rate of the sweep gas are titrated via the blender (see Figure 14.7), guided by the patient’s arterial blood gases.
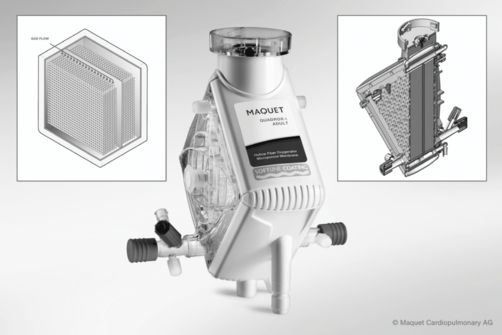
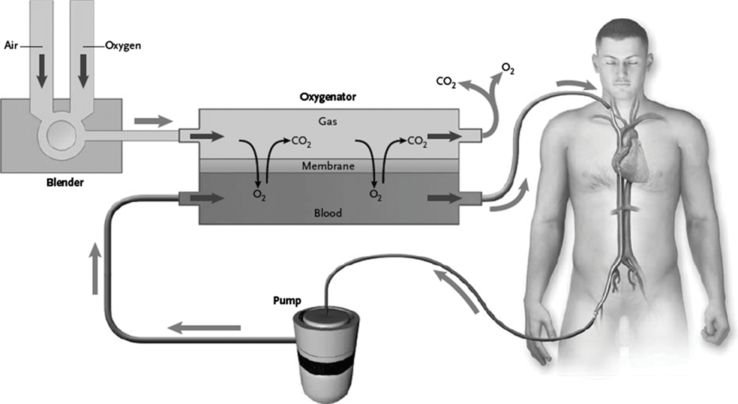
From [NEJM. Extracorporeal membrane oxygenation for ARDS in adults. Brodie D, Bacchetta M., 2011; 356 (20): 1905–14]
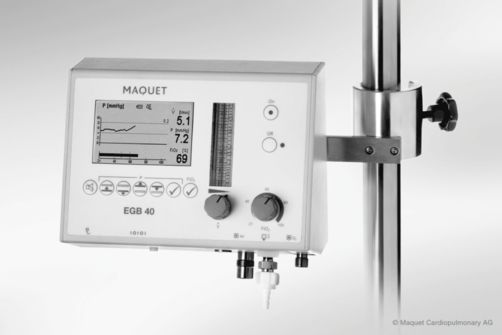
The O2 concentration and the blood flow rate control O2 exchange, while the sweep gas flow rate and gas diffusion gradient control CO2 elimination. Care must be taken when starting ECMO to avoid a very rapid decrease in CO2, and thus negatively affect cerebral blood flow due to respiratory alkalosis. Commonly the sweep gas flow required to maintain a normal acid–base balance is 1 to 2 times higher than the blood flow.
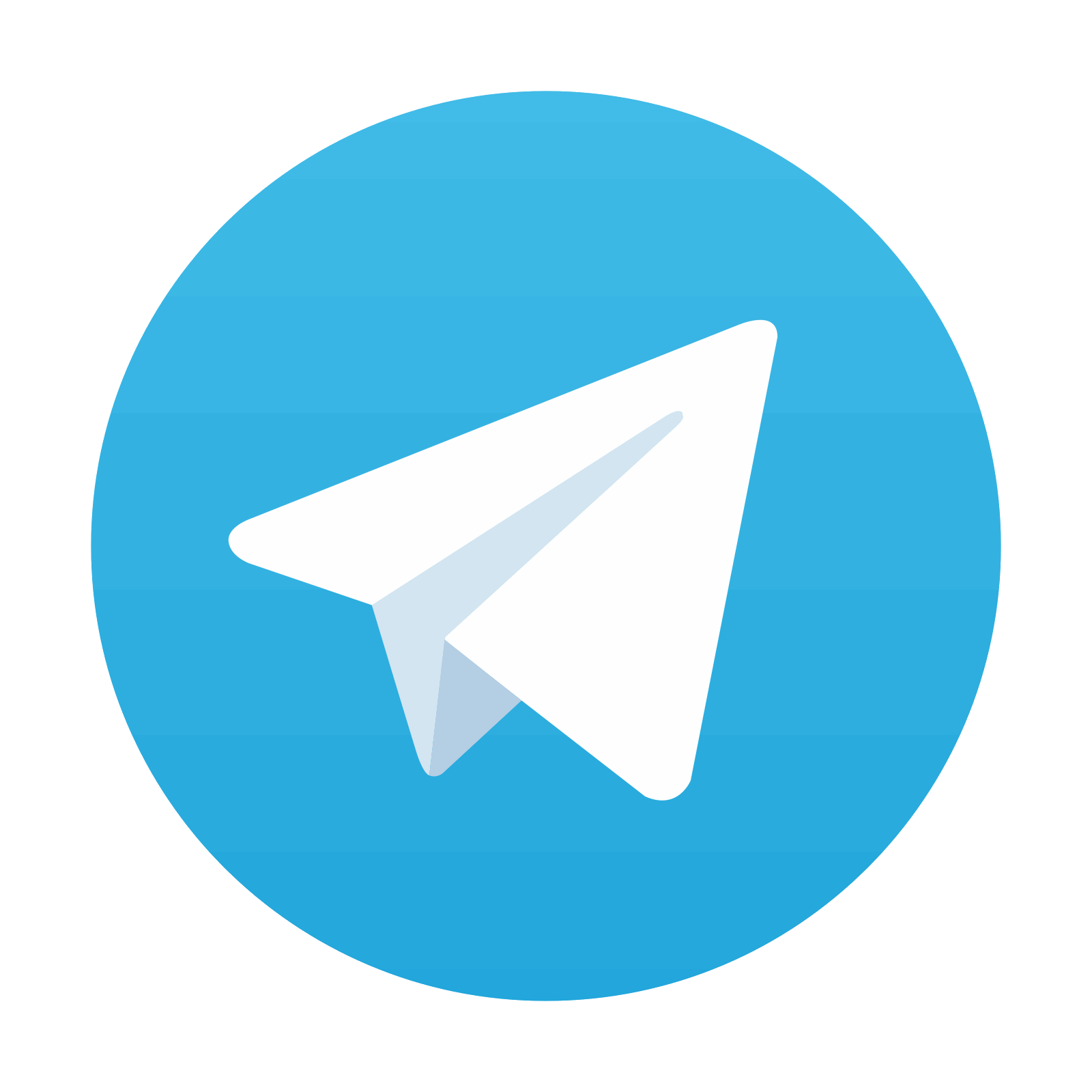
Stay updated, free articles. Join our Telegram channel

Full access? Get Clinical Tree
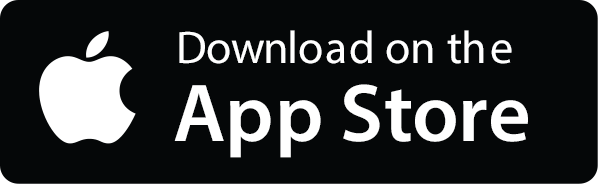
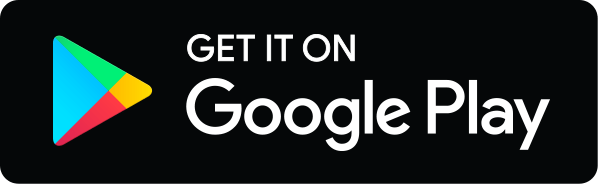