Exercise in Health and Cardiovascular Disease: Introduction
Exercise is beneficial for healthy individuals and those at high risk for cardiovascular disease (CVD), as well as those with manifest CVD. This chapter addresses the hemodynamics and health benefits of exercise and exercise conditioning programs, both in healthy individuals and those with or at risk for CVD.
Acute Hemodynamics
During physical activity, energy expenditure increases, and the compensatory cardiovascular response represents an integration of neural, biochemical, and physiologic factors. The cardiovascular control center is believed to reside in the ventrolateral medulla of the brain and to respond to both central and peripheral inputs. Central impulses arise from somatomotor centers of the brain. Peripheral impulses are generated by mechanoreceptors, found in muscles, joints, and the vascular system; chemoreceptors, found in the muscles and the vascular system; and baroreceptors, found in the vascular system. These impulses are transmitted by autonomic afferent fibers. The central control center regulates cardiac output (CO) and its distribution to organs and tissues according to metabolic demand.
The feed-forward command system located in the motor cortex provides a coordinated and rapid cardiovascular response to optimize tissue perfusion and maintain central blood pressure. This central command provides the greatest control over heart rate (HR) during exercise1 and is also involved in the pre-exercise anticipatory response.2 Stimulation of the central control center by the higher command centers leads to alteration of autonomic tone. Such may explain the influence of “emotions” on the cardiovascular response.
The cardiovascular control center also receives input from peripheral receptors. Stretch and tension of muscular and articular mechanoreceptors trigger afferent impulses that are important in the regulation of the circulatory response to dynamic exercise.3 Muscle chemoreceptors stimulated by products of metabolism influence the control center as well. This reflex neural input (termed the exercise pressor reflex) provides rapid feedback modifying the autonomic outflow in response to physical activity and exercise.4
Vascular baroreceptors are located in the aortic arch and carotid sinuses. They respond to changes in arterial blood pressure and regulate HR by eliciting reciprocal changes in both sympathetic and parasympathetic activity.5 The arterial baroreceptors protect the cardiovascular system from relatively short-term changes in blood pressure, as seen during physical exercise. Cardiopulmonary mechanoreceptors in the atria, ventricles, and pulmonary vessels aid in regulation of the circulatory response. An increase in blood pressure elicits reflex slowing of the heart, and the converse applies during hypotension. During physical activity, this feedback mechanism is altered so that blood pressure can rise. The aortic and carotid bodies contain chemoreceptors sensitive to arterial oxygen, carbon dioxide, and hydrogen ion concentrations. Whereas decreased arterial oxygen levels trigger an increase in arterial pressure, changes in carbon dioxide and hydrogen ion concentration have a relatively small effect.
Circulatory Adjustments with Exercise
The circulatory response to exercise involves a complex series of adjustments resulting in an increase in CO proportional to metabolic demands. These changes ensure that the metabolic needs of exercising muscles are met, that hyperthermia does not occur, and that blood flow to essential organs is protected. Adequate blood flow is delivered to exercising muscles through increased CO and redistribution of blood flow away from the viscera. CO is defined as the product of stroke volume (SV) and HR. The average CO at rest is approximately 5 L/min for both trained and untrained men. In women, the value is approximately 25% lower.
Resting CO increases immediately before the onset of physical exercise as a result of anticipatory changes in the autonomic nervous system resulting in tachycardia and increased venous return. After the onset of exercise, CO increases rapidly until steady-rate exercise is reached. CO then increases gradually until a plateau is achieved. The magnitude of the hemodynamic response during physical activity depends on the intensity and the muscle mass involved. In sedentary individuals, CO during maximal exercise increases approximately four times, to an average of 20 to 22 L/min. In elite-class athletes, the CO can increase eight-fold, to values of 35 to 40 L/min.
Heart Rate Response to Exercise
From rest to strenuous exercise, HR rapidly increases to levels of 160 to 180 beats/min. During short periods of maximal exercise, rates of 240 beats/min have been recorded. The initial rapid increase is likely the result of central command influences or a rapid reflex from muscle mechanoreceptors. The instant acceleration in HR is largely caused by vagal withdrawal. Later increases result from reflex activation of the pulmonary stretch receptors, which trigger increased sympathetic tone and more parasympathetic withdrawal. Increased circulating catecholamines play a role as well. During exercise, changes in HR account for a greater percent of the increase in CO than does SV. SV plateaus when the CO has increased to only half of its maximum. Further increases in CO occur by increases in the HR, although HR response in older subjects can be blunted.
Stroke Volume Changes with Exercise
Two physiologic mechanisms influence SV. Increased venous return elicits enhanced diastolic filling and more forceful systolic contraction. Neurohormonal influences also enhance contractility through direct effects.6
Enhanced Diastolic Filling
Diastolic ventricular filling (preload) is enhanced by slower HR or increased venous return. Increased end-diastolic volume stretches myocardial fibers, enhancing overlap of sarcomere myofilaments and improving ventricular compliance. This in turn results in enhanced contractility and greater SV. It is believed that this mechanism is responsible for increased SV during transition from rest to exercise or from the upright to the supine position. Resting CO and SV are highest in the supine position. Supine SV is nearly maximal at rest and increases only slightly during exercise. In normal supine individuals, increased CO with exercise results predominantly from an increase in HR, with little increase in SV. Venous return to the heart is lower in the upright position, resulting in a lower resting SV and CO. During upright exercise, however, SV can approach maximum SV observed in the recumbent position, usually without an increase in ventricular diastolic dimensions.6,7
Improved Systolic Emptying
Increases in SV during upright exercise most likely occur through the combined effect of enhanced diastolic filling and more complete emptying during systole. Exercise-induced increases in circulating catecholamines enhance myocardial contractility. In the early phase of upright exercise, CO increases because of a simultaneous increase in SV and HR. In the later phases of exercise, increases in HR are primarily responsible for further increases in CO.
Distribution of Cardiac Output during Exercise
Blood flow to tissues is generally proportional to metabolic activity. At rest, approximately 20% of CO is distributed to the skeletal muscle. However, during physical activity, the majority (≥85%) of the increased CO is diverted to the working muscles. This represents an increase from 4 to 7 to 50 to 75 mL/min of blood per 100 g of muscle. Even within active muscle, the increased blood flow is highly regulated with the greatest amount of blood being delivered to the oxidative portions of the muscle at the expense of the tissue with high glycolytic capacity. Local metabolic conditions, as well as neural and hormonal regulation of vasomotor tone, control the shunting of blood to active muscles. The local response is primarily caused by the buildup of vasodilatory metabolites in exercising muscle.
During exertion, parasympathetic activity is withdrawn, and sympathetic discharge is maximal, which results in increased release of norepinephrine from sympathetic postganglionic nerve endings. Plasma epinephrine levels are also increased. As a result, most vascular beds constrict, except those in exercising muscles, influenced by vasodilating metabolites. Blood flow to the skin increases during light and moderate exercise, favoring body cooling. Further increases in workload cause a progressive decrease in skin flow as the increasing cutaneous sympathetic vascular tone overcomes the thermoregulatory vasodilatory response.2 The kidneys and splanchnic tissues extract only 10% to 25% of the oxygen available in their blood supply. Consequently, considerable reductions in blood flow to these tissues can be tolerated through increased oxygen extraction.7 At rest, the heart extracts approximately 75% of the oxygen in the coronary blood flow. Because of limited margin of reserve and increased myocardial demands, coronary blood flow increases four-fold during exercise. Cerebral blood flow also increases during exercise by approximately 25% to 30%.8 During maximal exercise, however, cerebral flow may decrease because of hyperventilation and respiratory alkalosis.
On cessation of exercise, there is an abrupt decrease in HR and CO secondary to withdrawal of sympathetic tone and reactivation of vagal activity. In contrast, systemic vascular resistance remains lower for some time because of persistent vasodilatation in the muscles. As a result, arterial pressure decreases, often below pre-exercise levels, for periods up to 12 hours into recovery.9 Blood pressure is then stabilized at normal levels by baroreceptor reflexes.
Exercise Type and Cardiovascular Response
Different types of exercise impose different loads on the cardiovascular system. Isotonic (dynamic) exercise is defined as muscular contraction of large muscle groups resulting in movement, which induces a volume load to the heart. Isometric (static) exercise is defined as a constant muscular contraction of a smaller muscle group without movement. It provokes more pressure than volume load to the heart. Significant increases in both CO and oxygen consumption (VO2) and a decrease in systemic vascular resistance characterize the load posed by isotonic exercise. In contrast, isometric exercise increases systemic vascular resistance while producing only minimal changes in CO and VO2.10 A third type of exercise is resistance exercise. This is a combination of isometric and isotonic exercise evoked by using muscular contraction with movement, as in free-weight lifting. Most activities, such as sports or those that are job related, combine all three types of exercise (Table 100–1).
Isotonic | Isometric | Resistance | |
---|---|---|---|
Alternative terminology | Dynamic | Static | Resistive |
Example | Running | Static hand grip | Weight lifting |
Oxygen uptake | Greatest | Least | Intermediate |
CO | Greatest | Least | Intermediate |
Peripheral resistance | Greatest decrease | Least decrease | Intermediate |
The response to isotonic exercise is mediated through central and peripheral adaptations that increase oxygen delivery to exercising muscles. In normal sedentary individuals, VO2 typically increases 10-fold from rest to maximal exertion,11 but in world-class athletes, the increase is significantly greater. Maximal VO2 is therefore considered an indicator of the level or degree of conditioning.12
During isotonic exercise, such as running, peripheral vascular resistance decreases, and marked vasodilatation of vessels in exercising muscles occurs in conjunction with vasoconstriction of the splanchnic and renal vessels. In active muscles, local autoregulation in response to hypoxia, decreasing pH, and increased local temperature results in vasodilatation.
During prolonged dynamic exercise, skeletal muscle metabolism is primarily aerobic and requires a significant increase in oxygen supply to meet the increased demand for adenosine triphosphate (ATP). The increased oxygen requirements are met by an augmentation of the local blood flow and improved oxygen extraction.
The cardiovascular response to isometric exercise is different. Less oxygen is required to sustain the contraction of smaller muscle groups. With isometric exercise, the necessary VO2 is maintained with a smaller increase in CO. Increases in regional blood flow are limited by mechanical compression of blood vessels during sustained muscular contraction,13 and regional blood flow can actually decrease. To maintain regional perfusion, a pressor response is evoked, which is mediated, at least in part, by reflexes originating in the contracting muscles.14 The increase in blood pressure is proportional both to the relative muscle tension and the mass of the muscle groups involved.
Stroke volume usually declines as a result of increased left ventricular afterload and the absence of augmented venous return. In its pure state, static exercise represents a pressure, or systolic, load. To maintain the higher CO, the HR must increase, often out of proportion to the metabolic needs of the active muscle groups.
Resistance exercises are activities that use repetitive movements against a resistance, generating a low to moderate increase in muscle tension. The response to resistance exercise is determined by the extent of both the isotonic and isometric components. Physiologic responses are described relative to the percent of maximal voluntary contraction (MVC). Static contractions (<15%-25% MVC) do not fully occlude intramuscular blood flow, and oxygen can still be delivered to active muscles. If one can perform more than 30% MVC with no occlusion of blood flow, there is increased VO2, thus the aerobic or isotonic component of resistance exercise.15
Weight lifting is considered the prototype resistance exercise and is thought to have a high isometric component. Blood pressure and HR responses during weight lifting are proportional to the relative intensity of muscle contraction, the mass of the muscle groups involved, and the duration of the contraction.16 Weight training exercises have been shown to cause a significant increase in blood pressure.17 This is thought to be the result of restricted muscle perfusion and a centrally mediated pressor response caused by enhanced muscle tension. The HR response during maximal upper body resistance exercise is lower than that seen during maximal isotonic exercise.18 This contributes to a lower HR-blood pressure product during maximal resistance exercise compared with maximal dynamic exercise.
Previous concerns regarding the safety of resistance training have been rebutted by several reports that reveal that moderate resistance training programs are safe even in subjects with cardiac disease.19,20 At this time, it is believed that resistance training (done on a regular schedule) is useful for promoting muscle strength, flexibility, and functionality but probably contributes less significantly than does isotonic exercise to overall cardiovascular health and longevity. Resistance exercise should be done with care and in moderation in subjects with aortic or aortic valve disease.
Exercise Conditioning Training
Physical conditioning affects the cardiovascular and musculoskeletal systems in a variety of ways that improve work performance and exercise capacity. Maximal VO2 can increase two- to three-fold through conditioning induced by repetitive periods of dynamic exercise. Increased CO and peripheral adaptations that improve oxygen extraction contribute equally.21 Conditioning alters cardiac structure and function,22 enhancing exercise-induced increases in SV.
At rest, CO is similar for both trained and untrained individuals. Endurance training induces an increase in resting parasympathetic tone23 and reduces resting sympathetic activity. Heart rates below 30 beats/min have been recorded for some healthy athletes. Cardiac output is maintained in such individuals by increased SV.22 Training-induced increase in blood volume and intrinsic myocardial factors have been cited as the source of this enhanced resting and exercise SV (Table 100–2). During exercise, trained individuals achieve a larger maximal CO than do sedentary persons. In untrained persons, there is only a small increase in SV during the transition from rest to exercise, and the major augmentation in CO is induced by tachycardia. The improved cardiac performance after conditioning is secondary to both the Frank-Starling mechanism and augmented myocardial contraction and relaxation.
In previously sedentary individuals, 8 weeks of aerobic training increases SV. This change is associated with increased left ventricular end-diastolic dimension with preservation or even reduction of the end-systolic size.24 The enhanced end-diastolic dimensions are, however, much lower than those of well-trained athletes.25 It is not known whether this discrepancy results from prolonged training, genetic factors, or a combination of both. After cessation of training, changes largely regress within only 3 weeks, which is of great concern in adherence to exercise programs. Unfortunately, cardiovascular conditioning is more easily lost than gained, which accounts for the effort dyspnea often experienced by subjects with resumption of activity after a long absence.
Several factors contribute to the cardiac adaptations of exercise training. Parasympathetically mediated bradycardia prolongs diastolic filling time, and expanded plasma volume also increases preload.26 These changes enhance contractility through Frank-Starling mechanisms. Some studies have shown that endurance training results in increased compliance of the left ventricle,27 which is probably secondary to enhanced early diastolic filling and increased peak myocardial lengthening during exercise (see Table 100–2).28,29 These physiologic changes are accompanied by biochemical and ultrastructural alterations of the myocardial fibers, which have been demonstrated in the hearts of physically conditioned animals. There is an increase in lactic dehydrogenase and pyruvate kinase activity, which enhances the respiratory capacity of the cardiac myocytes. Myocytes enlarge and manifest more mitochondria and myofibrils. Observed ultrastructural changes in the sarcolemma and sarcoplasmic reticulum probably influence intracellular calcium homeostasis and can explain the improved diastolic function of the conditioned heart. Myocardial fibrosis and resultant impairment of ventricular filling related to aging contribute to exercise intolerance.
The cross-sectional area of the epicardial coronary arteries increases in response to exercise. Alterations in the coronary microcirculation have also been identified. Animal studies reveal increased capillary density and capillary-to-fiber ratio with training. Decreased diffusion distance between the capillaries and myocytes has also been observed.30 Some data suggest that training can promote coronary collateral formation in ischemic vascular beds.31 These adaptations may enable the heart to better tolerate transient ischemia and to function at a lower percentage of its total oxidative capacity during exercise.32 Thus, training-induced myocardial adaptations appear to protect against myocardial ischemia.
Skeletal muscle also adapts to long-term training with changes that enhance oxygen extraction. Capillary density and capillary-to-fiber ratio increase.33 The number of mitochondria increases, as do mitochondrial concentrations of oxidative enzymes. Other cellular adaptations include increases in myoglobin levels, increased concentrations of enzymes involved in lipid metabolism, and enhanced adenosine triphosphatase (ATPase) activity.34
Gender Differences
Available data suggest qualitatively similar responses to dynamic and static exercise in both women and men. Some quantitative differences have been demonstrated in teenage girls who manifest a 5% to 10% greater CO than boys of similar ages at any level of submaximal oxygen uptake.35 This is likely related to a 10% lower hemoglobin concentration in women. To deliver the same amount of oxygen, there is a proportionate increase in CO. The gross maximal aerobic capacity in women is approximately 50% lower than it is in men,36 but when adjusted to lean body mass, the difference is only 10% to 15%, a more accurate reflection of gender-related differences. The absolute number of skeletal muscle fibers and the fiber-type distribution are similar in women and men37; however, for reasons that are unclear, muscle fibers in men are hypertrophied relative to those in women, resulting in greater cross-sectional muscle mass. Although strength adjusted to cross-sectional muscle area is similar in men and women, men’s increased muscle mass usually yields greater isometric strength.38
Exercise-induced increases in SV also differ between the genders. Men manifest a progressive increase in ejection fraction with little or no increase in end-diastolic volume; in contrast, women tend to increase end-diastolic volume without a significant increase in ejection fraction.39 This results in a plateau of the ejection fraction during exercise in women compared with a progressive increase in men. Regardless of these differences in men and women, a recently reported meta-analysis of cardiorespiratory fitness in both healthy mean and women revealed no gender differences in all-cause mortality and cardiovascular events in healthy men and women. Those subjects with maximal exercise capacity of 7.9 metabolic equivalents (METs) or more had substantially lower rates of all-cause mortality and cardiovascular events compared with those with less than 7.9 METs.40
Aging Differences
Special considerations must be addressed when prescribing exercise for elderly individuals. In these subjects, maximal end-diastolic volume increases, but maximal HR, left ventricular ejection fraction, and CO are all lower than in young individuals. Coronary disease is common in elderly individuals and can affect the cardiac response to exercise. In addition, the increased potential for exercise-related myocardial ischemia and arrhythmias can increase the risk of adverse events. A critical factor in an elderly (>65 years of age) person’s ability to function independently is mobility. The overall focus for exercise training should be to enhance health-related fitness, reduce the risk of various chronic diseases, and improve overall quality of life. Considerable evidence demonstrates that physical activity, both endurance and resistance-type exercise, can significantly improve these indices and facilitate functional independence and overall well-being.41-47
Studies in community-dwelling older adults have revealed benefits in those who are physically active. One observational study48 involved 3075 adults (52% women, 42% black), ages 70 to 79 years, who performed a long-distance corridor walking test. Those who could complete the test with no difficulty walking had less overall mortality and less CVD as well as less limitation of mobility and disability. Another smaller study49 evaluated free-living activity expenditure including walking, climbing stairs, caregiving, and working for pay. Total energy expenditure was assessed using doubly labeled water and indirect calorimetry in 302 community dwellings of older adults (70-82 years of age) followed over a mean of 6 to 15 years. Results revealed that objectively measured free-living activity is strongly associated with lower mortality in healthy older adults. Therefore, credible data exist to support the benefits of a physically active lifestyle in the aging population.
Elderly persons should ideally undergo a medical evaluation before initiating an exercise program. This assessment should not only include a focused history and physical examination but should also identify psychosocial limitations to participation that are prevalent in this age group.43,50-54 For older, apparently healthy persons desiring to participate in a low- to moderate-intensity activity such as walking, an exercise test is usually not required. However, for more vigorous activities and for all cardiac patients, an exercise test is appropriate. A review of the individual’s medication regimen for possible interactions with activity programs should also be performed.55
As with young persons, the combination of endurance and resistance exercise is best for achieving the health and fitness goals in elderly individuals.56-59 However, some specific comments regarding the intensity, frequency, duration, progression, and mode of exercise for elderly persons are required. The exercise capacity of elderly individuals, both before and after exercise training, is usually lower.41,60,61 Furthermore, because many in this age group have been sedentary for years, specific muscle groups are often markedly deconditioned. In addition, musculoskeletal limitations, particularly arthritis, can be severely limiting. Thus, it is important to prescribe an exercise program with low-level energy expenditure, particularly during the first few weeks, with gradual increases thereafter. In these instances, however, participants can be encouraged to increase the frequency of exercise (with shorter duration), even to perhaps three or four times per day. Higher intensity training must be recommended with caution in this age group because of the potential for musculoskeletal injury.
Those whose exercise duration is limited (<15 minutes per session) because of physical or psychosocial limitations should attempt to exercise more frequently or intermittently. Certain subjects can benefit more because of this intermittent activity. One study supporting this was done in overweight women assigned to the same caloric restriction but randomized in an exercise program to either 40 minutes of continuous exercise or to four 10-minute “bouts” or periods. At the end of the activity program, those in the intermittent (bouts) group lost 8.9 kg of weight versus 6.4 kg in the continuous group. In the intermittent group, there was also improved adherence and a higher VO2 compared with the continuous exercise group.62 Conversely, for those who are not limited, increasing the duration of activity to as much as 45 to 60 minutes per session is valuable for increasing caloric expenditure and improvement of risk factors, including obesity, lipid abnormalities, hypertension, and elevated blood glucose level. Published science advisories and scientific statements from the American Heart Association have summarized current knowledge of the health outcomes of those who are overweight and the impact on cardiovascular risk of patients with type 2 diabetes mellitus.63,64
Many elderly persons have symptomatic concomitant medical and physical limitations (orthopedic, neurologic, and vascular) that can be exacerbated by weight-bearing exercise, especially higher impact activities such as jogging. Even walking can be difficult for elderly persons. Thus, even seemingly innocent activities should be carefully considered for potential adverse effects in this age group, especially when the activity requires individuals to bear their entire weight. Cycle and water exercise can be better tolerated by these subjects.
Implementation of Exercise Training
The type of activity, frequency, duration, intensity, and progression determine the effect of an exercise training program. Epidemiologic studies suggest that moderate-intensity activities, such as brisk walking, performed on a regular basis confer cardioprotection to both men and women.65-69 More vigorous activity can confer greater cardioprotection, but the majority of the benefit is accrued with moderate levels of exertion.66,67,70 Moreover, fitness appears to be a powerful, independent predictor of cardiovascular risk.71,72 High-intensity exercise programs are often associated with poor adherence rates and more musculoskeletal injuries. Thus, a highly structured program of vigorous exercise, especially in elderly individuals, is not generally recommended.
Current guidelines recommend that persons of all ages perform exercise of moderate intensity for 30 to 60 minutes, four to six times weekly or at least 30 minutes of moderate-intensity physical activity on a daily basis.73-76 At the present, only 10% to 20% of the population meets this recommendation.76,77 Because only a small percentage of the population is employed in physically demanding occupations, most need to perform this activity in their leisure time. Examples of recommended activities include brisk walking, cycling, swimming, and active yard work. The duration of any period of activity should be at least 10 minutes, and the accumulated daily duration should be at least 30 minutes. Those who choose shorter exercise periods can benefit (as discussed previously) from the effects of intermittent exercise on improved adherence and greater increases in VO2. In addition, during the recovery period (or periods) in an intermittent (discontinuous) exercise program, additional calories are expended in the postexercise state while the body returns to the resting metabolic state. These calories are not usually counted with those expended with the activity and amount to 20 to 30 kcal per exercise session, which could be doubled if one exercised twice daily.78
Those who are sedentary should be encouraged to initially perform a duration of activity that is “comfortable” and to gradually increase to 30 to 60 minutes of daily activity. People who meet these daily standards and who wish to increase their activity further should be encouraged to do so. Figure 100–1
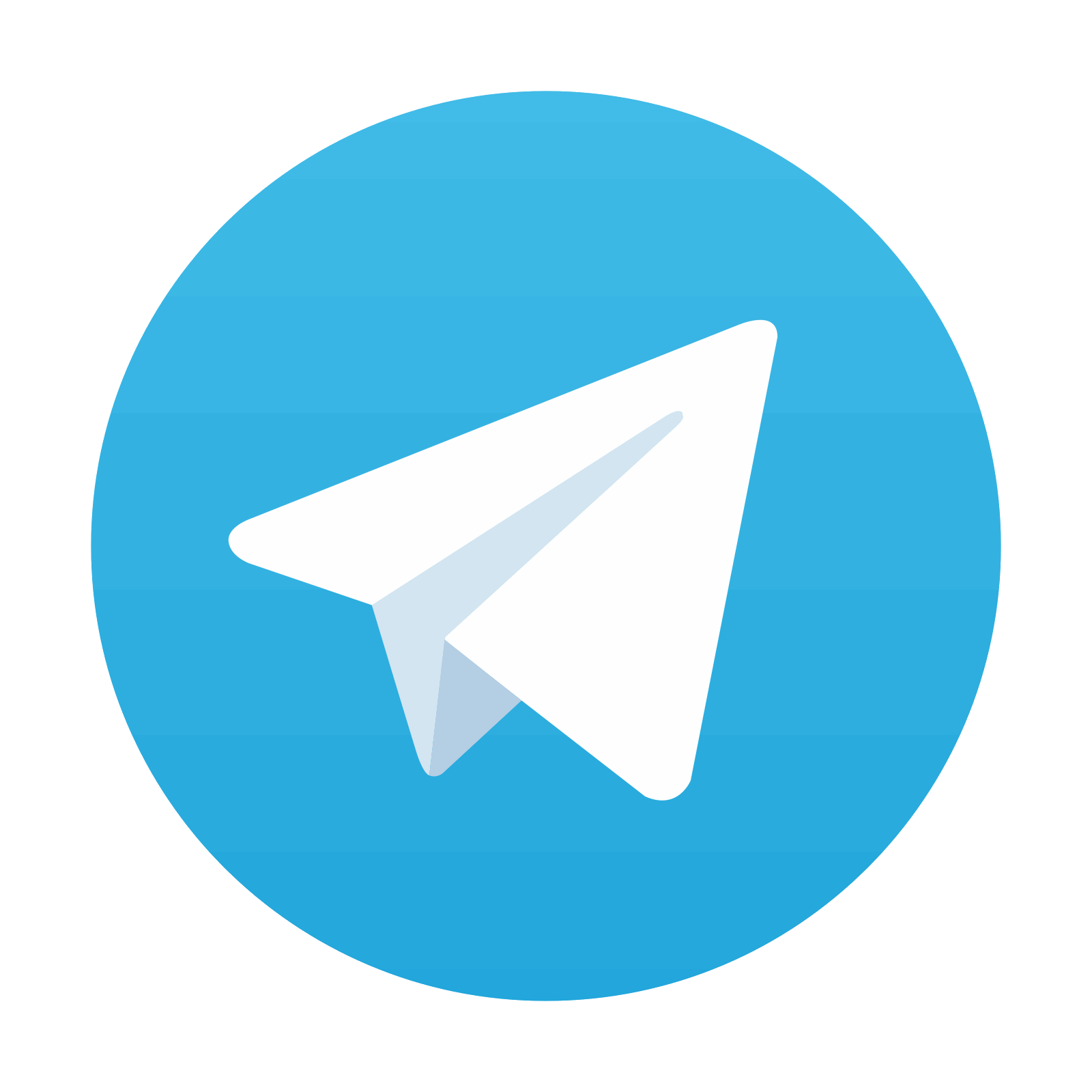
Stay updated, free articles. Join our Telegram channel

Full access? Get Clinical Tree
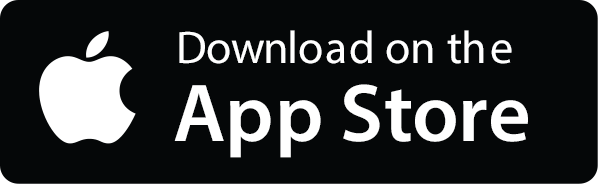
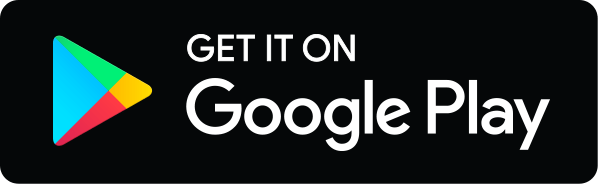
