Chapter 91 Electrical Therapy for Tachyarrhythmias
Future Directions
In their 25 years of existence, implantable cardioverter-defibrillators (ICDs) have moved to the forefront as the best therapy for the prevention of sudden cardiac death (Figure 91-1). Since their inception, they have transitioned from a cumbersome, last-ditch therapy to the standard of care for patients at risk of ventricular tachyarrhythmias. Despite this record of tremendous innovation during this relatively short history, in the minds of some, ICDs have become “good enough” and are on their way to becoming a commodity, with only simplification and reduction of inappropriate shocks being the remaining challenges to differentiate existing products from future products. In contrast to that perspective, this chapter serves to describe the multiple opportunities and challenges that portray an exciting future for devices providing electrical therapy for tachyarrhythmias.
Therapies for Ventricular Tachyarrhythmia Termination
Defibrillation
The most essential therapy an ICD is intended to provide is termination of ventricular fibrillation (VF). The loss of coordinated cardiac contraction associated with fibrillation causes patients to lose blood pressure and consciousness immediately, which, if not quickly reversed, results in death. Electric countershock has long been known to interrupt fibrillation. The re-entrant wavefronts that are the hallmark of fibrillation can only be halted by nearly simultaneous depolarization of the ventricular myocardium. To achieve this, a sufficient amount of energy must be provided such that a voltage gradient of approximately 5 V/cm traverses most of the heart.1 Traditionally, such shocks have been delivered by discharging a capacitor between two electrodes on, in, or near the heart. Early ICDs used monophasic truncated exponential waveforms. Biphasic waveforms proved to have much better defibrillation efficacy and thereby significantly advanced the use of ICDs in the early 1990s.2–6
Capacitors
Today’s ICDs use capacitors in the range of 100 to 140 µF. Connected with transvenous electrode systems, their discharge time constant is in the order of 4 to 6 ms. Mathematical modeling has predicted that the optimal discharge time constant to minimize the energy required for defibrillation is around 2 to 4 ms.7 A shorter time constant could be achieved by simply reducing capacitance below 100 µF. In an ICD, the shock is actually discharged from a bank of multiple (typically three) capacitors. Each capacitor can hold a maximum of approximately 250 V (a property determined by their chemistry, which is most commonly aluminum electrolytic). Stacked in series, the total voltage adds up to approximately 750 V. Using the formula , the total stored energy for a 125 µF system is 35 J. If the discharge time constant were to be optimized by reducing the capacitance to 100 µF or less, the stored energy would be reduced to 28 J or less. While the waveform would be more optimal, the reduction in total stored energy would decrease more quickly with decreasing capacitance than would the energy required to defibrillate, thus reducing the energy safety margin. This dilemma can only be resolved by improvements in capacitor technology such that individual components can hold a higher peak voltage and thereby maintain the same maximum energy despite the reduction in capacitance. Research into new metal oxides or entirely different materials may allow the development of the required capacitor improvements. Recent development has focused on tantalum oxide rather than the currently used aluminum for capacitor construction. In addition to the potential for higher peak voltage, tantalum capacitors offer higher energy density and can be shaped more easily to conform to the desired form of the overall device. These factors are likely to yield devices that are smaller with shapes better suited for implantation.
Defibrillation Waveforms
The truncated exponential waveform used in ICDs has the advantage of being easy to generate from the small components required for an implantable device, but it is not ideally suited for depolarizing excitable membranes. Since cell membranes cannot react completely to a rapid step increase in voltage, slow-rise defibrillation waveforms are theoretically more efficient.8 Studies on humans have shown that ramp waveforms can defibrillate with approximately 20% lower delivered energy and 24% lower peak voltage (Figure 91-2).9 However, with currently available components, the technology required to produce ramp waveforms cannot be incorporated into small ICDs. In the future, new or more efficient technology may facilitate inclusion of new waveforms that may have better defibrillation efficacy, result in less tissue damage, or be associated with less discomfort.
Shock Delivery Systems
Shock delivery leads and electrodes are a vital component of the total ICD system. From experimental and modeling data, the ideal electrodes would deliver a uniform electric field of approximately 5 V/cm across the ventricles for an effective defibrillation shock. To accomplish this task, the earliest commercial ICDs used large surface area epicardial patches placed directly around the heart. Thoracotomy, with its attendant risks, was required for patch placement; therefore, transvenous electrode systems were developed and soon supplanted epicardial leads. Transvenous electrode systems with their less uniform electric fields would likely not have been as effective an alternative, were it not for the significantly improved defibrillation efficacy of biphasic shock waveforms; these systems became available around the same time. Today, almost all ICD implants include a combination of a coil electrode in the right ventricle (RV) and the metal housing of the ICD (the “Can” electrode). A majority of implants also include a coil electrode in the superior vena cava (SVC) incorporated into the same lead as the RV coil, a so-called dual-coil lead. When the SVC coil is electrically coupled to the Can electrode, a dual-coil lead has been shown to have lower defibrillation thresholds than a single-coil (RV only) system in most paired studies in humans, though the differences are relatively small.10–13 Accordingly, many physicians choose to use a single-coil system, which, at least in theory, might offer improved reliability because of the simpler design and improved extractability because of less fibrous growth in the region of the SVC coil. If patients do not meet the required implant criteria with standard transvenous systems, other alternatives include placement of another coil lead in the SVC, azygous vein, or left posterior subcutaneous region (Figure 91-3).14–16
In order to eliminate some of the disadvantages of transvenous lead systems, recent research has explored the efficacy of totally subcutaneous ICD systems. Defibrillation would be achieved by shocking transthoracically between the device and one or more subcutaneous coil electrodes with either an anteroposterior vector or a vector solely on the left anterior quadrant (Figure 91-4).17–19 The feasibility of defibrillation has been established for at least some patients, albeit with higher energy requirements than for traditional transvenous ICD devices; however, published literature on the sensitivity and specificity for the detection of ventricular arrhythmias using an entirely subcutaneous system is sparse. One study on recorded signals has reported sensitivity and specificity comparable with transvenous ICD systems.20 Before understanding the future potential of such a system, other trade-offs, such as increased device density, increased charge times, the difficulty to reliably pace for bradycardia following shock discharge, and inability to use painless anti-tachycardia pacing (ATP) instead of full defibrillation shock energy, must be resolved.
Importance of Shock Reduction
The importance of shock reduction has multiple benefits. Improved device longevity by not delivering unnecessary high power shocks is the more straightforward benefit. In the current ICDs, each shock reduces device longevity by approximately 1 month. Another obvious benefit is enhanced quality of life for patients who are spared discomfort. Research has shown that patients with ICDs suffer from psychological distress ranging from general anxiety to post-traumatic stress disorder, with shocks being a primary factor.21,22 Another potential benefit may be monetary savings, since shocks are a significant reason for hospitalization of patients with ICDs.23 Perhaps the most important factor is a recent analysis showing the significant impact of shocks on patient mortality. Analysis of data from the Multicenter Automatic Defibrillator Implantation Trial (MADIT-II) and the Sudden Cardiac Death in Heart Failure (SCD-HeFT) trial revealed a significant increase in mortality risk associated with both appropriate and inappropriate shocks (Table 91-1).24,25 From the available data, it is unclear whether this association is related to shock therapy itself or only to the episodes that precipitate their occurrence. Analysis of data pooled from multiple trials focused on the use of ATP for shock reduction showed that shocks, but not ATP therapy for appropriately detected VT/VF episodes, were associated with increased mortality; the analysis also concluded that shocks for inappropriately detected VT/VF did not have a significant impact on mortality (see Table 91-1).26 Given the established benefit of SCD prevention with ICDs in both the MADIT-II and SCD-HeFT trials, despite the trials’ reliance on shocks for VT/VF termination, it is possible that the ICD survival benefit was underestimated and, in fact, could have been greater if ATP had been used more aggressively in these trials. Further research into the possible mechanism may allow future ICDs to modify shock therapy to reduce any avoidable consequences associated with shocks.
Anti-tachycardia Pacing
While defibrillation is the most essential therapy for an ICD, ATP for ventricular arrhythmias is actually the most frequently delivered therapy. Even in so-called primary-prevention patients—those without a prior history of VT/VF—monomorphic VT represents more than 90% of the ventricular tachyarrhythmias detected by ICDs (Figure 91-5).27,28 It is interesting to note that the use of ATP and the first implantable anti-tachyarrhythmia devices were, in fact, pacemakers for the treatment of supraventricular tachycardia. It was known at the time that ATP could also be effective for the termination of VT, but the possibility of acceleration of a relatively well-controlled rate to a more hemodynamically significant arrhythmia prevented its widespread use for the treatment of VT until combined devices with high-voltage defibrillation capability were developed.
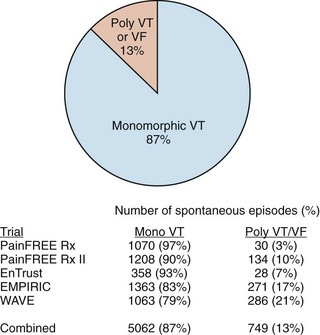
FIGURE 91-5 Distribution of monomorphic ventricular tachycardia (VT) versus polymorphic VT and ventricular fibrillation (VF) in five clinical trials sponsored by Medtronic Inc. (Minneapolis, MN) showing a predominance of monomorphic rhythms amenable to anti-tachyarrhythmia pacing.27–3048 Numbers shown are for spontaneous episodes adjudicated to be ventricular in origin. The detected rhythms are influenced by trial population, device type, and protocol for implantable cardioverter-defibrilator programming, but all studies demonstrated that polymorphic VT and VF represent only a minority of the episodes.
While ATP has long been used for the treatment of slow monomorphic tachycardia as a painless alternative to shock therapy, a series of trials in the past decade have demonstrated the success of empirically programmed ATP in treating fast ventricular tachycardia (FVT) as well. The Pacing Fast Ventricular Tachycardia Reduces Shock Therapies (PainFREE Rx), PainFREE Rx II, and EMPIRIC trials all delivered at least one sequence of burst ATP for fast ventricular tachycardia with a rate of 189 to 250 beats/min (320 to 240 ms).27–29 ATP was effective in terminating 49% to 78% of FVT episodes, providing a significant reduction in the need for shocks. One of the studies, PainFREE Rx II, had a control arm in which patients with similar FVT episodes received shocks. The results demonstrated a significant quality-of-life improvement for patients in the ATP arm compared with patients in the shock arm, which provided solid evidence for the benefit of shock reduction. The EMPIRIC trial had a control arm in which devices received physician-tailored programming as opposed to preset nominal programming. While both arms had similar rates of all-cause shocks, the trial demonstrated that the study’s nominal programming could be used empirically, thereby minimizing time spent during the implantation session. The relative paucity of VT acceleration or syncopal episodes was equally important in the trials. As further evidence becomes available, the use of ATP for FVT as a method of limiting shocks is expected to become more common. Some current ICDs, to eliminate any delay in therapy, incorporate a feature that allows ATP while also charging the capacitors.30 To preserve battery life, these devices can withhold charging once ATP termination has been established successfully.
Enhancements of Anti-tachycardia Pacing
One common belief with regard to the mechanism of ATP failure has been the lack of proximity of the pacing site to the re-entrant circuit responsible for VT. It has been postulated that when pacing from the RV apex, pacing wavefronts are unable to reach the left ventricular site of re-entry. However, recent work has suggested that the number of pulses required to reach the re-entrant circuit via decreasing or peeling back the refractory period may vary depending on the pacing site, but that, under most circumstances, the pacing train will reach the circuit regardless of where the pacing lead is placed. In a paired, randomized comparison of induced VT, ATP applied from both RV and left ventricular (LV) sites were equally effective at termination.31 In addition, in trials comparing RV ATP with biventricular (RV-LV) ATP for spontaneous VT, no significant difference based on pacing site was found.32,33
Given that physically reaching the re-entrant circuit is not dependent on the pacing site, a remaining explanation for ATP failure would be the inability of the pacing train to electrically interact with and terminate the re-entry. Clinical research is under way to exploit available information on the effect of heart rate and return cycle length following entrainment to automatically adjust the pace train, thus improving the results with better conduction into the excitable gap.34,35
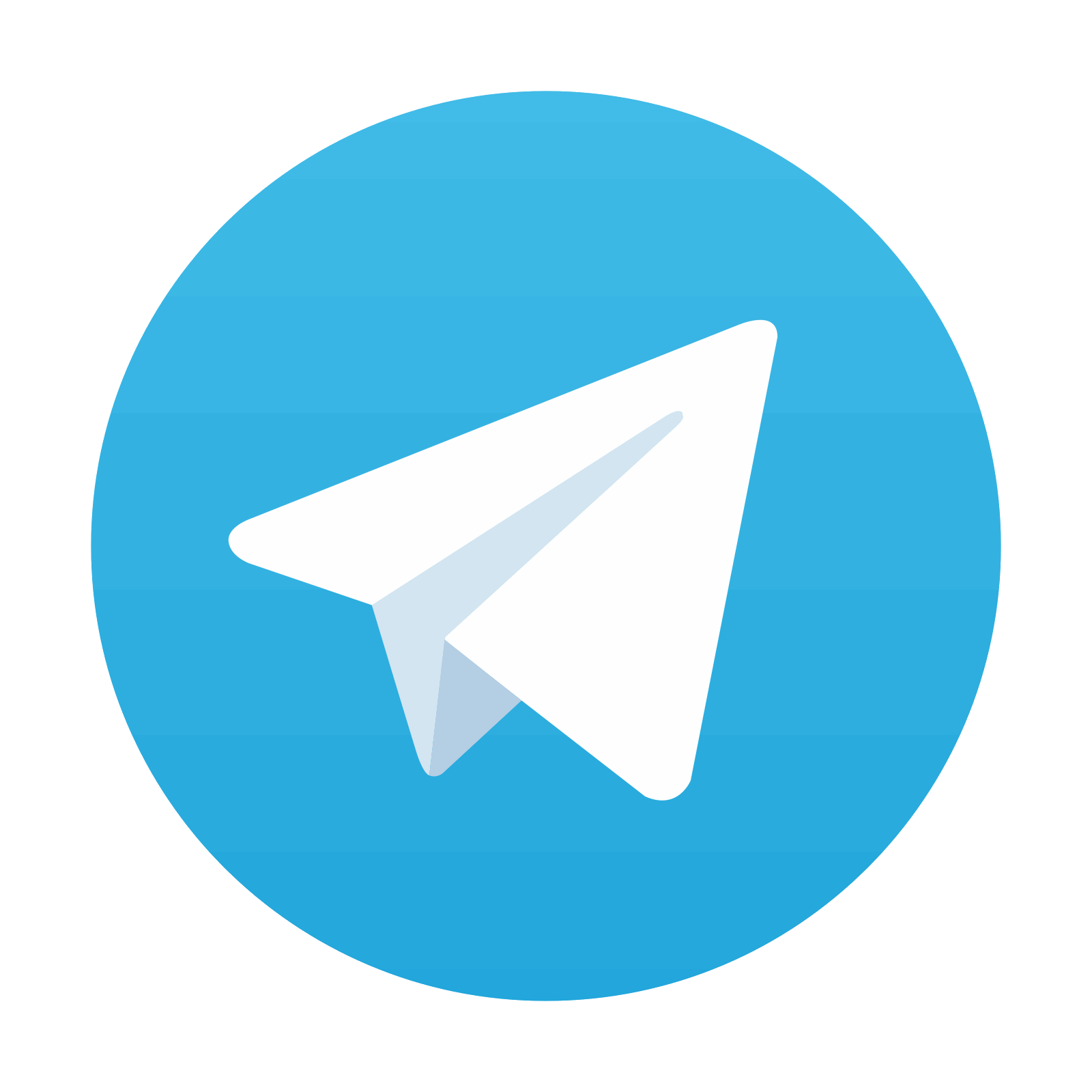
Stay updated, free articles. Join our Telegram channel

Full access? Get Clinical Tree
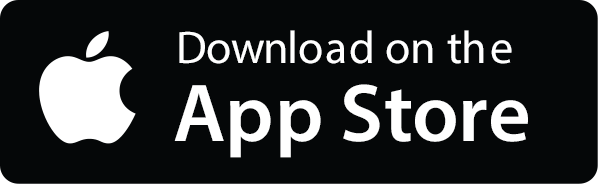
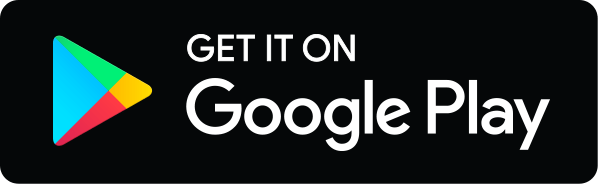