Diabetes
Doron Aronson
Elliot J. Rayfield
Epidemiology
In the past two decades, there has been an explosive increase in the number of people diagnosed with diabetes worldwide. The diabetes epidemic relates particularly to type 2 diabetes, and is taking place both in developed and developing countries. The World Health Organization estimates that the global number of people with diabetes will rise from the current estimate of 150 to 220 million in 2010, and 300 million in 2025 (1,2).
In the United States, almost 8% of the adult population and 19% of the population older than the age of 65 years have diabetes (3), and 800,000 new cases of diabetes are diagnosed per year. Approximately 90% of patients with diabetes have type 2 diabetes, which is now being diagnosed in young people, including adolescents (4). On the basis of fasting plasma glucose levels, one third to one half of cases of type 2 diabetes are undiagnosed and untreated (5).
Impaired glucose tolerance (IGT) is defined as hyperglycemia with glucose values intermediate between normal and diabetes, and affects at least 200 million people worldwide. Approximately 40% of subjects with IGT progress to diabetes over 5 to 10 years (1).
The decline in heart disease mortality in the general American population has been attributed to the reduction in cardiovascular risk factors and improvement in treatment of heart disease. Recent data from the Framingham Heart Study indicate that patients with diabetes have benefited in a similar manner to those without diabetes during the decline in cardiovascular event rates in the US population over the last several decades. Adults with diabetes have experienced a 50% reduction in the rate of incident cardiovascular disease (CVD), although patients with diabetes remained at a consistent, approximately twofold excess of cardiovascular events compared with those without (6).
Both type 1 and type 2 diabetes are powerful and independent risk factors for coronary artery disease (CAD), stroke, and peripheral arterial disease (7). Atherosclerosis accounts for 65% to 80% of all deaths among North American diabetic patients, compared with one third of all deaths in the general North American population (8). More then 75% of all hospitalizations for diabetic complications are attributable to CVD (8). A history of diabetes is equivalent in risk for death to a history of myocardial infarction, and the combination compounds the risk (9).
Coronary Artery Disease in Type I Diabetes
In type I diabetes, atherosclerosis occurs earlier in life, is more diffuse, and leads to higher case fatality and shorter survival (10). The earliest manifestations of CAD occur late in the third decade or in the fourth decade of life regardless of whether diabetes developed early in childhood or in late adolescence (11). The risk increases rapidly after the age of 40, and by the age of 55 years, 35% of patients with type 1 diabetes die of CAD. The protection from CAD observed in nondiabetic women is lost in women with type 1 diabetes (11,12).
The degree of chronic hyperglycemia is related to the progression of CAD in type 1 diabetes (10). Uncontrolled diabetes is associated with greater progression of surrogate markers of
atherosclerosis such as carotid IMT (13) and of coronary artery calcifications (14).
atherosclerosis such as carotid IMT (13) and of coronary artery calcifications (14).
The risk of CAD increases dramatically in the subset of type 1 diabetic patients which develop diabetic nephropathy (11,15). Data from the Steno Memorial Hospital showed that in patients with persistent proteinuria the relative mortality from CVD was 37 times that in the general population while in patients without proteinuria cardiovascular mortality was only 4.2 times higher (15). When nephropathy is superimposed on diabetes, some of the atherogenic mechanisms present in diabetes (hypertension, lipid abnormalities, and a hypercoagulable state) are accentuated (16). Nephropathy also results in accelerated accumulation of advanced glycosylation end products (AGEs) in the circulation and tissue that parallels the severity of renal functional impairment (17).
Coronary Artery Disease in Type 2 Diabetes
The relative risk of CVD in type 2 diabetes compared to the general population is increased two- to fourfold (18). The increased cardiovascular risk is particularly striking in women. A number of studies reported a disproportionate impact of CAD in diabetic women compared with diabetic men (19). Indeed, the usual protection that premenopausal women have against atherosclerosis is almost completely lost when diabetes is present.
Lipoprotein Disorders
The metabolic abnormalities associated with type 1 and type 2 diabetes results in changes in the transport, composition, and metabolism of lipoproteins. Lipoprotein metabolism is influenced by several factors including type of diabetes, glycemic control, obesity, insulin resistance, the presence of diabetic nephropathy, and genetic background (20). Abnormalities in plasma lipoprotein concentrations are commonly observed in diabetic individuals, and have a profound impact on the on the atherosclerotic process.
TABLE 4.1 Lipoprotein Abnormalities in Diabetes | ||||||||||||||||||||||||||||||||||||
---|---|---|---|---|---|---|---|---|---|---|---|---|---|---|---|---|---|---|---|---|---|---|---|---|---|---|---|---|---|---|---|---|---|---|---|---|
|
Lipoprotein Profile in Type 1 Diabetes
Glycemic control is the chief determinant of lipoproteins profile in patients with type 1 diabetes. In well to moderately controlled diabetes, lipoprotein levels are usually within the normal range. In patients with poor control, triglycerides are markedly elevated, low-density lipoprotein (LDL) is modesty increased (usually when HbA1c >11%), and high-density lipoprotein (HDL) levels are decreased (Table 4.1). Hypertriglyceridemia and hypercholesterolemia are readily reversible with intensive insulin therapy, and HDL levels may be higher than in normal controls (21).
Lipoprotein Profile in Type 2 Diabetes
The dyslipidemia in type 2 diabetes results from a complex interaction between an insulin-resistant state, obesity, and hyperglycemia (20), and is often present for years before the development of fasting hyperglycemia and the diagnosis of type 2 diabetes. The typical lipoprotein profile associated with type 2 diabetes includes high triglycerides, low HDL, and normal LDL levels (see Table 4.1). However, the composition of LDL particles is altered, resulting in a preponderance of small, triglyceride-enriched and cholesterol-depleted particles (small, dense LDL). This lipoprotein profile has been termed atherogenic lipoprotein phenotype, and is also characteristic of the metabolic syndrome and obesity (22,23).
The most consistent change is an increase in very low-density lipoprotein (VLDL) triglyceride levels (24,25). HDL levels are typically 25% to 30% lower than in nondiabetics and are commonly associated with other lipid and lipoprotein abnormalities, particularly high triglyceride levels.
Very Low-Density Lipoprotein Metabolism in Diabetes
Hypertriglyceridemia in type 2 diabetes results from high fasting and postprandial triglyceride-rich lipoproteins, especially VLDL (24), which is the consequence of both overproduction and impaired catabolism of VLDL (25). Increased VLDL production is almost uniformly present in patients with type 2
diabetes and hypertriglyceridemia, owing to an increase in free fatty acids (FFA) flux to the liver (25). Because maintenance of stored fat in adipose tissue depends on the suppression of hormone-sensitive lipase by insulin, insulin deficiency, or resistance results in increased hormone-sensitive lipase activity and excessive release of FFA from adipocytes. Because FFA availability is a major determinant of VLDL production by the liver, VLDL overproduction and hypertriglyceridemia ensues (25,26) (Fig. 4.1).
diabetes and hypertriglyceridemia, owing to an increase in free fatty acids (FFA) flux to the liver (25). Because maintenance of stored fat in adipose tissue depends on the suppression of hormone-sensitive lipase by insulin, insulin deficiency, or resistance results in increased hormone-sensitive lipase activity and excessive release of FFA from adipocytes. Because FFA availability is a major determinant of VLDL production by the liver, VLDL overproduction and hypertriglyceridemia ensues (25,26) (Fig. 4.1).
In type 2 patients with more severe hypertriglyceridemia, VLDL clearance by lipoprotein lipase (LPL), the rate-limiting enzyme responsible for the removal of plasma triglyceride-rich lipoproteins, is also impaired (24). LPL requires insulin for maintenance of normal tissue levels, and its activity is low in patients with poorly controlled type 2 diabetes (24). The result is enzymatic activity insufficient to match the overproduction rate, with further accumulation of VLDL triglyceride.
Increased fatty acid flux to the liver also results in the production of large triglyceride-rich VLDL particles because the size of VLDL is also mainly determined by the amount of triglyceride available. VLDL size is an important determinant of its metabolic fate (see below).
High-Density Lipoprotein Metabolism
Decreased HDL levels in diabetes are closely related to the abnormal metabolism of triglyceride-rich lipoproteins (24,25,26) (Fig. 4.2). During lipolysis of chylomicrons and VLDL, surface components (free cholesterol, redundant phospholipids, and apolipoproteins) are transferred into the HDL fraction. These components may enter nascent discoid HDL particles secreted by the liver. The free cholesterol is esterified by lecithin cholesterol acyl transferase to generate mature spherical HDL. Alternatively, these surface components may be incorporated into preexisting HDL particles. The latter process results in an increase in size and decrease in density of HDL particles, leading to the conversion of preexisting HDL3 (triglyceride depleted) to HDL2.
In diabetes, decreased HDL synthesis is related to the decreased LPL activity because the rate of HDL2 formation depends on the rate of flux of surface components from lipolysis of triglyceride-rich lipoprotein (see Figure 4.2). When LPL-mediated VLDL catabolism is inefficient, less surface material is transferred to HDL, impairing HDL formation.
Increased catabolism of HDL in diabetes also occurs because increased secretion of VLDL into plasma promotes the transfer of triglycerides from these lipoproteins to HDL in exchange for cholesteryl ester. This exchange occurs in plasma, and is facilitated by cholesteryl ester transfer protein (CETP), generating a triglyceride-enriched (and cholesteryl ester–depleted) HDL2. This particle is highly susceptible to catabolism by hepatic triglyceride lipase (HTGL), an enzyme found primarily on endothelial cells of hepatic sinusoids (25). HTGL has both triglyceride hydrolase and phospholipase activity, and generates smaller HDL3 particle, which are depleted in triglycerides and phospholipids (see Fig. 4.2).
Low-Density Lipoprotein Metabolism
In diabetes, although the absolute number of LDL particles is normal, alterations in LDL clearance and susceptibility to oxidative modification result in an increase in LDL atherogenic potential. The composition of LDL particles is altered, resulting in a preponderance of small, triglyceride-enriched and cholesterol depleted particles (22,23). These particles are more susceptible to oxidative modification, are particularly prone to induce endothelial dysfunction, and easily penetrate the arterial wall.
The formation of small, dense LDL in diabetes occurs in a similar fashion to the increased formation of small and dense HDL3, as described. CETP mediates the exchange of triglyceride from VLDL for cholesteryl ester in LDL. If sufficient LDL cholesteryl ester is replaced by triglyceride from VLDL then when the particle comes into contact with hepatic lipase, hydrolysis of newly acquired triglyceride in LDL and HDL by HTGL in turn decreases the size of LDL particles (27). The symmetry of the mechanisms for the formation of small, dense species of LDL and HDL (see Figure 4.2) helps to explain why low HDL levels and a preponderance of small, dense LDL are associated with diabetes and the metabolic syndrome and why HDL cholesterol level is strongly correlated with LDL size (27).
The glycosylation process (see Advanced Glycosylation End Products) occurs both on the apoprotein B (Apo B) (28) and phospholipid (29) components of LDL. Glycosylation of LDL Apo B occurs within the putative LDL receptor–binding domain, and impairs LDL-receptor–mediated LDL clearance.
Advanced glycosylation of an amine-containing phospholipids component of LDL is accompanied by progressive oxidative modification of unsaturated fatty acid residues and confers increased susceptibility of LDL to oxidative modification (29).
Endothelial Cell Dysfunction
Endothelial dysfunction can promote both the formation of atherosclerotic plaques, and the occurrence of acute events. One of the hallmarks of vascular disease in diabetes is endothelial dysfunction, which contributes to the initiation, progression, and clinical presentation of the atherosclerotic process in these patients.
Impaired Endothelium-Dependent Vasodilation
Impaired endothelium-dependent relaxation is a consistent finding in animal models and in human diabetes (30,31
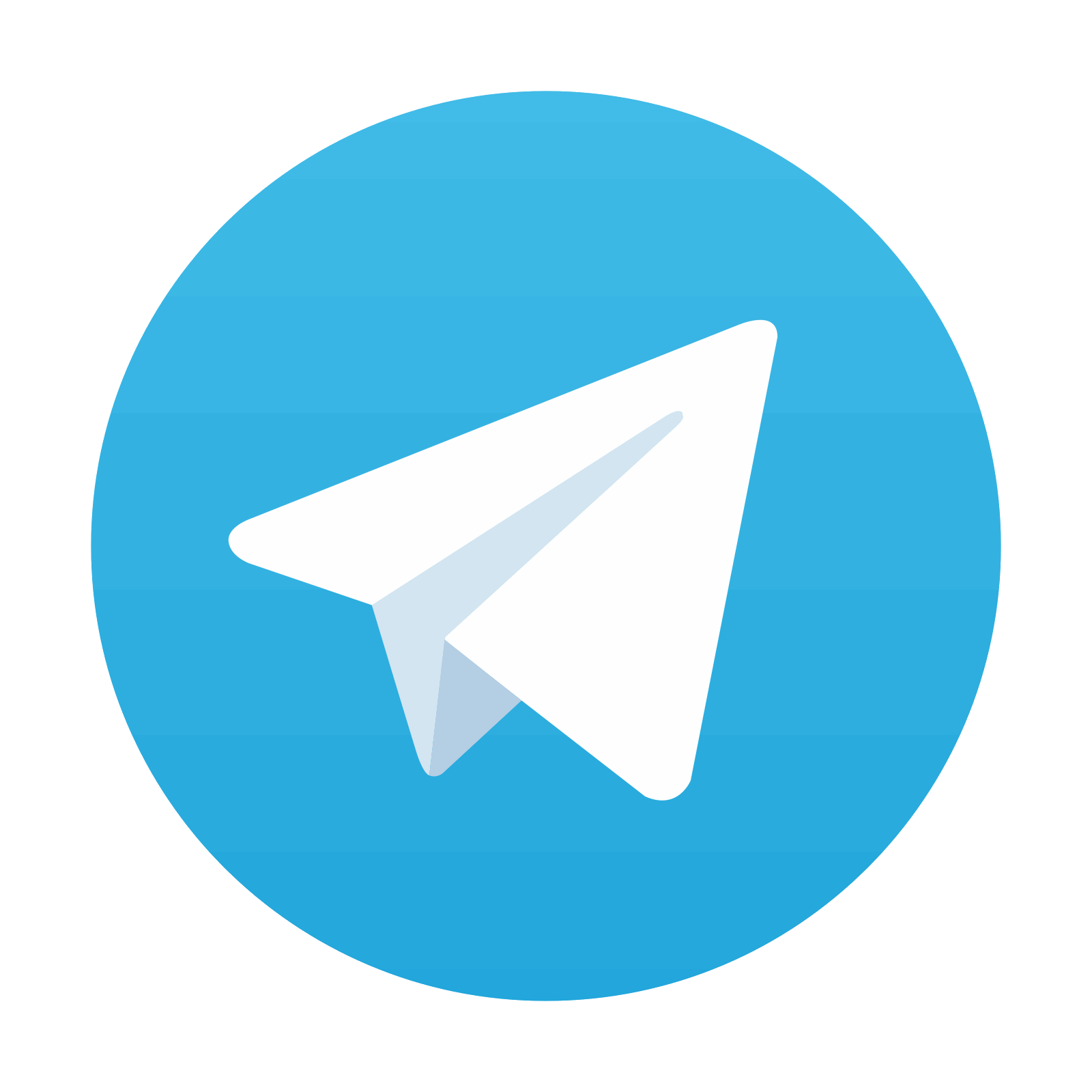
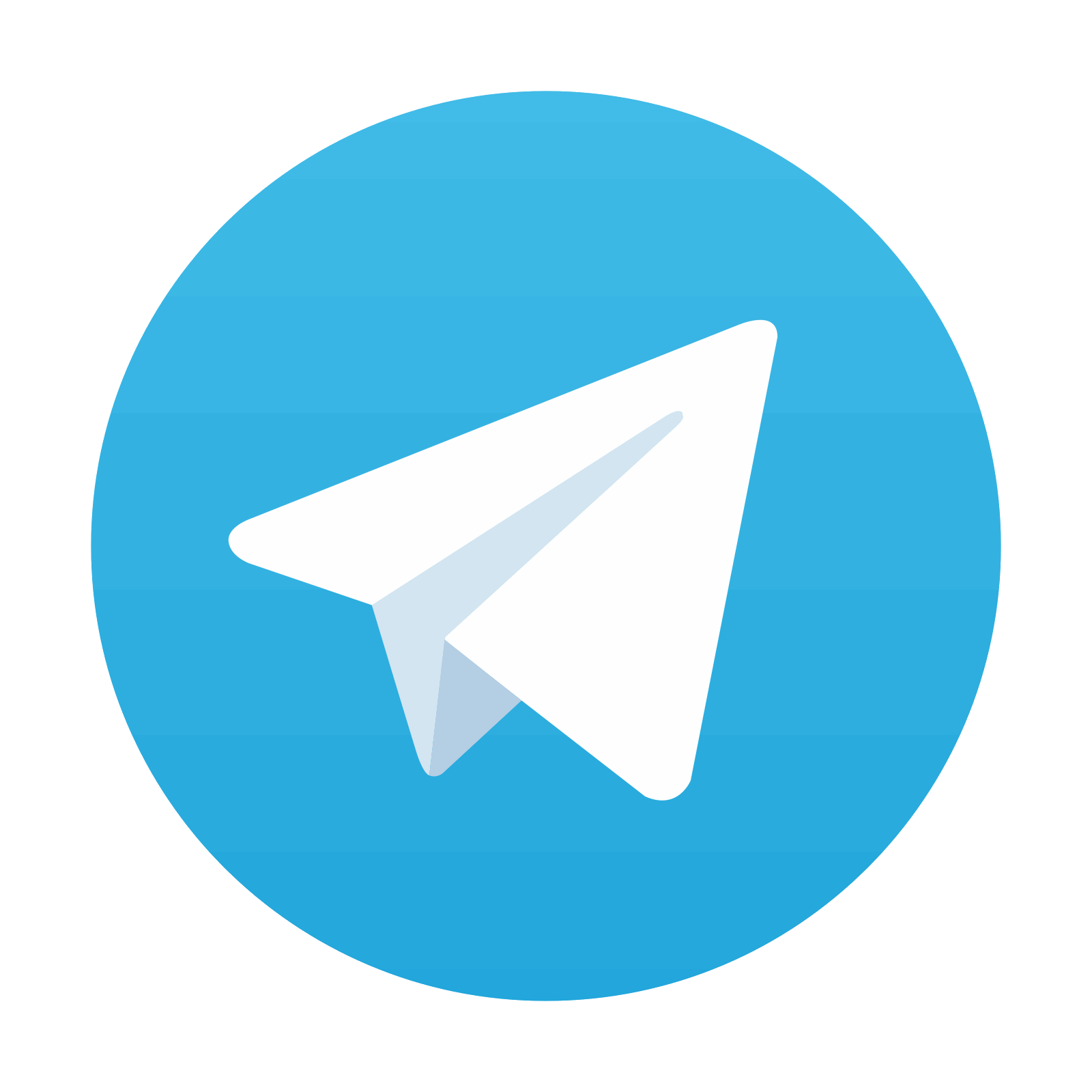
Stay updated, free articles. Join our Telegram channel

Full access? Get Clinical Tree
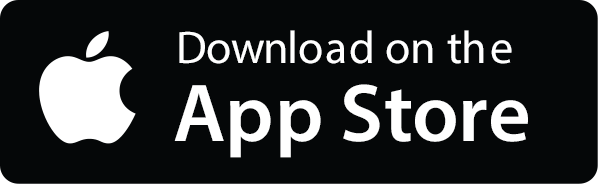
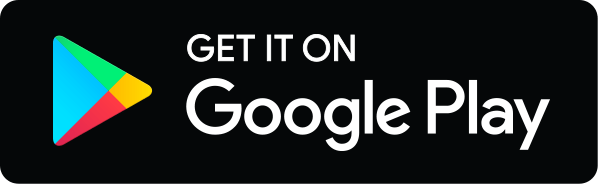
