CHAPTER 61 Critical Care for the Adult Cardiac Patient
IMMEDIATE POSTOPERATIVE CARE
Initial Evaluation
Initial Electrocardiogram
The initial electrocardiogram (ECG) can be difficult to interpret because of lead placement changes related to surgical dressings, cardiac pacing, and the common presence of conduction abnormalities. Right bundle branch block and first-degree atrioventricular (AV) block occur frequently but usually resolve in the first few postoperative hours. Establishing and maintaining normal sinus rhythm with an adequate rate is particularly important in the early postoperative period, when cardiac function may be impaired because of intraoperative ischemia or because of temporary diastolic dysfunction resulting from myocardial edema.
Body Temperature
Initial hypothermia is the rule, and it is best avoided by expeditious surgery and adequate rewarming while the patient is on cardiopulmonary bypass. Hypothermia compromises cardiac function, causes shivering and excessive metabolic demand, interferes with normal coagulation, and can aggravate or cause rhythm disturbances.1 Blankets and other auxiliary warming devices should be employed, particularly in the bleeding patient receiving cold intravenous solutions and blood products. Fever, particularly in the early postoperative period, is poorly tolerated. It should be treated aggressively with antipyretics and possibly with intravenous steroids if there is hemodynamic compromise.
Lines
All indwelling lines must be confirmed to be functional, particularly those delivering vasoactive drugs. Transport of the patient can partially or completely dislodge intravenous lines, many of which were outside the drape and unobserved during surgery. Hemodynamic instability resulting from failure of drug delivery and tissue injury resulting from drug infiltration must be avoided.
Cardiac Surgery with Cardiopulmonary Bypass
Cardiopulmonary bypass (CPB) induces many of the physiologic changes apparent in the postoperative patient, and its duration is a primary determinant of the rapidity of recovery. CPB nonspecifically activates the inflammatory system.2 This phenomenon begins with the interaction of heparinized blood and the bypass circuit.3,4 Generalized complement activation is seen, with elevations in C3a and C5a anaphylatoxins after discontinuation of CPB.3,5–9 This activation can lead to pulmonary sequestration of leukocytes3,6,10 and the production of superoxides and other products of lipoxygenation. This causes further leukocyte activation and the generation of leukotactic circulating factors that increase the local inflammatory response.11,12 Elevations in tumor necrosis factor, interleukin (IL)-1, and prostaglandin E1 have been described.13–15 The generalized inflammatory reaction seen after CPB may change vascular permeability and cause pulmonary hypertension as well as bronchial hyperreactivity.16 Together with transient elevations in left atrial pressure, reduced oncotic pressure from hemodilution and increased vascular permeability also contribute to increased pulmonary shunt from the resultant extravascular water.17
Platelets are activated by shear forces, heparin, foreign surfaces, and activated thrombin. Thrombin is a potent platelet agonist. Despite full anticoagulation with heparin, thrombin continues to be generated.18,19 The clinical consequences of platelet activation include generation of microemboli, thrombosis, and bleeding from diminution of platelet number and function.20 Additionally, vasoactive substances may be liberated from platelets in response to CPB or protamine infusion, which can cause pulmonary hypertension and systemic hypotension.21
Neutrophil activation occurs consequent to the complex interaction of blood with the CPB circuit. Proinflammatory mediators (e.g., IL-1, -2, -6, and -8 and tumor necrosis factor alpha [TNFα]), complement anaphylatoxins (C3a and C5a), plateletactivating factor (PAF), and leukotriene-B4 (LTB4) increase the number of cell surface adhesion molecules and promote neutrophil adhesion to the pulmonary endothelium. Neutrophil adherence and transmigration into the lung occurs under the influence of IL-8. Proteolytic enzyme and oxygen free radical release from activated neutrophils promote further tissue injury and inflammatory activation of endothelial cells.22
Endothelial cells are a central player in inflammation and coagulation. Specific agonists responsible for endothelial activation have been characterized and include IL-1β, TNFα, C5a, and thrombin.9,23 Endothelial cell activation results in a procoagulant, vasoconstrictive, and proinflammatory response. Once activated, the anticoagulant properties of the endothelial cell are dramatically reduced, with loss of heparan sulfate proteoglycan, conformational changes in the endothelial cell (which result in increased exposure to tissue factor and von Willebrand’s factor in the cellular submatrix), increased expression of tissue factor and plasminogen activator inhibitor type 1, and loss of thrombomodulin. The vasoconstrictive effects occur through the production of endothelin-1 and thromboxane A2. Proinflammatory changes are seen with increased expression of P and E selectins and consequent recruitment and sequestration of inflammatory cells.4
A specific manifestation of the inflammatory response in cardiothoracic surgery is the post-pericardiotomy syndrome.24 This syndrome, occurring in 10% to 30% of patients, is a self-limited condition beginning in the second or third week after surgery. It is associated with fever and pleuritic, precordial, or substernal chest pain.25 Chest radiographs in patients with post-pericardiotomy syndrome commonly show pleural and pericardial effusions (Fig. 61-1). This syndrome has been associated with specific reactive antibodies and may be seen after any operation that violates the pericardium.25,26 It is treated with nonsteroidal anti-inflammatory agents, although corticosteroids may be necessary in severe cases.27,28
Cardiac Surgery without Cardiopulmonary Bypass
On-pump coronary artery bypass surgery has been demonstrated to reduce symptoms and prolong life; however, this still comes at some cost. Neurocognitive dysfunction (50% to 75%), atrial fibrillation (30%), transfusion (30% to 90%), stroke (2%), and death (2% to 5%) continue to temper the success of conventional coronary artery bypass surgery.29 Because CPB is perhaps the most invasive aspect of conventional coronary revascularization, and because morbidity of coronary revascularization can been attributed to the bypass circuit, hypothermic cardiac arrest, aortic cannulation, and cross-clamping,30 surgeons are now using advanced stabilizing devices to perform coronary anastomoses on the beating heart without CPB. With this approach, through a standard sternotomy incision and with available stabilizing systems, complete revascularization of all three coronary distributions can be performed with the heart beating. The term off-pump coronary artery bypass (OPCAB) generally refers to multivessel coronary artery bypass performed off the CPB machine, through a standard sternotomy. This approach has achieved considerable support, with between 20% and 25% of coronary surgery in the United States being performed in this manner.31 Some individual surgeons or institutions are now performing the majority of coronary revascularization procedures without CPB. Although initially a number of cases were considered inappropriate for OPCAB techniques, more and more of these have been successfully performed in this manner. For example, cases of re-do coronary grafting and complete arterial grafting have been successfully performed as OPCABs. Many surgeons, however, still view intramyocardial coronary arteries and severe LV dysfunction as strong contraindications to OPCAB.
Although OPCAB procedures are ultimately focused on reduction of morbidity and mortality, initial studies have sought to confirm equivalent operative mortality and graft patency relative to conventional on-pump procedures. These early studies included low-risk, highly selected patients undergoing one- or two-vessel bypass.30
Despite many randomized controlled trials and three meta-analyses, the issue of off-pump versus on-pump myocardial revascularization has not been definitively addressed and remains an area of controversy. Proponents stress that OPCAB decreases the odds of postoperative atrial fibrillation by up to 42%, transfusions by up to 57%, need for inotropes by up to 52%, neurocognitive dysfunction at 2 to 6 months by up to 44%, and average hospital length of stay by up to 1 day at no measurable risk to the patient.30 However, the concern that fewer grafts tend to be performed with OPCAB than with standard coronary artery bypass graft (CABG) surgery,30 and that freedom from revascularization favors on-pump CABG has tempered global adoption of the OPCAB technique.32,33 Given our current understanding, a consensus statement has been published by the International Society for Minimally Invasive Cardiothoracic Surgery to better define current practices. They suggest OPCAB is a safe alternative to conventional CABG and should be considered specifically in high-risk patients undergoing surgical revascularization and in patients with specifically identified risk factors to reduce perioperative mortality, morbidity, and resource utilization.34
As mentioned, conventional cardiac surgery results in a significant inflammatory response. This response appears to be a nonspecific response that includes complement and leukocyte activation.2,3,5–7,10–12 Although the exact mechanism of activation is unclear, the strongest stimulant for the response is the blood–artificial surface contact that occurs with CPB. This response can be modified by coating the tubing and oxygenator surfaces with biological materials, and by improving the hemodynamic performance of the system. Theoretically, by eliminating the heart-lung machine entirely, this response could be significantly reduced.
At least two studies have now investigated the inflammatory response during heart surgery, comparing groups in which coronary revascularization is performed with and without CPB.35,36 Angelini and colleagues37 looked at four important inflammatory markers: neutrophil elastase (an endopeptidase released with neutrophil activation), IL-8 (a potent neutrophil chemotactic and activating factor), and C3a and C5a (fragments generated with activation of the common complement pathway). These markers were measured at four time-points within the first 24 hours postoperatively. Patients were randomized to two groups: coronary revascularization with CPB and standard cardioplegic arrest, and OPCAB. The OPCAB group demonstrated significantly lower levels of all four markers and had reduced leukocyte, neutrophil, and monocyte counts. Together, these findings strongly support a reduced inflammatory response with OPCAB. The clinical significance of this difference is unclear, because by 4 hours the activated complement components have decreased in the on-pump group to OPCAB levels, which interestingly are still higher than baseline.38 Ideally, larger prospective randomized trials will help determine the clinical impact of reduced inflammatory response.
Coagulopathy and postoperative bleeding represent a major morbidity for cardiac surgery patients. Many surgeons believe that OPCAB procedures will help to reduce these problems. OPCAB requires less intraoperative anticoagulation and avoids blood–artificial surface contact, which should reduce platelet activation and destruction. Furthermore, systemic cooling that may further impact negatively on coagulation function can be avoided with OPCAB. Several studies have compared on-pump procedures to OPCAB and report significant reduction in postoperative bleeding, reduced need for perioperative transfusion, and reduced rate of take-back for bleeding.39 Indeed, when coagulation and fibrinolysis variables have been studied, marked changes in fibrinolytic and coagulation variables occur within the first 24 hours. Conventional on-pump revascularization causes a transient decrease in the platelet count, fibrinogen level, and activation of plasminogen with increased D-dimer formation, which only after 24 hours approximate the levels seen in OPCAB.40 Furthermore, a few studies41,42 have compared the differences seen between OPCAB and conventional revascularization with respect to platelet activation and endothelial activation, and they have not demonstrated any significant differences in platelet function or endothelial activation markers.38
CARDIAC DYSFUNCTION AFTER CARDIAC SURGERY
Low Cardiac Output State
Output and Filling Pressure
Low cardiac output after CPB has historically been recognized as a cause of sudden death.43 Low cardiac output resulting from ventricular dysfunction causes a series of adaptive neurohumoral responses, as well as, geometric changes (dilation and hypertrophy) in the heart. Acute loss of 20% to 25% of functioning myocardium44 causes significant reduction in cardiac output and carries extremely poor short-term45 and long-term prognoses.46
Measurement and therapeutic manipulation of both cardiac output and central filling pressures are critical to the postoperative care of cardiac surgical patients and are predictive of survival (Fig. 61-2).47 Central pressures, cardiac output, and venous saturation are routinely measured by means of a flow-directed pulmonary artery catheter. The cardiac index (cardiac output expressed as liters per minute per meter squared) has a normal range of 2.1 to 4.9 L/min/m2.48 In adults, a cardiac index of at least 2.0 L/min/m2 during the immediate postoperative period is required for normal convalescence.47
Oxygen Delivery
Shock is currently conceptualized as a clinical syndrome resulting from an imbalance between tissue oxygen demand and tissue oxygen supply. The general goals of postoperative care are to prevent shock and provide adequate oxygen delivery. Oxygen delivery of less than 335 mL/min/m2 has been associated with a decrease in oxygen consumption49 and with the development of progressive lactic acidosis.50
Lactic acidosis has been suggested as a metabolic monitor to correlate with total oxygen debt, the magnitude of hypoperfusion, and the severity of shock.51–53 However, a direct relationship to total oxygen delivery has been disputed.54 MVO2 provides a useful index of the adequacy of circulation and reflects to some extent mean tissue oxygen level.55 MVO2 is measured with specialized pulmonary artery catheters, and patients with a saturation of less than 60% or those who demonstrate a decrease of more than 5% suffer more frequent postoperative complications.56 However, others have shown poor correlation between saturation, or trends in saturation, and outcome.57 Rapid changes in whole-body oxygen consumption may reduce its overall predictive value.
Adequate regional oxygen delivery is even more difficult to determine. Organ requirements and hormonally activated reflex changes in regional blood supply may occur in the postoperative state. For example, the kidneys, skin, and resting muscles are blood-supply independent and maintain viability by increased oxygen extraction. The heart and brain, on the other hand, are blood-supply dependent, with near maximal oxygen extraction at rest. Sympathetically controlled reflexes compensate for these differences by a shift of blood flow from the skin and splanchnic region at low circulating volumes.58 Increasingly, the effects of splanchnic hypoperfusion on postoperative complications and persistent acidosis are being recognized.59 Acute changes in regional blood supply may be mediated by differing degrees of sympathetic innervation to precapillary sphincters and arterioles.60 Alterations in the metabolic activity are common after heart surgery. These can be monitored through capnography, which determines the partial pressure of CO2 in expired gases.61 Rewarming, with subsequent peripheral vasodilation, and shivering have been shown to increase metabolic and circulatory demands62 and can be eliminated with paralysis and sedation. This promotes hemodynamic stability and decreases the need for inotropic support.63 Thus, the actual definition of low cardiac output syndrome must include evidence of inadequate oxygen delivery relative to consumption. Clinical evidence of diminished peripheral perfusion and end-organ ischemia must be coupled with the objective measurements of cardiac output and MVO2 to establish the diagnosis.
Preload
Maintenance of adequate preload is fundamental in the postoperative management of cardiac surgical patients (Fig. 61-3). The optimal pulmonary capillary wedge pressure in postoperative cardiac surgical patients is unknown, but a range of 14 to 18 mm Hg has been suggested, with increases in extravascular lung water occurring above this level.64 Preload correlates directly with the force of ventricular contraction, and the result is a ventricular volume change from end diastole to end systole determined by transmural pressure and compliance of the ventricular wall. Pericardial pressure is normally reflected by the right atrial pressure (Fig. 61-4). However, tight closure of the pericardium may adversely affect transmural pressure and decrease stroke volume.37,65 Pulmonary artery wedge pressures and the central venous pressure (CVP), indicating LV and RV volumes, respectively, accurately reflect reduced filling pressures, whereas high filling pressures may be determined by changes in transmural pressure or myocardial compliance rather than accurately reflecting preload. Most postoperative cardiac surgical patients are relatively hypovolemic and have a labile reactive vasculature.66 In the immediate postoperative period, causes of hypovolemia include large urine volumes, ongoing blood loss, and a significant cross-sectional increase in vascular beds with rewarming.
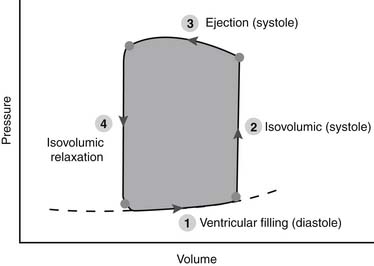
Figure 61–4 Idealized pressure–volume loop for the left ventricle represents a single cardiac cycle.
(Reproduced with permission from Chatterjee K, Parmley WW: The role of vasodilator therapy in heart failure. Prog Cardiovasc Dis 1977;19:301.)
The summation of these physiologic changes is a reduced preload, particularly in the left ventricle. This trend should be anticipated and managed with appropriate volume therapy to prevent precipitous hypotension and low cardiac output. Immediate preload augmentation may be achieved with passive straight leg raising, with transient 8% to 10% increase in cardiac output,67 although this should be viewed as only a temporizing measure and should be quickly supplanted by appropriate volume administration.
Afterload
Postoperative Effects
After a cardiac operation, elevation in afterload is a well-recognized phenomenon. The incidence varies with cardiac pathology, operative procedure, and definition of the resulting hypertension. After valve replacement, the reported incidence ranges between 8% and 12%.68 After myocardial revascularization, afterload is elevated in 8% to 61% of patients.69,70 Increased systemic vascular resistance at the arteriolar level appears to be the major determinant of arterial pressure after coronary artery surgery.71 The etiology of postoperative hypertension remains unclear, but contributing factors include a decreased baroreceptor sensitivity23,66 and an elevated renin-angiotensin activity.72,73
Sympathetic stimulation and elevated levels of catecholamines also have been identified in the early postoperative period.70,74,75 Furthermore, postoperative pain may increase afterload and can be managed with the administration of morphine sulfate.76 Alternatively, some patients develop hypotension postoperatively, which may be related to the systemic inflammatory response or the release of vasoactive substances.
Although pulmonary artery pressures are measured directly (RV afterload), the ascending aortic pressure is inferred by measurements made at a peripheral artery (usually the radial). Systolic amplification may occur, elevating the measured systolic radial artery pressure. However, mean pressures are similar between peripheral and central regions, and these should be considered when managing patients in the postoperative setting. In the control of afterload, autoregulation at various sites should be considered. The central nervous system autoregulates mean blood pressures of 50 to 150 mm Hg.77 A reset lower limit for autoregulation may be higher in hypertensive patients.78 Renal autoregulation requires a mean blood pressure of 70 mm Hg.58 The heart with residual coronary disease also requires adequate mean arterial pressure (65 mm Hg), as do patients with pathologic concentric myocardial hypertrophy.79
Acute afterload reduction in the postoperative period is frequently beneficial (Fig. 61-5). Adequate preload must be achieved before the institution of vasodilating agents. Afterload reduction with low filling pressures often produces a compensatory tachycardia, which may be deleterious. When it is applied to patients with high filling pressures, there is usually no change or a slight reduction in heart rate. Additionally, experimental evidence suggests that afterload reduction, when applied to low or normally filled ventricles, may increase infarct size.80 When applied in a setting of high preload, infarct size may be reduced.81,82 This may have implications in patients after incomplete myocardial revascularization.
Therapeutic Approaches
The degree to which hemodynamic improvement can be obtained with afterload reduction is difficult to predict. Therapeutic results depend on the end-systolic pressure–volume relationship of each patient. However, afterload reduction generally improves cardiovascular function and diminishes preload. Preload augmentation coupled with afterload reduction has additional positive effects on overall cardiac function.83 Afterload reduction also improves forward ejection in patients with residual mitral84 and aortic insufficiency.85
Although various afterload-reducing agents are available, nitroglycerin and nitroprusside are the most frequently used agents in the immediate postoperative period. Nitroprusside may increase ST-segment elevation in perioperative ischemia and cause significant intracoronary shunt.86 Nonetheless, it continues to be the agent of choice for the acute management of postoperative hypertension.87 Nitroglycerin improves coronary collateral flow and may prevent coronary spasm, and it is frequently used for the first 12 to 24 hours after coronary revascularization.86,88
The frequent use of nitroprusside mandates a knowledge of its adverse effects. The lethal dose is approximately 7 mg/kg.89 When high doses are used (greater than 7 μg/kg/min) for prolonged therapy, cyanogen, cyanide, and thiocyanate are potential toxic breakdown products. Signs of toxicity are subtle and include a narrowing of the arteriovenous oxygen difference and the development of metabolic acidosis.90 Thiocyanate levels may be measured under these circumstances, with levels of 50 to 100 mg/L associated with cyanate toxicity, and 200 mg/L may be lethal. Discontinuance and dialysis are the mainstays of treatment, although prophylactic infusion of hydroxocobalamin (25 mg/hr) has been shown to decrease cyanate concentration.
Alternative parenteral agents should be considered when high-dose nitroprusside therapy is necessary: (1) Hydralazine, although longer acting, may also be effective.91 (2) Beta-blockers are effective in the management of postoperative hypertension and are being used more commonly in patients with LV failure. Esmolol, a short-acting selective beta-blocker, may be particularly useful in this setting, although these agents may have a significant negative inotropic effect. (3) Intravenous nitroglycerin may achieve afterload reduction as well as coronary dilation.
Many patients require afterload reduction throughout their postoperative course. Patients with persistent hypertension and with reduced LV function will benefit from long-term afterload reduction and should be converted to oral medications. Chronic afterload reduction, in patients with significant congestive heart failure, has been shown to have a positive impact on survival.92 In one large study of patients with symptomatic congestive heart failure (New York Heart Association [NYHA] class II and class III), a significant reduction in long-term morbidity and mortality was seen in those treated with enalapril, an angiotensin-converting enzyme (ACE) inhibitor.93 Additional studies have confirmed that ACE inhibitors are particularly useful in the management of this problem.94,95
Patients with asymptomatic LV dysfunction (NYHA class I and class II) may benefit significantly from long-term therapy with ACE inhibitors. Although no significant difference in overall mortality was observed when compared with placebo, 37% and 36% decreases in development of symptomatic congestive heart failure and hospital admission for congestive heart failure, respectively, have been demonstrated.96 Although the mechanism of this positive impact has not been completely defined, two-dimensional echocardiographic studies in post–myocardial infarction patients have shown that ACE inhibitors attenuate LV enlargement.97 Nitrates92 and hydralazine98 have also been used but are less effective than ACE inhibitors.99
Heart Rate
The postoperative control of heart rate is important and mandates standard application of temporary, epicardial, bipolar atrial, and ventricular pacing wires in most patients. Normal sinus rhythm, through synchronized end-diastolic preload augmentation, is responsible for approximately 25% of cardiac output in the postoperative setting (Fig. 61-6).100 Changes in heart rate are common after cardiac surgery and include sinus bradycardia, junctional rhythm, and first-, second-, or third-degree heart block. These phenomena are usually transient and may be related to perioperative beta-blockade, intraoperative antiarrhythmics, or metabolic damage during cardioplegic arrest (potassium or magnesium).101,102 Inadequate myocardial protection may cause ischemia of the conduction system.102,103 Permanent injury to the conduction system is most often the result of direct surgical injury during intracardiac procedures. Management of disturbances in heart rate must be individualized. Simple atrial pacing (in the range of 90 to 110 beats per minute) is optimal for sinus bradycardia. With AV nodal blocks, AV pacing with an interval in the range of 150 to 175 msec is usually optimal.104,105 The AV interval also depends on selected heart rate (Fig. 61-7). The normalization of AV synchrony by these means is associated with a loss of the normal ventricular activation sequence, depressing ventricular function at constant preload and afterload by approximately 10% to 15%. Optimal heart rate determination must be individualized for each patient with reference to measurements of cardiac output. When in place, temporary pacing wires constitute a direct current pathway to the heart and must be insulated when not in use. Caution is needed when connecting a rapid-pacing device to the wires to ensure atrial connection. Temporary pacing wires are usually removed in the early postoperative period but may be left in place indefinitely. High pacing thresholds usually develop within 2 weeks, unlike permanent endocardial electrodes. When temporary pacing is not possible, bradyarrhythmias can be treated pharmacologically with atropine or isoproterenol. Alternatively, a pulmonary artery catheter with an additional pacing port or the Zoll transthoracic pacemaker may be employed.106
Inotropic State
Inotropic agents may be necessary to achieve adequate cardiac function (Fig. 61-8) and to maintain peripheral oxygen delivery. Improvement in cardiac function by pharmacologic intervention is generally achieved at the expense of increased myocardial oxygen consumption. Inotropic agents should be considered only after manipulations of heart rate, preload, and afterload have been maximized. In general, myocardial function improves throughout the postoperative course, allowing weaning from inotropic support.107
Various inotropic agents are available and all are administered by either intravenous bolus or continuous infusion. They should be administered through a central venous or pulmonary artery catheter whose intravascular position has been ascertained to prevent perivascular infiltration. Most inotropic agents act through stimulation of adrenergic receptors (Table 61-1). β-Adrenergic agonists mediate an increase in intracellular calcium concentration.108 Clinical experience with multiple inotropic agents has demonstrated synergism109 despite in vitro evidence that the maximal positive inotropic effect of one drug precludes augmentation by another. This may be because of alterations in the adenylate cyclase system in patients with heart failure, or because of alterations in beta-adrenoreceptor density.108,110–114
Nonadrenergic inotropes include digoxin, calcium chloride, phosphodiesterase inhibitors (amrinone, milrinone, and enoximone), and triiodothyronine. The presence of metabolic acidosis may interfere with the effectiveness of inotropic agents.115,116 Notably, most inotropic agents (most importantly, β-adrenergic agonists) have proarrhythmic effects. Improved cardiac output must be balanced against risks of inducing either atrial or ventricular arrhythmias. Patients who require high doses of dopamine, epinephrine, or norepinephrine experience dangerous vasoconstrictive effects because of the α-adrenergic agonist effect of these agents at higher doses. This effect can result in limb, mesenteric, or renal ischemic injury.
Mechanical ventricular support should be considered before the patient suffers significant end-organ hypoperfusion, which may retard ultimate recovery. Insertion of an intra-aortic balloon pump (IABP) via a percutaneous femoral arterial approach is the initial step in mechanical ventricular support. A properly functioning IABP provides increased diastolic coronary perfusion and augmented cardiac output by reducing LV afterload (Fig. 61-9). Greater deterioration of ventricular function may warrant assist devices that provide complete support; these devices are used as bridges to either myocardial recovery or possible cardiac transplantation.
< div class='tao-gold-member'>
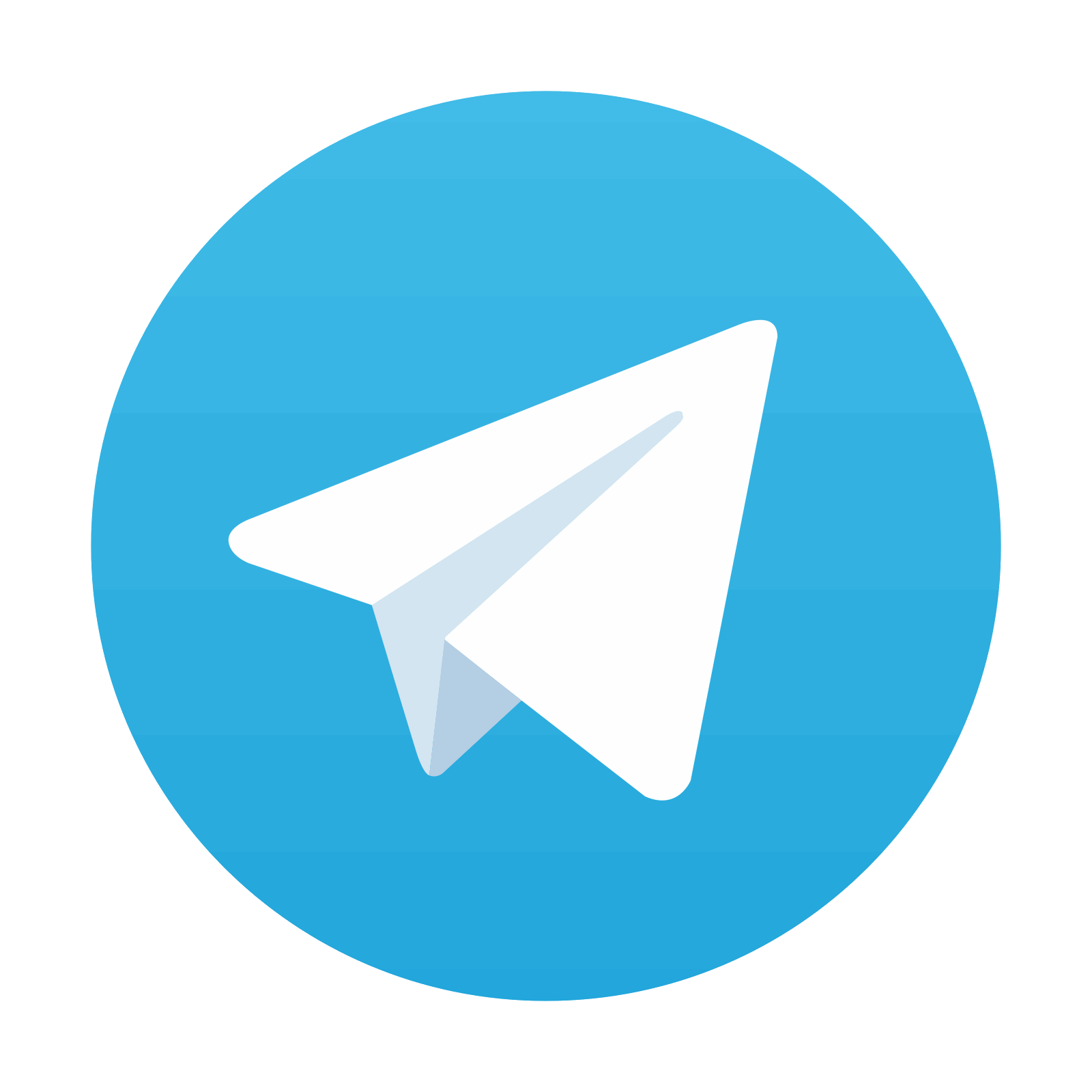
Stay updated, free articles. Join our Telegram channel

Full access? Get Clinical Tree
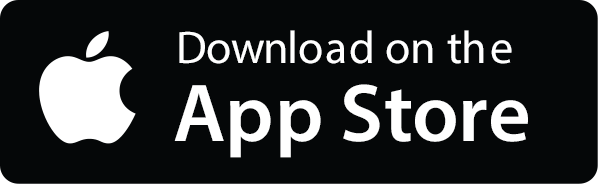
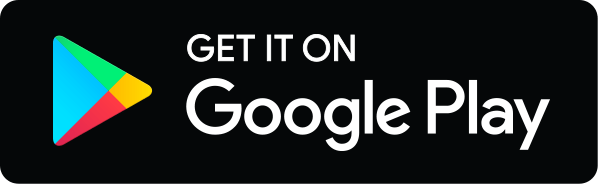