Introduction
Cough is a symptom that has been experienced by every human and is an essential innate protective mechanism that ensures the removal of mucus, noxious substances, and infections from the larynx, trachea, and large bronchi. Cough also minimizes the inhalation of toxic material. Impairment or absence of coughing can be harmful or even fatal in disease. Cough may also be a sign of disease in or outside the airways and lungs and a useful indicator for both patient and physician for initiating diagnosis and treatment of disease processes. When cough itself is persistent and excessive, it can be harmful and deleterious and may need to be suppressed directly.
Because cough is a normal defensive mechanism, it is a symptom experienced by healthy individuals. Epidemiologic surveys have shown that between 11% to 18% of the general population report a persistent cough, but it is not known how much this cough is “normal” or is associated with disease. Reports of chronic cough in these surveys may be due to the presence of cigarette smokers; the exposure of an urbanized population to environmental indoor and outdoor irritants and air pollution; or undiagnosed illnesses associated with cough. The potential contribution of irritants to cough is illustrated by the report of excessive cough in New York firefighters who worked in the dust fall-out of the World Trade Center attacks on September 11, 2001. Cough is also a common presenting symptom to the clinician. In the United States, cough is the most common complaint for which patients seek medical attention and the second most common reason for a general medical consultation; patients with persistent cough constitute about 10% to 38% of a chest specialist outpatient practice. In the United Kingdom, about 3 million prescriptions are written annually for cough preparations by general practitioners, representing a cost of $3 million; this is an underestimate because a vast number of cough mixtures are also bought over the counter without a medical prescription. In the United States, cough and cold medicines sold over the counter between 2007 and 2012 amounted to $2.3 billion.
Definition of Cough
Cough is initiated as a series of respiratory maneuvers that leads to a sudden expulsion of air creating a characteristic cough sound. It usually starts as a deep inspiration, followed by a strong expiration against a closed glottis, which then opens with an expulsive flow of air, followed by a restorative inspiration; these are the inspiratory, compressive, expulsive, and recovery phases of cough ( Fig. 30-1 ). The cough sound in the first of three phases is an explosive sound heard during the expulsive phase consisting of a noiselike waveform. This is followed by an intermediate phase when there is decreased airflow associated with a decreasing sound amplitude. Finally, there is often a third phase called the voiced or glottal phase, that constitutes the second sound, produced by the vibration of a partly closed glottis, which produces a regular periodic noise ( Fig. 30-2 ; listen to the associated and ).



The initial inspiration of cough may be followed by a series of expiratory efforts, with closures of the glottis but not intervening inspirations—a “cough bout” or an “epoch” (see Fig. 30-2 ). Punctate mechanical stimulation of the trachea or larynx causes a brief but strong expiratory effort—the “expiration reflex”—rather than a true cough and, in the case of the glottis, reflex glottal closure ; these reflexes presumably act to prevent or minimize entry of foreign material into the trachea and lungs. Throat clearing, as in postnasal drip, consists more of this expiration reflex or “huff” than a full cough. Not only are there different patterns of “cough,” but these patterns may be differently affected by pharmacologic and physiologic inputs. Cough sounds may have clear harmonic patterns, but the basis of these patterns has been little analyzed and their significance, if any, has not been established. Induced cough is usually preceded by an awareness of airway irritation and an “urge-to-cough,” involving activation of sensory processing and limbic regions in the cerebral cortex. Evoked cough may be either involuntary (reflexively evoked) or voluntary (a behavioral outcome in response to the urge-to-cough), the former mediated by sensory inputs at a brain-stem level and the latter originating in motor regions of the cerebral cortex. It is unclear what proportion of cough in disease is reflexive versus behavioral. Habit cough is a variant of voluntary cough, which may or may not be associated with an urge-to-cough. Once a cough has been induced, there will be secondary feedbacks from both the lungs and the higher parts of the brain, which do not seem to have been analyzed.
Thus, the definition of cough may need to be extended to embrace, or at least consider, these different patterns and neural circuits. This consideration is not a semantic quibble. The various defensive respiratory patterns defined as cough have different afferent and central nervous pathways, but clinical descriptions of cough have seldom differentiated between them. Antitussive drugs could have selective actions on the different neural pathways and therefore have different indications in the clinic.
Physiology
Experimentally, involuntary coughing appears to be initiated only from those structures innervated by the vagus nerve and its branches. These are predominately the larynx and the proximal tracheobronchial tree, but they also include the lower part of the oropharynx and the smaller bronchi, as well as the tympanic membrane and the external auditory meatus. Irritation of all these sites can cause coughing. The one clear exception to vagally mediated coughing is that caused voluntarily ( Fig. 30-3 ); of all the highly complex defense mechanisms, cough is the only one that we can mimic voluntarily and accurately. We can also inhibit it voluntarily. Most patients can suppress their cough for 5 to 20 minutes if they try hard.

Spontaneous coughing can be initiated by a wide variety of inflammatory or mechanical changes in the airways and by inhalation of a large number of chemical and mechanical irritants. Rapid and large changes in lung volume can cause cough, as can psychological effects such as laughter. The extremely wide variety of stimuli that can trigger cough points to a similarly wide range of properties of the sensory receptors that are the starting point of the cough reflex.
Sensory Receptors for the Cough Reflex
The most sensitive sites for initiating cough are the larynx and tracheobronchial tree, especially the carina and points of bronchial branching. Inhaled materials impinge on these points. In experimental animals and humans, it is difficult or impossible to induce coughing from the smaller airways and alveoli. This is teleologically understandable because in the smaller airways even a vigorous cough would not move gas fast enough to cause turbulence and shearing forces at the airway wall, so the cough would be ineffective. In view of the wide range of stimuli for coughing, one would expect that there would be several types of cough-evoking sensory nerves and that the sensory nerve receptors would be “polymodal” (i.e., to respond to a variety of chemical, physical, and pharmacologic mediators). Indeed, at least two types of airway sensory nerve subtypes for initiating cough have been described and these each respond to a range of different stimuli. However, broadly speaking these sensory subtypes can be categorized as either nociceptors, which detect a range of noxious chemical irritants but are relatively insensitive to mechanical stimuli, or mechanoreceptors, which have some (but limited) chemosensory properties but are exquisitely sensitive to punctate (touchlike) mechanical stimuli.
Larynx and Pharynx
Nerve receptors (or perhaps better termed “sensors,” which is used in this chapter) in the laryngeal mucosa are activated by the mechanical and chemical stimuli for cough; their fibers run mainly in the superior and recurrent laryngeal nerves before joining the vagus nerves. Many studies of laryngeal afferent innervation suggest that the sensors for cough belong to the broad group of rapidly adapting receptors (RARs), albeit a different class to the classic RARs described in the intrapulmonary airways and lungs. Laryngeal and pharyngeal mechanosensors are normally silent, as would be expected and, when activated, cause rapidly adapting discharges with an irregular pattern that are conducted in fast-velocity vagal myelinated (Αδ) fibers. Their many stimuli include cigarette smoke, ammonia, ether vapor, acid and alkaline solutions, hypotonic and hypertonic saline, and punctate mechanical stimulation by catheter, mucus, or dust (but not stretch or bronchospasm); all these stimuli can provoke cough. The larynx and pharynx are also innervated by cough-evoking nociceptors. These are unmyelinated slow conducting C-fibers that are characterized by their responsiveness to the chemical capsaicin, the active ingredient in hot chili peppers. Nociceptors are also responsive to a wide range of proinflammatory molecules, which notably include bradykinin, prostaglandins, leukotrienes, proteases, and cytokines, as well as noxious irritants such as capsaicin, acid, nicotine, and acrolein. Laryngeal nociceptor activation during anesthesia has been shown to induce apnea rather than cough, which might indicate that these sensory fibers are inhibitory to cough. However, studies in conscious animals show laryngeal nociceptor activation to be a powerful stimulus for coughing, suggesting that anesthesia confounds the role of laryngeal nociceptors in generating cough responses.
Although strong cough can be induced from the larynx, its denervation or bypass makes little difference to the strength of cough caused by inhaled irritants. As with many protective/defensive mechanisms, the cough reflex displays much “redundancy”—that is, a doubled sensory input for cough does not result in a doubling of the motor response. Activation of sensors in the larynx or pharynx may cause either a “typical” cough, starting with an inspiration, or the expiration reflex, starting with an expiratory effort. The difference is presumably due to various types of cough sensors in the mucosa or the timing of the sensory input to the brain stem relevant to the respiratory cycle. Although cough induced from the pharynx may seem to be the exception to the vagal rule, in that the pharynx is supplied mainly by the glossopharyngeal nerve, there is a small pharyngeal branch of the superior laryngeal (vagal) nerve that could mediate cough, and this seems to be especially active in humans. For example, postnasal drip associated with inflammation due to nasopharyngitis and sinusitis can induce cough possibly due to spread of inflammatory mediators into the larynx.
Tracheobronchial Tree
Touch-sensitive (stretch-insensitive) mechanosensors are also found in the trachea and large bronchi of those species that cough. These sensors have nerve terminals under or within the epithelium ( Fig. 30-4 ), concentrated at points of airway branching; some of them lie close (1 µm) to the epithelium. Their appearance and location suggest that they might be sensitive to intraluminal irritants or particulate matter that physically displaces the epithelium. They are comparable with those in the larynx in their sensitivity to chemical and mechanical irritants. Farther along the bronchial tree in the intrapulmonary airways and lungs, the mechanoreceptors change functionally in that they gain sensitivity to stretch stimuli, as well as additional chemosensitivity (notably becoming sensitive adenosine triphosphate (ATP) analogues that activate purinergic receptors). These sensors represent the classic RARs known to be involved in the Hering-Breuer deflation reflex. In experimental animals, intrapulmonary RAR activity is enhanced by pulmonary congestion, atelectasis, bronchoconstriction, and decreases in lung compliance, all of which can contribute to cough in patients. However, whether intrapulmonary RARs can directly initiate coughing is unclear. The other type of intrapulmonary mechanoreceptor ( slowly adapting stretch receptors [SARs] involved in the Hering Breuer inflation reflex) may inhibit cough through central mechanisms.

C-fiber sensors are also found in the tracheal, bronchial, and alveolar walls. They are activated by much the same range of stimuli as are those in the larynx, but their evoked responses are not homogeneous and, at least for those in the alveolar walls, do not include cough. In some species the C-fiber sensors may release tachykinins, such as substance P, by an axon reflex, which in turn causes neurogenic inflammation. This may explain the effect of tachykinin antagonists in inhibiting cough, although axon reflexes do not appear to be prevalent in human airways. C fibers are also responsible for generating the urge-to-cough, which may trigger behavioral coughing in an attempt to relieve the urge. Indeed, there is no convincing evidence that C-fiber receptors can be a primary sensory input for reflexive cough. Airway C-fiber sensors have been differentiated into two groups and, in the guinea pig, a new group of Αδ-nociceptors has been identified. Jugular ganglia C fibers can initiate coughing, whereas nodose ganglia C fibers do not and may even be inhibitory to cough. The role of Aδ-nociceptors in cough has not been defined. Thus, the fact that multiple sensory nerve subtypes can elicit or modify cough suggests that the total pattern of cough may be due to the interaction of several reflexes. This view, together with the variety of physiologic patterns of sensitivities and responses of mechanosensors and nociceptors in various parts of the respiratory tract, could explain the great divergence of cough patterns in different conditions and in different patients. The effectiveness of antitussive drugs that act at peripheral sites (e.g., by the inhalation of anesthetic aerosols) may depend on their relative actions on the different groups of receptors that affect cough or on their central connections.
Membrane Receptors/Channels on Sensory Endings for Cough
The past decade has seen extensive studies on the membrane/channel receptors in sensory nerves involved in coughing. The details are complex, so they are summarized only briefly; however, the results are of considerable importance in not only indicating how coughing may be induced but also by pointing to possible future targets in antitussive therapy.
Mechanically-activated cough sensors are stimulated by touch but not stretch and are thought to express mechanically gated membrane channels that are unique to this class of airway sensory neuron. These channels have yet to be identified. In addition, they have other channels that can be activated by acid and belong to the acid-sensing ion channel (ASIC) family and the NaV1.7 subtype of voltage gated sodium channels, characterized by their sensitivity to the neurotoxin, tetrodotoxin. However, the mechanosensor membranes normally lack several transient receptor potential (TRP) channels, which are found in C- and Aδ-nociceptors, namely the temperature-sensitive transient receptor potential vanilloid-1 (TRPV1) and transient receptor potential cation channel, subfamily A, member 1 (TRPA1) receptors, although the expression of these TRP channels on mechanosensors may be induced during inflammation. TRPV1 receptors are directly activated by capsaicin, much used as a tussive agent, and are sensitized or indirectly activated by heat, protons, bradykinin, arachidonic acid derivatives, ATP, and phosphokinase C. TRPA1 is coexpressed with TRPV1 on many vagal C fibers in the airways and is activated by allyl isothiocyanate (mustard oil), cinnamaldehyde (from cinnamon), and acrolein (from cigarette smoke). TRPA1 has been shown to mediate a myriad of sensory nerve dependent processes and may interact functionally with TRPV1. C fibers also express tetrodotoxin-insensitive voltage-gated sodium channels.
Thus, the way in which a large variety of tussive stimulants activate the sensory nerves responsible for cough and the urge-to-cough is beginning to be clarified, and it is now recognized that stimuli must produce the correct pattern of action potential firing in order to encode for coughing. Of note, the membrane receptors on cough sensors have been studied mainly in experimental animals, and their relevance to human cough is still unclear.
Central Nervous System Control
A (possible) circuit diagram for the interaction of cough inputs and outputs and the respiratory rhythm generator in the brain stem has been proposed. Reflex coughing is integrated in the medulla oblongata, where the afferent fibers for coughing first relay in or near the nucleus of the tractus solitarius; the motor outputs are in the nucleus retroambigualis, sending motoneurons to the respiratory muscles, and in the nucleus ambiguous, sending motoneurons to the larynx and bronchial tree. In the cat, brain-stem pathways for the expiration reflex differ from those for a typical cough, and pathways for cough from the larynx differ from those from the tracheobronchial tree. These observations, if true for humans, may point to different antitussive agents appropriate to the two sites.
Afferent inputs to the brain stem are relayed to higher brain regions where inputs are integrated in pontine, subcortical, and cortical nuclei. However, these ascending pathways may be different for sensors innervating the upper versus lower airways. Studies with functional magnetic resonance imaging of the human brain during induced cough are delineating those areas involved and have shown them to be important for the different sensory discriminative and motor aspects of noxious airway stimulation ( Fig. 30-5 ). For example, the anterior insula cortex is activated in a stimulus-dependent manner, suggesting that it plays a role in monitoring the amount of sensory input arising from the airways. The primary sensory cortex, on the other hand, is activated in a perception-dependent manner, suggesting that it is intimately involved in assimilating sensory inputs and coding for the urge-to-cough intensity. The posterior parietal and prefrontal cortices provide spatial awareness (i.e., where is the stimulus located), and parts of the limbic system (e.g., the orbitofrontal cortex) help shape emotive responses to the irritation. Cough can also be initiated voluntarily, a process that originates in motor and premotor cortical brain regions. These voluntary descending pathways can probably bypass brain-stem integrative centers (see Fig. 30-4 ) because some patients with brain-stem damage lack a spontaneous cough reflex but can consciously induce coughing to clear the airways. The motor cortex and inferior frontal gyrus can also generate cough suppression, which inhibits brain stem–mediated cough via poorly defined pathways. Greater understanding of the central control of coughing is desirable because most antitussive drugs act centrally, and we know little about how they do this. However, research has shown that central nervous membrane receptors for cough include those that respond to serotonin, gamma-aminobutyric acid, N-methyl- d -aspartate (NMDA), neurokinins, and dopamine, results that could have considerable therapeutic implications.

The central nervous pathways for cough show interactions and plasticity, as do the peripheral mechanisms already described. For example, afferent fibers from mechanosensors and C-fiber receptors converge in the nucleus of the solitary tract. Neurokinins released from the latter potentiate the activity of the former, especially cough and reflex bronchoconstriction, and this potentiation is enhanced by continued C-fiber activity. Ongoing activity of peripheral afferents may therefore lead to central plasticity of cough circuits, thereby reducing the need for peripheral inputs to elicit coughing. This is likely important for chronic cough in respiratory disease but may also be seen in some cases of gastroesophageal reflux disease (GERD), where activity in esophageal afferents, which do not normally cause cough, may activate the cough reflex when sensitization takes place.
Motor Outputs
Although glottic closure is usually regarded as an essential and definitive component of cough, in both human and experimental animals, the closure may be incomplete or even absent, and this does not seem to impair the effectiveness of the cough in terms of airway clearance. In addition, the glottal closure reflex has a lower threshold to irritants than does the expiration and cough reflexes and may therefore depend on different pathways.
Coughing is associated with respiratory actions other than those of the respiratory skeletal muscles. There is usually bronchoconstriction, although this may be masked or reversed by the dramatic changes in lung volume. The afferent mechanisms for reflex cough and reflex bronchoconstriction may be different, even though the initial stimulus is the same. Thus, extrapulmonary mechanosensors and jugular ganglia–derived C fibers may evoke cough, whereas the bronchoconstriction is mainly by intrapulmonary RARs and nodose ganglia–derived C-fiber sensors. Bronchoconstriction would increase linear velocity of airflow and lessen the inflow of irritant material to deeper parts of the airways.
The afferent sensors for cough also cause reflex secretion of mucus from airway submucosal glands. Mucus entraps inhaled particles and irritant chemicals, and the material is thus cleared from the airways by mucociliary transport and by the cough itself. Mucus could also act as a physicochemical barrier between the luminal irritants and the airway wall. However, an increase in mucus secretion in conditions associated with cough has never been accurately measured, perhaps because of lack of appropriate methods.
Mechanics of Coughing
The inspiratory phase of cough consists of a deep inspiration through a widely opened glottis. The inhaled volume varies greatly, from a low to a nearly complete vital capacity. The inspiration may draw material into the lungs; however, the large lung volume provides a better mechanical efficiency for the expiratory muscles of cough because they are stretched, their stretch reflex is activated, and there is a stronger elastic recoil of the lung to aid expiration. Furthermore, the deep inspiration opens the airways in preparation for their clearance during the expiratory phase.
In the compressive phase of cough, which lasts about 200 ms, the glottis closes while the expiratory muscles contract, and the intrapleural and intra-alveolar pressures rise rapidly to a range of values that can vary from 40 to 400 cm H 2 O, which can be used as an index of the intensity of the cough. The expulsive phase follows when the glottis opens. The expiratory flow rate depends on both air leaving the central airways during dynamic collapse as a result of the high intrathoracic pressure and the effect of high alveolar pressure, increased during the compressive phase and maintained at a high level by the contraction of the expiratory muscles. The expulsive phase of coughing may be long-lasting, with a large expiratory tidal volume, or it may be interrupted by glottic closures into a series of short expiratory efforts, each having a compressive and an expulsive phase. What determines the pattern of coughing has not been established but may depend on the anatomic site of origin of the cough, on the different nerve types of receptor activated, and on the strength of their activation.
Maximum expiratory flow is effort independent because it is limited by dynamic compression of the airways. This compression starts immediately downstream from the “equal pressure point” at which intraluminal and extraluminal pressures around the bronchial wall are equal so that transbronchial pressure is zero. The effectiveness of cough depends on peak airflow and will therefore be greater with a larger elastic recoil of the lung and a greater stiffness of the central airways. Dynamic compression of the airways downstream from the equal pressure point increases velocity, kinetic energy, and turbulence of the air passing through the proximal airways. Thus, the clearing capacity of the cough is improved. If cough consists of a series of expiratory efforts, with lung volume decreasing with each effort, dynamic compression is predicted to move into the more peripheral bronchi, which will be progressively cleared of intraluminal material. However, at present this description is largely theoretical and needs to be established experimentally.
Whereas mucociliary transport is the major method of clearing the airway lumen in healthy subjects, cough is an important reserve mechanism, especially in patients with lung disease. In many lung diseases, mucociliary clearance is impeded and cough is necessary to remove the increased amount of secretions and debris. Healthy subjects have twice the mucociliary clearance rate of that in patients with chronic bronchitis, but when cough is permitted or encouraged, the patients increase their clearance by 20%, whereas healthy subjects increase their clearance by only 2.5%. As would be expected, all studies point to the fact that cough is effective in causing clearance if there is hypersecretion of mucus; by definition, a dry cough is an unproductive one.
Neural Mechanisms of Cough Hypersensitivity
In disease, the sensory receptors for cough can show an exaggerated response to stimuli that would normally be harmless or mildly irritating. This increase in sensitivity of mechanosensors and C-fiber receptors can be caused by stimuli including allergen challenge, viral infections, ozone, cigarette smoke, and a variety of inflammatory mediators. Mechanosensors can also be sensitized by mucus in the airways, smooth muscle contraction, and mucosal edema. Increases in sensitivity have been related to structural changes in the nervous receptors, in particular in their intracellular mediators, and in the nerve cell bodies in the jugular and nodose ganglia and in the afferent pathways entering the brain stem. These effects lead to central sensitization, a neural mechanism in the central nervous system (CNS) that amplifies incoming sensory inputs and produces hyperreflexic states.
The alterations in the structure and function of vagal sensory neurons and central processing pathways seen in disease models of hypertussia (increased cough sensitivity to known stimuli) reflect cough neuropathy ( Fig. 30-6 ). The concept of a vagal sensory neuropathy producing airway hypersensitivity is comparable with the well-known role of peripheral sensory neuropathy leading to chronic pain syndromes. In patients with neuropathic pain, damage or disease of the peripheral sensory nervous system or its central projection pathways underpins pain hypersensitivity, characterized by the clinical pain symptoms of hyperalgesia (an elevated sensitivity to painful stimuli) and allodynia (pain in response to nonpainful stimuli). Neuropathic pain may act in tandem with inflammatory pain mechanisms in which altered nerve fiber activity is associated with both local tissue inflammation, as well as damage or disease of the nervous system. Indeed, a host of inflammatory mediators released from resident and infiltrating cells can sensitize or damage the airway nervous system to alter its normal functions. Although the nerve damage in neuropathic pain may be easy to recognize (e.g., the crushing or severing of peripheral nerves associated with major trauma), neuropathies can manifest as a result of less apparent insults including viral infection, metabolic diseases (e.g., diabetes), and other causes of neuroinflammation.

Approach to the Patient with Cough
As emphasized in all national guidelines, the approach to the management of a patient with cough is first to identify the cause(s) of the cough and then to treat the cause(s). Cough may be indicative of trivial or serious airway or lung diseases and also of extrapulmonary processes. Often, the cause may not be found, the treatment of the putative cause may not suppress or improve the cough, or the cause may have no effective treatment. In those cases, therapy that suppresses cough by inhibiting the cough pathway without treating the cause (“symptomatic,” “nonspecific,” or “indirect” antitussives) is necessary.
The differential diagnosis of cough is extensive and includes infections, inflammatory and neoplastic conditions, and many pulmonary, as well as extrapulmonary, conditions ( Table 30-1 ). The protocol for investigating a chronic cough—defined as a cough that has persisted for more than 3 weeks—takes into account several factors pertaining to the pathophysiology of cough and to the most common causes of cough. Persistent cough may be due to the presence of excessive secretions, airway damage and infection, or the establishment of a hypersensitive cough reflex. A protocol based on systematic evaluation using history, examination, and laboratory investigations focusing on the anatomic sites of cough receptors that constitute the afferent limb of the cough reflex is a most widely advocated approach to diagnosis and treatment.
ACUTE INFECTIONS |
Tracheobronchitis |
Bronchopneumonia |
Viral pneumonia |
Acute-on-chronic bronchitis |
Pertussis |
CHRONIC INFECTIONS |
Bronchiectasis |
Tuberculosis |
Cystic fibrosis |
AIRWAY DISEASES |
Asthma |
Eosinophilic bronchitis |
Cough variant asthma |
Chronic bronchitis |
COPD |
Chronic postnasal drip |
PARENCHYMAL DISEASES |
Interstitial pulmonary fibrosis |
Emphysema |
Sarcoidosis |
TUMORS |
Bronchogenic carcinoma |
Alveolar cell carcinoma |
Benign airway tumors |
Mediastinal tumors |
ASPIRATED FOREIGN BODIES |
MIDDLE EAR PATHOLOGY |
CARDIOVASCULAR DISEASES |
Left ventricular failure |
Pulmonary infarction |
Aortic aneurysm |
OTHER DISEASES |
Gastroesophageal reflux disease |
Laryngopharyngeal reflux |
Recurrent microaspiration |
Endobronchial sutures |
Obstructive sleep apnea |
Laryngeal dysfunction |
DRUGS |
Angiotensin-converting enzyme inhibitor medications |
The foremost consideration for the clinician at the first visit is to (1) determine the severity, (2) assess the cause(s) of the cough, and (3) plan investigations and treatment. Various indicators in the history and examination of the patient may provide clues to the diagnosis, although these may not be entirely reliable or specific and may be absent in many cases.
An acute cough due to an upper respiratory virus infection generally lasts for no more than 3 weeks, although some postviral coughs may persist for many weeks or months. Thus, a chronic cough is considered to be one that persists longer than 3 weeks. The one caveat is that many patients with an “idiopathic” cough often state that their cough originated during an upper respiratory tract infection. Nonetheless, a cough that has lasted for more than 2 to 3 months is unlikely to be due to an upper respiratory tract infection, and other associated causes must be investigated.
Cough with sputum production usually points toward conditions such as chronic bronchitis and bronchiectasis or other causes of bronchorrhea. The diagnostic value of knowing that the cough is productive is probably limited because similar causes are often found for both productive and dry cough. In addition, the volume of sputum produced is difficult to estimate accurately (usually depending on sputum cups and block of salivary contamination), and coughing itself leads to sputum production. The concept of a dry versus a productive cough as delineating a cough secondary to an increased cough reflex for the former and a cough secondary to excessive mucus production for the latter is not entirely correct. An enhanced cough reflex may be present in both productive and nonproductive cough. Features that are associated with an increased cough reflex include cough triggered by taking a deep breath, laughing, inhaling cold air, and prolonged talking. Therefore, the diagnostic approach remains similar whether or not the cough is productive.
The characteristics of the cough, however, may sometimes help direct the diagnostic evaluation. Throat clearing may be associated with a postnasal drip, a predominantly nocturnal cough may be attributed to asthma, or coughing after meals may be related to coexistent GERD. However, the predictive value of these characteristics is low. A cough with a “honking” or “barking” quality, particularly in a child, has been associated with a psychogenic or habit cough.
Many cigarette smokers have a chronic cough but rarely seek medical advice regarding their cough because they expect that the irritant effect of cigarette smoke is the cause of their cough. However, chronic smokers may actually have a reduction in their cough reflex sensitivity, perhaps due to desensitization or damage of the epithelial nerve endings. A change in the pattern or characteristics of their cough, such as an increase in intensity (usually after an upper respiratory tract infection), or accompanying hemoptysis may force a smoker to seek medical attention. A chest radiograph is mandatory in this situation.
Measuring Cough
Assessment of cough frequency and severity rests mainly on the history. In very severe cough, patients may experience complications such as vomiting, rib fractures, tiredness, incontinence, and syncope ( Table 30-2 ), and their presence indicates that the chronicity and intensity of the cough are severe. The effect of cough on the patient’s lifestyle and psychological well-being may also provide an idea of the severity of the cough. Questionnaires that are specifically devised to measure these effects have been developed and validated. Direct measurement of the frequency and severity of cough has also been developed. In many published studies, the effectiveness of particular interventions on cough has been determined qualitatively in the clinic, but the use of more quantitative tools is now gradually being introduced (see later).
RESPIRATORY |
Pneumothorax |
Subcutaneous emphysema |
Pneumomediastinum |
Pneumoperitoneum |
Laryngeal damage |
CARDIOVASCULAR |
Cardiac dysrhythmias |
Loss of consciousness |
Subconjunctival hemorrhage |
CENTRAL NERVOUS SYSTEM |
Syncope |
Headaches |
Cerebral air embolism |
MUSCULOSKELETAL |
Intercostal muscle pain |
Rupture of rectus abdominis muscle |
Increase in serum creatine phosphokinase |
Cervical disc prolapse |
GASTROINTESTINAL |
Esophageal perforation |
OTHER |
Social embarrassment |
Depression |
Urinary incontinence |
Disruption of surgical wounds |
Petechiae |
Purpura |
Cough can be measured in several ways: (1) cough severity can be judged by the patient recording her or his perception on a visual analogue scale ranging from mild to severe or on a nominal line from 0 to 10; (2) the cough reflex can be measured by counting the cough responses to inhalation of tussive agents such as capsaicin, the pungent extract of peppers, or citric acid– or low-chloride-content solutions; (3) cough frequency and intensity can be measured by quantitative ambulatory recording; and (4) cough-specific, health-related quality of life questionnaires can provide a quantitative measure of the impact of chronic cough on the patient. There is a correlation between the various measures of cough as, for example, between objectively measured cough frequency and subjective scoring systems measured by the cough visual analogue scale or the Leicester Cough questionnaire, although this correlation remains weak. This means that subjective scoring systems may be responsive to not only cough frequency but also other factors related to the cough. Therefore, a combination of both subjective and objective measurements should ideally be used. All these features are of concern to the patient.
Measurement of the Cough Reflex
Persistent cough may result from an increase in the sensitivity of the cough sensors. Most patients with a persistent cough, due to a range of causes, have an enhanced cough reflex to inhalation of an aerosol of capsaicin or of citric acid when compared with healthy noncoughing subjects. Successful treatment of the primary condition underlying the chronic cough (specific or disease-specific treatment) sometimes leads to a normalization of the cough reflex. The degree of the cough responsiveness to inhaled capsaicin may contribute to the severity of the cough. Of relevance to the evaluation and treatment strategies for persistent dry cough is the fact that the cough response can be augmented by various mediators of inflammation such as the prostaglandins PGE 2 and PGF 2α and bradykinin through a process of sensitization. Measurement of the cough reflex response to such agents may be useful in confirming the presence of a persistent cough or in assessing the response to treatment. At the moment, only centers with an interest in cough use it for clinical and research purposes.
Measurement of Cough Frequency and Intensity
Advances in computer technology and digital sound recording have led to the development of ambulatory systems for audio or audiovisual recording of cough, with the ultimate objective of automatically obtaining cough counts and possibly intensity in a real-life setting. Several devices have been described. The number of cough sounds and the cough seconds, a measurement of the time of coughing, have been shown to correlate with scores on a Leicester Cough Questionnaire (LCQ) and on a cough visual analogue scale.
Although the methods are valuable in counting the number of expiratory efforts associated with cough, they do not allow assessment of cough intensity (because this need not correlate with intensity of expiratory sound). They can be used to differentiate between individual coughs and those associated with a cough epoch, although the definition of the distinction is arbitrary. Both the frequency and intensity of cough efforts are features of importance to the patient. Current methods do not allow a distinction between a “true” cough and an expiration reflex, a feature that might seem trivial to a patient but should be important to the investigator because it might provide insight into the location of the cough trigger.
Quality of Life Questionnaires
Quality of life questionnaires specific for the evaluation of the impact of chronic cough provide subjective measurements that are likely to reflect the severity of cough from the viewpoint of the patient. Such measurements integrate both the impact of frequency and the intensity of cough. The LCQ uses a seven-point Likert response scale for 19 items from three domains (physical, psychological, and social) and is shown to be repeatable and sensitive in patients with chronic cough. This is increasingly becoming a useful clinical tool.
Diagnosis and Investigations of Chronic Cough
The history and examination will sometimes indicate the likely associated diagnosis or diagnoses, and the timing of various investigations may vary according to presentation ( Table 30-3 ). Initial investigations may be limited to a chest radiograph, particularly in a cigarette smoker. Abnormalities have been reported in 10% to 30% of chest radiographs of cigarette smokers, although the yield of tumors is likely to be lower. Further investigations (e.g., computed tomography [CT] or fiberoptic bronchoscopy) may be pursued despite a “normal” chest radiograph.
|
Patients on angiotensin-converting enzyme (ACE) inhibitor therapy should discontinue such therapy, with replacement by other appropriate treatments. Patients who provide a good history of an upper respiratory tract infection may be observed for 3 to 4 weeks before further investigation or therapeutic trial, although institution of an anti-inflammatory therapy such as inhaled corticosteroids can be useful in controlling this type of cough.
After these initial steps, investigation of the major causes of cough should be considered. Postnasal drip (“nasal catarrh” or “upper airway cough syndrome”), asthma, and GERD are the three most common conditions associated with a chronic cough, and a diagnostic approach to exclude these conditions is sensible. Postnasal drip, secondary to rhinosinusitis, is an often overlooked condition. If there is a history of postnasal drip or rhinosinusitis, examination of the nose and sinuses with a computed axial tomogram of the sinuses may be indicated. Treatment consists of corticosteroid nasal drops together with an antihistamine, with the possibility of adding antibiotic therapy and a short period of treatment with a nasal decongestant. An asthma diagnosis is supported by the presence of diurnal variation in peak flow measurements, bronchial hyperresponsiveness to histamine or methacholine challenge, and the presence of eosinophils in sputum. Under the umbrella of “asthma” would also be included cough-variant asthma and eosinophilic bronchitis. However, a therapeutic trial with inhaled corticosteroids may be the best initial approach, particularly when the history and examination provide supportive clues. It is important that effective doses of medication over a sufficient period of time are given. Often, a longer-than-usual period of treatment is necessary to control the cough, and GERD may be initially evaluated with ambulatory esophageal pH monitoring.
Often, more than one of these conditions may coexist and cough may respond only with concomitant treatment of these. For example, inhaled steroid therapy and gastric acid suppression with a proton pump inhibitor or H 2 -histamine blockers would be indicated for the coexistence of asthma and GERD, respectively.
Bearing in mind that there are a myriad of other less common causes of a chronic cough, investigations must proceed further if the common causes have been excluded. Lung function tests to include lung volumes and diffusing capacity, as well as a CT of the lungs, should be considered in case of bronchiolar or parenchymal disease or unsuspected bronchiectasis. Fiberoptic bronchoscopy should be considered and, apart from excluding small central tumors, provides mucosal biopsies for histologic diagnosis (e.g., to diagnose eosinophilic bronchitis).
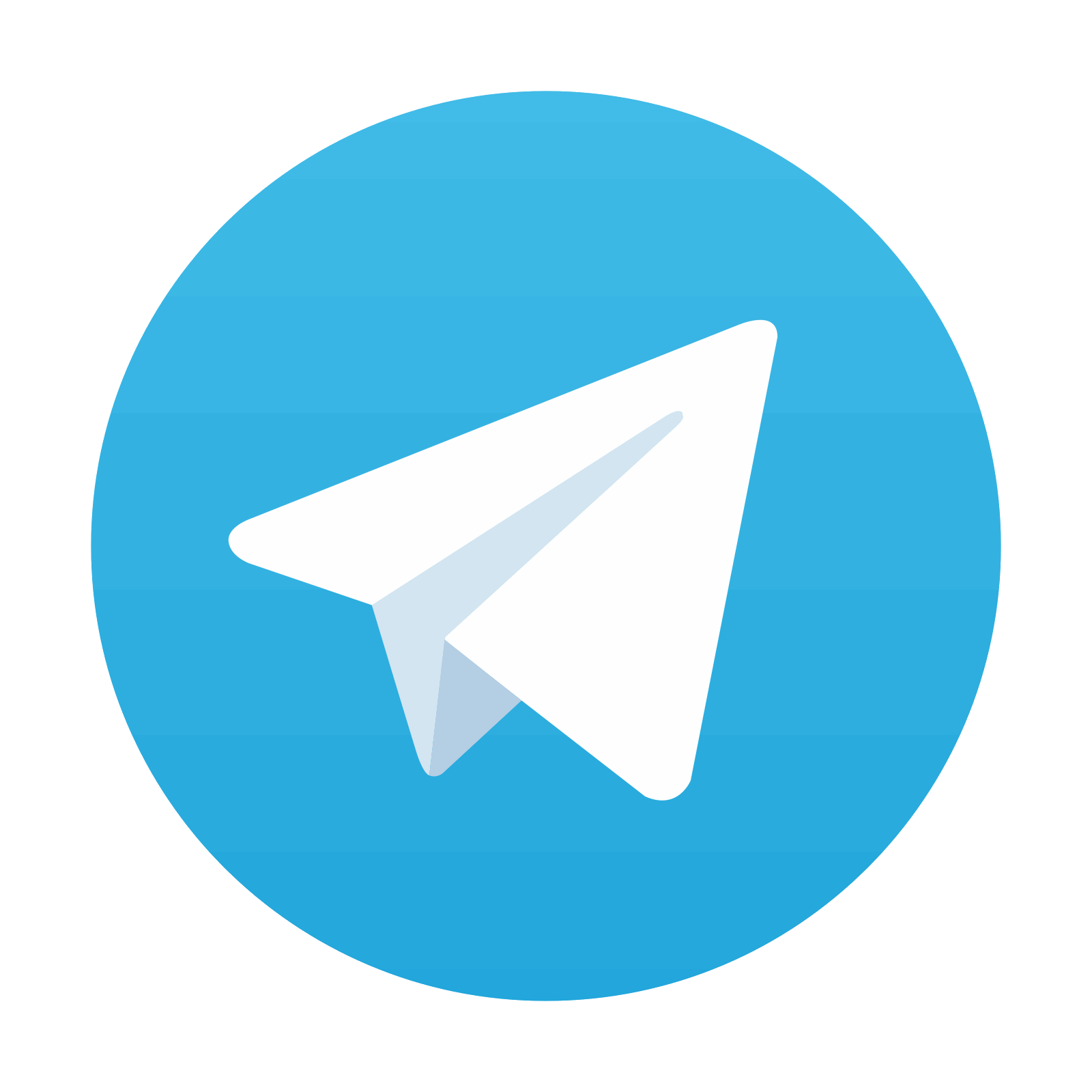
Stay updated, free articles. Join our Telegram channel

Full access? Get Clinical Tree
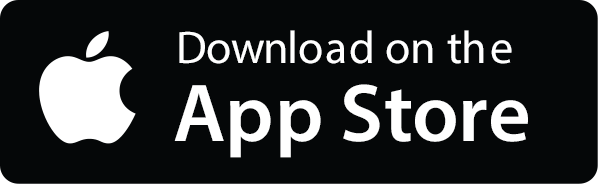
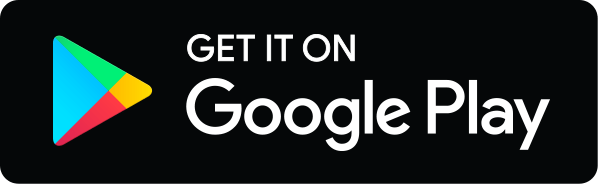