Introduction
Asthma is one of the oldest known diseases, but it has only been recognized as a major public health problem since the mid-1970s. The prevalence of asthma has increased dramatically, and asthma is now recognized as a major cause of disability, medical expense, and preventable death. Asthma has attracted the full spectrum of biomedical investigation, from studies of the prevalence of asthmatic symptoms in different populations to studies of the effects of substitution of single base pairs in genes in animal models of allergic sensitization of the airways. These studies continue to refine the scientific understanding of asthma and suggest new approaches to diagnosis and treatment. The scope and depth of these studies present significant challenges in reviewing the topic of asthma. This chapter combines the perspectives of the authors with a “snapshot” of the body of knowledge, which is expanding at an explosive pace.
Definition
Although asthma is a clearly recognized clinical entity, agreement on a precise definition has proved elusive. Asthma has been more often described than defined. The earliest feature described was the labored, rapid breathing typical of asthmatic attacks; the word “asthma” is derived from the ancient Greek word for “panting.” As knowledge about asthma has grown, the features described as characteristic of asthma have expanded. Measurement of maximal expiratory flow led to recognition of reversible airflow obstruction as a characteristic feature; measurement of changes in airflow after inhalation of chemical or physical irritants led to the definition of bronchial hyperresponsiveness. In addition, studies of bronchial biopsies added a description of characteristic pathologic features. This evolution in the understanding of asthma is summarized in the definition offered in the National Heart, Lung, and Blood Institute’s (NHLBI’s) 2007 Update on Asthma Pathophysiology and Treatment Guidelines :
“Asthma is a chronic inflammatory disorder of the airways in which many cells and cellular elements play a role, including mast cells, eosinophils, T lymphocytes, macrophages, neutrophils, and epithelial cells. In susceptible individuals, inflammation causes recurrent episodes of wheezing, breathlessness, chest tightness, and coughing, particularly at night or in the early morning. These episodes are usually associated with widespread but variable airflow obstruction that is often reversible either spontaneously or with treatment. The inflammation also causes an associated increase in bronchial hyperresponsiveness to a variety of stimuli.”
A feature found even more consistently than eosinophilia in bronchial biopsies from patients with asthma is thickening of the lamina reticularis immediately underneath the subepithelial basement membrane. This thickening is considered a hallmark of airway “remodeling”; however, this feature has not yet been incorporated into consensus definitions of asthma.
The consensus conference “definition” of asthma serves well as a description of the major features of asthma but does not hold up as a precise definition. No feature is unique to asthma, and no feature is universal in patients with the condition. For example, all tests of airway caliber may be normal between attacks, even in patients whose attacks are sudden and severe. Bronchial responsiveness may be normal over most of the year in patients with seasonal asthma, and bronchial hyperresponsiveness is often found in people with allergic rhinitis but without asthma. Even the association between eosinophilic bronchial inflammation and asthma is inconstant. Some patients with recurrent episodes of wheezing and dyspnea associated with reversible airflow obstruction and bronchial hyperresponsiveness have no evidence of eosinophilic inflammation on bronchial biopsies. Other patients have eosinophilic inflammation of the bronchial mucosa and chronic cough responsive to treatment with an inhaled corticosteroid but have neither airflow obstruction nor bronchial hyperresponsiveness. Finally, some patients with severe asthma have a predominance of neutrophils, rather than eosinophils, in their bronchial mucosa.
The lack of firm, universally agreed-upon criteria for defining asthma complicates epidemiologic studies of the prevalence of asthma in different populations and of changes in prevalence in the same population over time, but agreement on “working definitions” of asthma has led to many informative studies.
The recognition of asthma as a complex, multifactorial disorder has led to a greater focus on the individual and the varied disturbances in function that contribute to a more or less common clinical expression. Recent advances in science have not necessarily led to a more precise definition of asthma; rather, these advances have made the need for agreement on a definition seem less urgent.
Clinical Diagnosis
The diagnosis of asthma is often made in the ambulatory care setting and is based on a careful personal history, physical examination, and lung function testing ( Fig. 42-1 ). A number of different diseases can mimic asthma and cause some or all of the symptoms of asthma. These include vocal cord dysfunction, chronic obstructive pulmonary disease (COPD), cystic fibrosis, bronchiectasis, congestive heart failure, sleep apnea, pneumonia, sarcoidosis, and psychosomatic diseases and need to be considered when making the diagnosis of asthma.

Personal History
Asthma can arise at any age but usually presents for the first time in childhood. Risk factors for asthma include a family history of asthma and a personal or family history of atopy (e.g., atopic dermatitis, seasonal allergic rhinitis, conjunctivitis). In addition, asthma can develop in individuals sensitive to aspirin or exposed to chemical toxins or environmental allergens.
A comprehensive history is therefore critical in the assessment of the asthmatic patient. Most patients with asthma complain of intermittent symptoms of cough, shortness of breath, and/or wheezing, often described as a high-pitched musical whistling. They often experience periods of symptom-free normal breathing interrupted by periods of difficulty breathing. Asthma symptoms can last for a few minutes or days. Cough is a frequent complaint in asthma; the cough may or may not be accompanied by sputum production, may worsen at night or with activity, and/or may develop after exposure to allergens. Shortness of breath and wheezing, usually on exhalation, often manifest during an exacerbation and can be triggered by infection, cold air, exercise, exposure to chemical fumes or other airborne irritants, pet dander, molds, house dust mites, or other allergens. In addition, some patients describe bandlike chest tightness, or chest heaviness, during an exacerbation. Complaints of sharp stabbing or knifelike pain would be unusual in asthma and should direct the clinician to alternative diagnoses.
Work History
Symptoms that improve on weekends and vacations and worsen at work should raise the suspicion of occupational and/or work-exacerbated asthma. Occupational asthma is new-onset asthma due to causes and conditions attributable to a particular occupational environment, whereas work-exacerbated asthma is defined as exacerbation of known asthma by the work environment. Indeed, up to 15% of all adult asthma can be attributed to occupation. The work exposure history should focus on identifying agents that were present at the time of asthma diagnosis or when asthma symptoms worsened. There are two major types of occupational asthma: sensitizer-induced asthma and irritant-induced asthma. Sensitizers are often further classified into low-molecular-weight (small chemicals) and high-molecular-weight (usually proteins) agents. Reactive airway dysfunction syndrome (RADS), the best-defined form of irritant-induced asthma, results from a single high-dose exposure to irritants. (For a more detailed discussion of RADS and occupational asthma, see Chapter 72 .)
Physical Examination
The physical examination should focus on the head and neck, chest, and skin. The physical examination is often normal; however, findings such as wheezing during normal breathing and/or a prolonged expiratory phase may suggest asthma. A maneuver to enhance wheezing, forced exhalation, is not specific for asthma, but suggests airway obstruction or collapse. In addition to breathing abnormalities, subjects with asthma often have concomitant signs of associated allergic conditions and evidence of upper respiratory tract inflammation and obstruction with inflamed nasal passages and/or nasal polyps or large tonsils. The findings of eczema, hives, or atopic dermatitis on skin examination are supportive of an asthma diagnosis.
Physiology of Airflow Limitation
As understanding of the pathogenesis of asthma improves and strategies for treatment evolve, it is important to recognize that the link between the pathophysiology and the treatment of asthma is functional, involving variable limitation of air flow. These two cardinal manifestations of asthma, variability of symptoms in response to environmental factors and limitation of air flow, are crucial to making the diagnosis of asthma and distinguishing asthma from other obstructive lung diseases.
During asthma exacerbations, diffuse narrowing of the airways results in profound physiologic consequences. This narrowing has been thought to take place disproportionately in the small bronchi, although more recent studies suggest a prominent role for large and medium airways. As a result, lung function tests are abnormal, with an increase in airway resistance and a decline in maximal expiratory flow. Airway narrowing also prevents the lungs from completely emptying (“air trapping”) due to resistance to expiratory flow and bronchial closure at higher than normal lung volumes. The breath-to-breath variability of asthmatic obstruction and air trapping led to the concept of dynamic hyperinflation. As a result of dynamic hyperinflation, asthmatic patients breathe at higher total lung volumes, detectable as increased residual volume. Despite elevated total lung volumes, asthmatics typically have reduced tidal ventilation. Decreasing forced vital capacity (FVC) suggests worsening air trapping, whereas worsening of the forced expiratory volume in 1 second (FEV 1 )/FVC ratio indicates increasing airway narrowing.
At high lung volumes, flow limitation due to bronchial narrowing is offset by increased circumferential traction on the bronchial airways due to “tethering” of airways to inflated alveoli. This tethering effect may be less effective in asthma because the altered mechanical properties of the extracellular matrix in asthmatic airways stiffen the airways and reduce the mechanical forces opposing airway smooth muscle (ASM) contraction. The net effect is that the work of breathing increases significantly, due in part to decreased lung and chest wall compliance at higher thoracic volumes and in part to the greater effort required to overcome the resistance of narrowed airways. The diaphragm and intercostal muscles are overloaded due to thoracic hyperinflation and mechanically disadvantaged due to suboptimal positioning on their length-tension curves. As a result, accessory muscles of respiration, including the abdominal muscles and the sternocleidomastoids, are required. A large proportion of the subjective dyspnea associated with asthma exacerbations has been attributed to respiratory muscle fatigue.
The airway obstruction and closure in asthma is nonuniform, with significant regional variability that may not be completely reflected by decreases in peak flow. Although pulmonary blood flow is reduced in areas of alveolar hypoventilation, the magnitude of this response is insufficient to offset more than moderate or severe airflow obstruction. Ventilation-perfusion mismatching leads to a widened alveolar-arterial oxygen difference that tracks with increasing asthma severity; the arterial oxygen tension in acute severe asthma typically falls below 70 mm Hg. Arterial carbon dioxide tension initially falls as alveolar ventilation increases because carbon dioxide elimination is less impaired by ventilation-perfusion mismatching than is oxygen uptake. As respiratory muscles fatigue, carbon dioxide tension increases, so a normal or elevated P co 2 during an asthma exacerbation suggests impending respiratory failure. Worsening of airflow obstruction or any factor that decreases respiratory drive (such as sedation) can drop alveolar ventilation precipitously; the resultant rise in P co 2 further inhibits respiratory drive and muscle performance and hastens respiratory failure.
Pulmonary Function Testing (see Chapter 25 )
Even between asthma exacerbations, pulmonary function testing shows characteristic changes reflecting the reduced flow and air trapping of dynamic hyperinflation. Decreased expiratory flow can be easily and reproducibly detected with a peak flowmeter in ambulatory patients, and peak expiratory flow (PEF) measurement is an accepted method to correlate physiologic function with the clinical severity of asthma. However, peak flow measurements are not standardized and cannot be correlated with other measures of lung function. PEF as a percentage of the predicted value is 10% higher than FEV 1 on average, with a great deal of variability between measurements. PEF measurements tend to underestimate less severe obstruction and overestimate more severe obstruction. Currently, the recommended use of PEF measurement is for the daily monitoring of ambulatory patients with difficult-to-manage asthma. In this situation, the values should be compared with an individual patient’s baseline measurement obtained when asymptomatic and well controlled.
Spirometry
The best and most standardized test of airflow obstruction is the FEV 1 . The FEV 1 has the advantage of being an objective, non-patient-reported measurement of lung function. Improvement in FEV 1 of more than 12% and 200 mL after bronchodilator treatment indicates reversible airflow obstruction and is suggestive, but not diagnostic, of asthma. Accurate measurement requires stopping long-acting β 2 -agonists (LABAs) for at least 12 hours and short-acting bronchodilators for at least 6 hours. Because the FEV 1 may be normal or near-normal between asthma flares, it is a poor marker of response to bronchodilators in mild asthma. The absolute value of the FEV 1 is dependent on the FVC and also reflects the small airways. Interpretation of FEV 1 therefore requires simultaneous FVC measurement. In asthma, the relative reduction in FEV 1 is typically greater than the reduction in the FVC. As a result, the FEV 1 /FVC ratio in asthma is usually less than 70%. A characteristic flow-volume loop is created with scooping of the expiratory loop consistent with airflow limitation ( Fig. 42-2 ). However, in severe asthma, this ratio may actually increase as air trapping increases residual volume and reduces the FVC. This apparent reversible restriction due to air trapping has been reported in asthma, but lung volumes measured by body plethysmography are usually normal or elevated.

The performance of the forced expiratory maneuver requires inhalation to total lung capacity before exhalation. In normal patients, the resultant stretch of intrapulmonary airways causes reflex bronchodilation and a shift in the pressure-volume curve. This alteration in lung mechanics is generally attributed to a transient decrease in ASM tone. However, some asthmatics have the opposite response, developing bronchoconstriction during deep inspiration. The mechanism of this “spirometry-induced bronchoconstriction” is unknown, but some evidence suggests that it may be partly due to increased inflammation and remodeling of asthmatic airways.
An alternative measure of airflow obstruction is the midexpiratory flow, measured between 25% and 75% of the FVC ( forced expiratory flow [FEF] 25%-75% ). Measured at lower lung volumes than FEV 1 , reductions in the FEF 25%-75% may be more sensitive for identifying obstruction in small airways. Studies in patients at high risk for asthma based on atopic symptoms have shown that this index is valuable in predicting airway hyperresponsiveness. Similarly, in childhood asthmatics who have become asymptomatic, the FEF 25%-75% is a sensitive marker of residual physiologic abnormalities. The clinical utility of the FEF 25%-75% is limited by the lack of acceptable standardized values. High numbers of false-positive and false-negative results are seen when 80% of predicted FEF 25%-75% is used for classification.
The limitations of the FEF 25%-75% are due in part to large variation in the duration of FVC maneuvers. To address this issue, measurement of the forced expiratory volume over the first 6 seconds (FEV 6 ) has been proposed as an alternative to the FVC. The advantages of this maneuver over the FVC include ease of measurement, a more explicit end-of-test definition, and reduced risk of syncope. Data are available suggesting parameters that may be used for disease classification, although the sensitivity and specificity of the FEV 1 /FEV 6 in comparison with the FEV 1 /FVC ratio remain controversial.
Lung Volumes and Diffusing Capacity
As a result of dynamic hyperinflation and consequent air trapping, residual volume, functional residual capacity, and total lung capacity may be elevated in asthma. Gas dilution techniques measure only the communicating volume of gas that equilibrates with the tracer gas during the single or multiple breath maneuvers and may underestimate these volumes due to regional heterogeneity in the distribution of ventilation. For this reason, body plethysmography is often considered the method of choice for lung volume evaluation in severe asthma. However, because plethysmography measures the total volume of compressible gas within the thorax and abdomen, the inclusion of intra-abdominal gas may lead to overestimation of total lung capacity, particularly in severely obstructed asthmatics.
Single-breath diffusing capacity is a marker of carbon monoxide gas transfer in the lungs and is reduced in most chronic lung diseases because of altered alveolar capillary volume and/or maldistribution of inspired gas due to airflow obstruction. In asthma the diffusing capacity is normal or elevated if airflow obstruction is not severe, and this finding can be useful in distinguishing asthma from other obstructive lung diseases. Elevated diffusing capacity in asthma has been attributed to increased perfusion of well-ventilated upper lung zones and is associated with large lung volumes. The unexpected finding of an increase in diffusing capacity should raise the possibility of undiagnosed asthma, whereas decreased diffusing capacity in a suspected asthmatic is suggestive of an alternate diagnosis or coexisting condition.
Provocative Challenges and Airway Hyperresponsiveness
Airway Hyperresponsiveness
Airway hyperresponsiveness (AHR) is a characteristic feature of asthma. Hyperresponsiveness can develop in response to a number of nonspecific environmental irritants, pharmacologic agonists, and inflammatory mediators. In the past, the predominant view was that airway inflammation was the mechanism causing AHR. This concept has been criticized because a number of studies have shown dissociation between AHR and inflammation, highlighting the fact that inflammation alone is insufficient as a mechanism of disease. Evidence suggests that a number of inflammatory type 2 T helper (Th2) cytokines, CD4 cells, and biochemical mediators contribute to the development of AHR in asthma. Exposure to Th2 cytokines in asthmatic airways can increase the isometric constrictor response of ASM by increasing calcium release from intracellular stores. In addition to the airway inflammation, factors contributing to mechanical airway obstruction have also been implicated in the pathogenesis of AHR, including epithelial permeability, smooth muscle hypertrophy, mucus hypersecretion, and airway remodeling.
AHR to environmental stimuli is a hallmark of asthma. Patients suspected of having asthma despite normal lung function testing usually develop bronchoconstriction in response to a provocative stimulus. Direct stimulation, during which a substance known to induce bronchoconstriction via direct action on smooth muscle is inhaled into the airways, is the most widely used method of assessing bronchial hyperresponsiveness. Nebulized methacholine, delivered in doubling concentrations until FEV 1 falls by more than 20%, is the agent most commonly used for bronchoprovocation. The concentration that causes a 20% fall is labeled the PC 20 (provocative concentration resulting in 20% fall in the FEV 1 ) and can be used to quantify the degree of AHR. A PC 20 less than 16 mg/mL is consistent with mild AHR, less than 4 mg/mL is consistent with moderate AHR, and less than 1 mg/mL with severe AHR, with lower PC 20 levels generally corresponding to more severe asthma. Bronchial hyperresponsiveness also has been associated with increased risk of developing persistent asthma and airway remodeling.
Indirect stimuli, such as cold air, exercise, inhalation of hypertonic saline, mannitol, and adenosine monophosphate, may also induce bronchoconstriction. Rather than working directly on smooth muscle, indirect stimuli cause the release of inflammatory mediators from airway cells that then interact with ASM to induce bronchoconstriction. Although the dose response is more difficult to assess with indirect stimuli, the results have direct applicability to daily asthma symptoms. For example, direct challenge of athletes suspected of having exercise-induced bronchoconstriction is less likely to uncover bronchoconstriction than indirect challenge using a surrogate for physical activity. There also may be differences between direct and indirect stimuli in the evaluation of response to treatment, with indirect challenge providing a better idea about response to therapy.
Impulse Oscillometry
Impulse oscillometry (IOS) is a form of forced oscillometry testing, a noninvasive technique to characterize the airway through the superimposition of pressure fluctuations into the airway during normal tidal breathing. IOS delivers pressure oscillations via fixed square waves of 5- to 20-Hz frequency to measure airway resistance, the energy required to propagate a pressure wave through the airway, and reactance, the energy generated by the lungs’ recoil against that pressure wave. These measurements allow calculation of the force necessary to propagate this pressure wave through the respiratory system, known as impedance. The location of airway diseases can also be localized because lower-frequency oscillations tend to travel to the lung periphery and higher-frequency ones reach only the most proximal airways.
Measurements are recorded during normal passive tidal breathing and require little training. Not surprisingly, IOS is most well studied in younger children. Significant correlations between FEV 1 and FVC by spirometry and impedance and resistance by IOS have been described. Most impressively, IOS has outperformed spirometry in sensitivity and specificity for the diagnosis of asthma in young children when compared to a methacholine challenge and validated clinical questionnaire. Despite increasing acceptance in the pediatric population, experience in adult patients remains limited.
Imaging
Although traditionally, thoracic imaging has been used to rule out alternative pathologies, recent advances in imaging offer additional noninvasive techniques to support the diagnosis of asthma. The chest radiograph is most commonly normal in stable asthmatics, although nonspecific findings such as hyperinflation and bronchial wall thickening can be appreciated. The primary role of the plain chest radiograph remains to rule out asthma mimics in those with atypical symptoms and to evaluate difficult-to-control symptoms.
Most commonly, chest computed tomography (CT) is used to evaluate chest radiographic abnormalities or identify those mimics less apparent on traditional chest radiography, such as bronchiolitis, bronchiectasis, tracheobronchomalacia, endobronchial lesions, and vascular anomalies. Increasingly, high-resolution chest CT techniques offer a useful tool to assess large and small airway pathology directly and indirectly. Nonspecific findings such as bronchial wall thickening, bronchial wall dilation, airway dilation, bronchiectasis, mucoid impaction, and mosaic lung attenuation consistent with air trapping are described in studies of asthmatic subjects. In a recent qualitative analysis of high-resolution CT scans of 185 severe asthma patients, abnormalities were present in 80% of the subjects with 62% demonstrating bronchial wall thickening and 40% bronchiectasis. Quantitative assessments of the airway tree and lung parenchyma are now possible with advances in CT technology and a number of associated proposed algorithms. Several studies have demonstrated increased large airway wall thickness in asthmatic patients versus healthy controls regardless of disease severity. Others support a further relationship between asthma severity and measured airway thickness. Clinical application of these techniques, however, remains limited by mostly single-center experiences and a lack of proof-of concept studies. Despite the limited data, this is an emerging area in the diagnosis and phenotypic characterization of asthma.
Fraction of Exhaled Nitric Oxide
Nitric oxide (NO) and related compounds are generated by various resident and inflammatory airway cells and have a broad variety of functions as inflammatory mediators, vasodilators, bronchodilators, and neurotransmitters. In the asthmatic airway, NO exhibits a paradoxical response by enhancing airway dilation at low concentrations but propagating inflammatory responses at higher concentrations. Eosinophilic airway inflammation, as measured by tissue-based eosinophilia, bronchoalveolar lavage eosinophilia and bronchial epithelial major basic protein density, correlates with fraction of exhaled NO (FeNO) in some asthma patients.
Noninvasive measurements of FeNO have been standardized for clinical use and may serve as a complementary tool in asthma diagnosis. However, the FeNO in stable, mild asthma after use of inhaled corticosteroids is often in the same range as that in normal, nonasthmatic patients. FeNO falls in a dose-dependent manner with steroid use, making FeNO less useful as an ongoing biomarker in patients using steroid therapy. A recent trial showed that an FeNO greater than 45 parts/billion excludes well-controlled asthma with a negative predictive value of nearly 90%. High FeNO suggests a severe, steroid-responsive phenotype. A decline in FeNO greater than 40% between visits is a sign of improved control, and low levels of FeNO predict lower risk of acute exacerbation. Measuring the FeNO during a period of good control can provide a baseline for comparing trends over time when following symptoms and response to treatment. The American Thoracic Society (ATS) has published guidelines on the use of FeNO for the diagnosis and management of asthma; however, widespread use of this technology is still lagging. More recently, International ATS/ European Respiratory Society (ERS) guidelines warned against the use of FeNO to guide treatment in severe asthma, citing low-quality evidence.
Management of Asthma
Introduction
The inherent complexities in evaluating and treating asthma are evident from studies in the 1970s and 1980s documenting a significant number of asthma deaths that were attributable to failure of clinicians to recognize the clinical severity of asthma, thus leading to inadequate management. For example, a study of asthma deaths in the West Midland and Mersey regions of England in 1979 led to the conclusion that failure to recognize the severity of asthma delayed treatment, that routine asthma management was unsatisfactory, and that 86% of the deaths might have been preventable. A similar study in 1988 included the observation that a large number of asthmatics admitted to the hospital for observation were discharged with no escalation in the management of their asthma. This ultimately led the NHLBI to convene the first expert panel to develop guidelines for the diagnosis and treatment of asthma, and the resulting recommendations were published in 1991. Other guidelines soon followed, extending the NHLBI’s initial recommendations. Subsequent revisions have provided clinicians with a reliable framework for evidence-based management of patients with asthma. These guidelines emphasize maintaining long-term control of symptoms through attention to environmental and social components of asthma and using treatment regimens tailored to the severity of each patient’s symptoms. Crucial components of management include initial evaluation of severity ( Table 42-1 ) and ongoing assessment of control; appropriate pharmacologic therapy to reverse bronchoconstriction and ameliorate inflammation; identification and control of environmental factors that worsen symptoms or trigger exacerbations; and creation of a partnership between the patient and the health care professional to ensure that therapy is tailored to symptoms.
Components of Severity | CLASSIFICATION OF ASTHMA SEVERITY (YOUTHS ≥ 12 YR AND ADULTS) | |||
---|---|---|---|---|
Intermittent | PERSISTENT | |||
Mild | Moderate | Severe | ||
IMPAIRMENT | ||||
Symptoms | ≤2 days/wk | >2 days/wk but not daily | Daily | Throughout the day |
Nighttime awakenings | <2×/mo | 3–4×/mo | >1×/wk but not nightly | Often 7×/wk |
Short-acting β 2 -agonist use for symptom control | ≤2 days/wk | >2 days/wk but not daily | Daily | Several times per day |
Interference with normal activity | None | Minor limitation | Some limitation | Extremely limited |
Lung function | Normal FEV 1 between exacerbations | |||
|
|
|
| |
RISK | ||||
Exacerbations (consider frequency and severity) | 0–2/yr | >2/yr | >2/yr | >2/yr |
Frequency and severity may fluctuate over time Relative annual risk of exacerbations may be related to FEV 1 |
Assessment
Asthma Control
The assessment of patients with asthma involves five essential steps: determining the current degree of control based on symptoms, reliever medication use, recent exacerbations, and lung function; and evaluating the risk of future adverse outcomes. With this in mind, the NHLBI updated guidelines and the Global Initiative for Asthma (GINA) proposed a parallel scheme for asthma control based on expert opinion ( Table 42-2 ). The NHLBI and GINA categorizations represent categoric interval variables with threshold values. For example, GINA guidelines accept some daytime symptoms (<2 times/week) in the definition of “controlled” asthma, whereas nocturnal symptoms move a patient to the “partially controlled” category. The importance of control indices is underscored by the relationship between poor asthma control and substantial degrees of physical impairment and diminished quality of life, even after taking the baseline severity of asthma into account.
CLASSIFICATION OF ASTHMA CONTROL (YOUTHS ≥ 12 YR AND ADULTS) | |||
---|---|---|---|
Components of Control | Well Controlled | Not Well Controlled | Very Poorly Controlled |
IMPAIRMENT | |||
Symptoms | ≤2 days/wk | >2 days/wk | Throughout the day |
Nighttime awakening | ≤2 times/mo | 1–3 times/wk | ≥4 times/wk |
Interference with normal activity | None | Some limitation | Extremely limited |
Short-acting β-agonist use for symptom control (not prevention of EIB) | ≤2 days/wk | >2 days/wk | Several times/day |
FEV 1 or peak flow | >80% predicted/personal best | 60%–80% predicted/personal best | <60% predicted/personal best |
Validated questionnaires | |||
ATAQ | 0 | 1–2 | 3–4 |
ACQ | ≤0.75 * | ≥1.5 | N/A |
ACT | ≥20 | 16–19 | ≤15 |
RISK | |||
Exacerbations | 0–1/yr | ≥2/yr † | |
Consider severity and interval since last exacerbation | |||
Progressive loss of lung function Treatment-related adverse effects | Evaluation requires long-term follow-up care Medication side effects can vary in intensity from none to very troublesome and worrisome. The level of intensity does not correlate to specific levels of control but should be considered in the overall assessment of risk |
* ACQ values of 0.76–1.4 are indeterminate regarding well-controlled asthma.
† (1) The level of control is based on the most severe impairment or risk category. Assess impairment domain by patient’s recall of previous 2–4 wk and by spirometry or peak flow measures. Symptom assessment for longer periods should reflect a global assessment, such as inquiring whether the patient’s asthma is better or worse since the last visit. (2) At present, there are inadequate data to correspond frequencies of exacerbations with different levels of asthma control. In general, more frequent and intense exacerbations (e.g., requiring urgent, unscheduled care, hospitalization, or intensive care unit admission) indicate poorer disease control. For treatment purposes, patients who had two or more exacerbations requiring oral systemic corticosteroids in the past year may be considered the same as patients who have not-well-controlled asthma, even in the absence of impairment levels consistent with not-well-controlled asthma.
Several different validated instruments exist for assessing asthma, including the Asthma Control Questionnaire ( Table 42-3 ), Asthma Control Test ( Fig. 42-3 ), Asthma Therapy Assessment Questionnaire ( Table 42-4 ), and Asthma Control Scoring System ( Fig. 42-4 ). All are useful because they direct history taking, provide goals for the management of symptoms, and guide adjustments in treatment.
Please answer questions 1–6. | |
|
|
* This is a validated questionnaire that assesses asthma control during the prior week by determining the presence of symptoms of asthma and use of rescue medication.

|
Total points—0–4, with more points indicating more control problems |

Lung Function
Lung function testing is an important part of assessing asthma, both for making the initial diagnosis and as a means of evaluating the response to therapy. A study from the Genetics in Asthma Network suggested that FEV 1 and FVC are useful components of a standardized scheme for identifying the contribution of genes and environments to disease expression. Further proof of the importance of lung function testing was provided by a report that asthmatic patients with lower FEV 1 values were at significantly higher risk of needing acute care. Although the FEV 1 , FEV 1 /FVC ratio, and PEF are useful in the diagnosis and monitoring of asthma, the utility of these measurements in improving the outcome of asthma remains incompletely defined. However, a low prebronchodilator FEV 1 is a strong predictor of decline in asthma control and a low postbronchodilator FEV 1 is a marker of future risk. In mild asthma, however, FEV 1 is often normal or near-normal and thus may be poorly responsive to bronchodilators.
PEF measurement is a standard method to correlate physiologic function with asthma severity and has been used as a marker of asthma control in many clinical trials. However, individual peak flow measurements are highly variable and the variability in peak flow has a greater predictive value for future exacerbations than individual PEF measurements themselves. The clinical usefulness of peak flow measurements is also limited by patient resistance to home monitoring and difficulty with consistently maintaining peak flow records.
Biomarkers
The emergence of an increased body of literature to support the presence of asthma endotypes has made it imperative that we develop and identify biomarkers associated with each asthma type. These biomarkers will become increasingly important in determining the specific therapies that are administered to a particular patient. Currently, there are two accepted types of inflammation in asthma based on the presence or absence of Th2 inflammation: a “Th2 high” phenotype that is characterized by the presence of peripheral eosinophilia, sputum eosinophils, elevated FeNO and/or increased markers associated with Th2 inflammation (e.g., serum periostin) and a “Th2 low” phenotype characterized by the absence of any of these markers of Th2 high status. In fact, the Th2 low phenotype has no known biomarkers and this phenotype clearly requires additional study.
Before the introduction of specific biomarkers, elevated blood eosinophils were proposed as a means to identify patients with predominant Th2 inflammation. Alterations in blood eosinophil levels are associated with response to various therapies, and increased eosinophils are correlated with more severe disease. A blood eosinophil level of greater than or equal to 0.3 × 10 9 /L (300 cells/µL) has become increasingly accepted as a peripheral marker of eosinophilic inflammation. An elevated level of blood eosinophils is predictive of response to anti-IL-5 therapy.
High sputum eosinophil counts predict response to inhaled corticosteroids and an increased risk of future exacerbations, and an increase in sputum eosinophil counts following corticosteroid dose reduction predicts future deterioration of asthma control. A change of 50% in sputum eosinophil counts is considered to be a clinically significant marker of response to therapy. However, sputum eosinophils may be difficult to quantify in clinical practice and their current value is primarily in clinical research.
FeNO has some advantages compared with counting the number of sputum eosinophils. Measurement of FeNO can be performed rapidly in a primary care setting, requires less technical expertise, and provides real-time objective physiologic data. In particular, when accompanied by more traditional diagnostic tools, it may be used to identify eosinophilic asthma. ATS guidelines support the use of FeNO for the diagnoses of eosinophilic airway inflammation and asthma when objective evidence is necessary. FeNO concentrations less than 25 ppb (<20 ppb in children) suggest that eosinophilic airway inflammation and responsiveness to corticosteroids are unlikely; elevated levels suggest eosinophilic airway inflammation and responsiveness to corticosteroids. Major limitations of using FeNO to confirm the diagnosis of asthma include the relatively high prevalence of noneosinophilic phenotypes that are characterized by a lack of increased FeNO and difficulty with clinical interpretation of values obtained in the setting of concomitant steroid use that may lead to false-negative results. Of note, the ATS guidelines predominantly apply to patients with mild to moderate asthma. A recent ERS/ATS task force recommended against the routine use of FeNO to diagnose asthma and to evaluate response to therapy in patients with severe disease. However, there is still a role for FeNO in phenotyping patients by identifying those with a Th2 high/eosinophilic phenotype, regardless of asthma severity.
Serum total immunoglobulin E (IgE) has been used to identify patients with asthma who are likely to respond to therapy with omalizumab, a drug that targets IgE. Omalizumab prevents IgE from binding to its receptor on the cell surface and reduces the amount of free IgE present in the blood. There are currently no commercially available assays to measure free IgE. Baseline IgE is only a modest predictor of response to omalizumab therapy.
Periostin is secreted by bronchial epithelial cells and fibroblasts in response to IL-4 and IL-13. Periostin is expressed in asthma patients and can be measured in the serum. Periostin is an emerging biomarker that offers some advantages over peripheral eosinophilia and FeNO because serum levels of periostin tend to be less variable, correlate well with tissue eosinophilia, and are not altered by treatment with steroids. More importantly, serum periostin is predictive of response to therapy with biologic agents that target IL-13, making its future use as a biomarker much more promising. Periostin is the first biomarker in asthma to be directly correlated with the underlying pathophysiology and makes the possibility of personalizing asthma therapy more likely to be realized.
The increased interest in identifying biomarkers for use in clinical practice and standardizing of biomarkers obtained in asthma clinical trials led to the convening of a task force to identify biomarkers that should be included in asthma outcomes. The task force recommended FeNO, sputum eosinophils, blood eosinophils, total IgE, allergen-specific IgE, urinary leukotriene E 4 , and several new emerging markers. The emerging markers include airway imaging, exhaled breath condensate, and proteomic and genomic techniques to identify signatures in tissue obtained from patients with asthma. Although these rapid throughput methods are promising, they may be years away from clinical application. The current biomarkers are summarized in Table 42-5 .
Biomarker | Asthma Phenotype | Predicts |
---|---|---|
Periostin | Th2 high | Response to anti-IL-13 and IL-4 therapy |
Elevated exhaled nitric oxide (>50 ppb in adults, >35 ppb in children) | Th2 high | Response to inhaled steroids |
Sputum eosinophils >3% | Th2 high | Response to inhaled steroids |
Peripheral eosinophils (>0.3 × 10 9 /L or 300/µL) | Th2 high | Response to anti-IL-5 therapy |
Elevated total IgE > 30 IU | Allergic | Response to omalizumab |
Allergy skin tests and elevated specific IgE | Allergic asthma with atopy | Response to immunotherapy, omalizumab |
Lack of elevated peripheral and sputum eosinophils and low FeNO | Th2 low | Response to tiotropium and macrolides (likely to be poor responders to steroids) |
Asthma Treatment Approaches
Overview
Current guidelines recommend adjusting therapy in a stepwise fashion to reduce daily symptoms and risk of exacerbations while minimizing the use of medications ( Fig. 42-5 ). The hierarchy of treatment recommendations is based on the available literature about efficacy and safety and provides a prominent role for controller medications at all levels of asthma treatment. In general, the strategy and recommendations in adults and children are similar. However, the increased risk of adverse effects and/or the lack of safety data in pediatric patients for theophylline, omalizumab, and the 5-lipoxygenase inhibitor zileuton led to their exclusion from current pediatric treatment guidelines ( eFigs. 42-1 and 42-2 ).

Specific Pharmacologic Agents
β 2 -agonists.
β 2 -agonists have been used for thousands of years in the form of the ephedrine-containing herbal preparation, ma huang. However, it was not until the 1940s, when the nonselective β-agonist isoproterenol was introduced, that β-agonists became the standard of care for treating airway disease. The subsequent development of the β 2 -selective agents, albuterol and terbutaline, with improved side effect profiles, led to their current role as the cornerstone of asthma therapy.
β 2 -agonist activity is primarily mediated by binding to a specific G-coupled transmembrane receptor found in high abundance on ASM. Receptor binding leads to increased intracellular cyclic adenosine monophosphate and relaxation of ASM. Notably, β 2 -receptors are also found on other resident airway cells, including airway epithelial cells and circulating immune cells, and binding to these sites may reduce vascular permeability and inflammatory mediator release. One concern raised about the chronic use of β 2 -agonists is that of receptor desensitization. As with most signaling receptors, repeated exposure to β 2 -agonists can lead to decreased responsiveness of membrane receptors through receptor down-regulation via receptor endocytosis and uncoupling of receptors from downstream transduction pathways. This response appears to be self-limited and minor in ASM.
β 2 -selective agonists are usually administered by aerosol, which maximizes delivery to target tissue (ASM), while minimizing the exposure of nontarget tissues. Both short-acting β 2 -agonists (SABAs) and LABAs are available, and the onset and duration of action are primarily related to lipophilicity. Short-acting agents are used for rescue or emergent treatment, whereas LABAs are used in combination with inhaled corticosteroids for chronic management.
A number of highly selective SABAs are now available, and all have a rapid onset of action with peak action between 60 and 90 minutes. Because they are inhaled directly into the airways, systemic side effects are minimal, even at high doses. A β 2 -agonist preparation containing only the active enantiomer of albuterol was developed in an attempt to minimize side effects. However, clinical trials have not shown significant differences in outcome or tolerability compared with racemic albuterol. On the basis of current guidelines, these medications should be used to treat symptoms not adequately controlled on a regimen of long-acting controller agents. Increased frequency of SABA use is a sign of inadequate control of symptoms or overreliance on rescue medication. Patients using more than one canister a month or requiring excessive doses of rescue inhalers (defined as > 2 doses/week) should be considered for a step-up in therapy.
LABAs have minimal utility for treating acute asthmatic symptoms because of their delayed onset of action. However, in patients with inadequately controlled asthma, they can be added to an inhaled corticosteroid (ICS) to improve symptoms and efficacy of the ICS. Formoterol and salmeterol are the two LABAs available. Formoterol has a shorter onset of action than salmeterol, whereas salmeterol has a longer duration of action. Each has been shown to improve lung function, reduce symptoms, and reduce the frequency of exacerbations.
Adverse events associated with the use of β 2 -agonists are primarily due to unwanted activation of β 2 -receptors in nonpulmonary tissue. Cardiac stimulation causes tachycardia and arrhythmias, whereas skeletal muscle stimulation causes tremors and hypokalemia. Since the introduction of β-agonists, there has been concern about the clinical implications of receptor desensitization. Two unique SABAs, isoproterenol forte and fenoterol, were implicated in two “epidemics” of increased asthma deaths in the 1960s and 1970s, respectively ; later analysis suggested that this might have been due to excessive use, rebound bronchoconstriction due to short duration of action, tachyphylaxis, or lipophilicity. After these agents were withdrawn from the market, mortality declined and the safety profile of subsequent SABAs has been good. Clinical data suggest that “as-needed” versus scheduled SABA treatment is associated with improved physiology and fewer, less severe exacerbations.
Adverse events with LABAs have also been of concern, particularly because of their long duration of action. In the Salmeterol Multicenter Asthma Research Trial (SMART), patients were randomized to salmeterol or placebo plus usual care, with approximately 13,000 patients in each arm. There was no significant difference in risk of death in either group, but subgroup analysis identified a small but increased risk of death that was more prominent in African American subjects in the salmeterol arm of the trial. However, the African American patients also had poorer control of symptoms at baseline (with more exacerbations and hospitalizations) and a lower rate of inhaled corticosteroid use than the white patients. Importantly, the use of LABAs with inhaled corticosteroids in the same metered-dose inhaler has been shown to be safe and effective.
Several studies have explored the relationship between polymorphisms in the β 2 -receptor and responses to β-agonist therapy. Specifically, in patients treated with scheduled albuterol, the presence of the Arg/Arg polymorphism at the codon 16 locus (B16) was associated with lower PEF and FEV 1 , and increased symptoms, more rescue inhaler use, and a higher rate of exacerbations as compared with patients who were Arg/Gly or homozygous Gly/Gly. All of these symptoms improved when regular albuterol was withdrawn. Use of LABAs (with and without inhaled corticosteroids) has been shown to worsen physiologic markers of respiratory function in Arg/Arg patients in some studies, although these findings are not consistent. The LARGE study demonstrated beneficial effects of LABAs in combination with ICS irrespective of B16 genotype, and the authors recommended against alterations in the current use of LABA. Further research is required; however, thus far there are no indications that use of LABA in the Arg/Arg genotype is associated with undue risk. Additional genetic studies are examining potential mechanisms by which β-adrenergic receptor polymorphisms may contribute to the risk of LABA therapy in some patients with asthma.
LABAs have modest effects on improving asthma control and reducing severe exacerbations, with an uncertain safety profile. Despite the conflicting data on the safety of LABA therapy in certain populations, the U.S. Food and Drug Administration (FDA) felt that there was enough concern to warrant the addition of a black box warning on all drugs containing LABAs. The use of LABAs should be judicious, and clinicians should strive to discontinue these medications if patients no longer require them. Additionally, it is critical to prescribe LABAs only with concomitant ICSs and to warn patients about the potential dangers of LABA monotherapy. In fact, the FDA recommends the use of combination inhalers rather than two separate inhalers in order to avoid the possibility of patients using monotherapy. In 2010 the FDA mandated that manufacturers of drugs containing LABAs conduct large studies to demonstrate safety, and these studies are ongoing.
Other Bronchodilators.
Anticholinergic agents act as bronchodilators via competition with acetylcholine at neuromuscular junctions, thereby blocking transmission of bronchoconstrictor reflexes. The short-acting anticholinergic ipratropium bromide is effective in patients with COPD but is considered a second-line agent for treating asthma, most likely because cholinergic tone contributes less to bronchoconstriction in asthma. Although no trials have compared short-acting anticholinergics with SABAs, randomized trials studying the addition of anticholinergics to β-agonists do not show any additional benefit in patients with chronic asthma. However, specific asthma phenotypes might be more likely to respond to anticholinergic treatment, including patients with fixed airflow obstruction, advanced age, or longer duration of disease. Furthermore, anticholinergics may be an acceptable alternative for certain subgroups of patients who do not tolerate β-agonist treatment or for patients with the Arg/Arg B16 genotype, although this has not been studied directly.
Tiotropium, a long-acting anticholinergic agent, has been shown to be beneficial for treating the symptoms of COPD. Spirometric improvement has been demonstrated in case series of asthmatics who do not have sputum eosinophilia and asthmatics with concomitant COPD. Three recent randomized controlled trials have indicated a role for tiotropium as add-on therapy to both inhaled corticosteroids and ICS/LABA combination therapy in patients with moderate to severe asthma with improved markers of airway function. Bronchodilator responsiveness to albuterol, higher resting cholinergic tone (defined by a lower resting heart rate), and increased airway obstruction (defined by a lower FEV 1 /FVC ratio) are predictors of response to tiotropium. Although not yet approved for asthma, it is expected that it will be approved within the next 3 to 5 years.
Inhaled Corticosteroids.
With recognition of the central role of inflammation in the pathophysiology of asthma, contemporary treatment strategies now emphasize inhaled corticosteroids for long-term control of symptoms. Corticosteroids suppress inflammatory responses through a broad influence on signal transduction and gene expression pathways. Corticosteroids bind to a specific cytoplasmic receptor that translocates to the nucleus, where it modulates expression of inflammatory genes through inhibition of histone acetyltransferases and recruitment of histone deacetylases, two classes of histone modifiers that control DNA unwinding and gene expression epigenetically. Airway inflammatory cell influx and markers of airway inflammation in asthma are reduced by corticosteroid administration.
Systemic corticosteroids have been used in the treatment of asthma since the 1940s and continue to be a cornerstone of the management of acute exacerbations. However, systemically administered corticosteroids are associated with a variety of undesirable side effects. The introduction of ICSs in the 1970s ushered in a new era in the treatment of asthma. As with bronchodilators, delivery of drug directly into the lungs through the use of inhaled preparations minimizes systemic toxicity and improves therapeutic benefit.
The use of ICSs improves all aspects of asthma control. ICSs reduce asthmatic symptoms, improve lung function, decrease airway inflammation, and control AHR. A large meta-analysis showed that ICSs reduce asthma exacerbations by 55% when compared with placebo and reduce the risk of hospitalization by 50% when compared with use of as-needed β-agonists alone. Furthermore, risk of death from asthma is reduced in a dose-response manner with the use of ICS. As a result, ICSs are considered to be first-line therapy for all patients requiring more than twice-weekly β-agonist use. Predictors of response to inhaled corticosteroids include FeNO level greater than 47 ppb, reversibility to albuterol and a decreased FEV1, and the presence of increased sputum eosinophils (>2% to 3%). Interestingly, a large proportion of asthma patients are persistently noneosinophilic and unlikely to respond to therapy with ICS, supporting the need for standard phenotyping of asthma with subsequent personalized therapy.
The initial recommendations regarding the use of ICS in patients with mild to moderate persistent asthma emphasized their use on a daily to twice-daily basis. Recently, there has been increased evidence that ICSs can be efficacious at reducing asthma symptoms and achieving asthma control when used intermittently in both children and adults. Despite these studies, there is still debate about the intermittent use of ICS; certainly ICS should not be used intermittently in patients with severe disease.
There are multiple different inhaled corticosteroid preparations and delivery devices on the market ( Table 42-6 ). The inhalers differ in particle size ranging from a median mass aerodynamic diameter of about 1 micron to those with large particles that are 2 to 5 microns in size. The delivery devices include dry powder inhalers and metered dose inhalers. The choice of inhaled corticosteroids is based on the physician’s discretion about the patient’s needs and often rests on cost, convenience of dosing, and reduction of side effects.
ICS Generic/Trade Names | Dosage Forms | Age | Low Daily Dose | Medium Daily Dose | High Daily Dose |
---|---|---|---|---|---|
| HFA MDI: 40 or 80 µg/puff | 5–11 | 80–160 | >160–320 | >320 |
≥12 | 80–240 | >240–480 | >480 | ||
| Respules for nebulization: 0.25, 0.5, 1.0 mg/neb | 0–4 | 0.25–0.5 | >0.5–1.0 | >1.0 |
5–11 | 0.5 | 1.0 | 2.0 | ||
Flexhaler DPI: 90 or 180 µg/inh | 5–11 | 180–400 | >400–800 | >800 | |
≥12 | 180–600 | >600–1200 | >1200 | ||
Symbicort HFA MDI: 80/4.5 or 160/4.5 µg/puff | ≥12 | 320 (80/4.5 2 puffs bid) | 640 (160/4.5 2 puffs bid) | ||
| HFA MDI: 80 or 160 µg/puff | 5–11 * | 80–160 | >160–320 | >320 |
≥12 | 160–320 | >320–640 | >640 (Mfr highest recommended dose 640 µg/day) | ||
| HFA MDI: 44, 110, or 220 µg/puff | 0–11 | 88–176 | >176–352 | >352 |
≥12 | 88–264 | >264–440 | >440 | ||
Flovent Diskus DPI: 50, 100, or 250 µg/inh | 5–11 | 100–200 | >200–400 | >400 | |
≥12 | 100–300 | >300–500 | >500 | ||
Advair HFA MDI: 45/21, 115/21, or 230/21 µg/puff | 4–11 | 180 (45/21 2 puffs bid) | 460–920 (115–230/21 2 puffs bid) | ||
≥12 | 180 (45/21 2 puffs bid) | 460 (115/21 2 puff bid) | 920 (230/21 2 puffs bid) | ||
Advair Diskus DPI: 100/50, 250/50, or 500/50 µg/inh | 4–11 | 200 (100/50 1 inh bid) | 500–1000 (250–500/50 1 inh bid) | ||
≥12 | 200 (100/50 1 inh bid) | 500 (250/50 1 inh bid) | 1000 (500/50 1 inh bid) | ||
| Asmanex Twisthaler DPI: 110 or 220 µg/inh | 4–11 | 110 (Mfr highest recommended dose 110 µg/day) | 220–440 | >440 |
≥12 | 220 | 440 | >440 (Mfr highest recommended dose 800 µg/day) | ||
Dulera HFA MDI: 100/5 or 200/5 µg/puff | ≥12 | 400 (100/5 2 puffs bid) | 800 (200/5 2 puffs bid) |
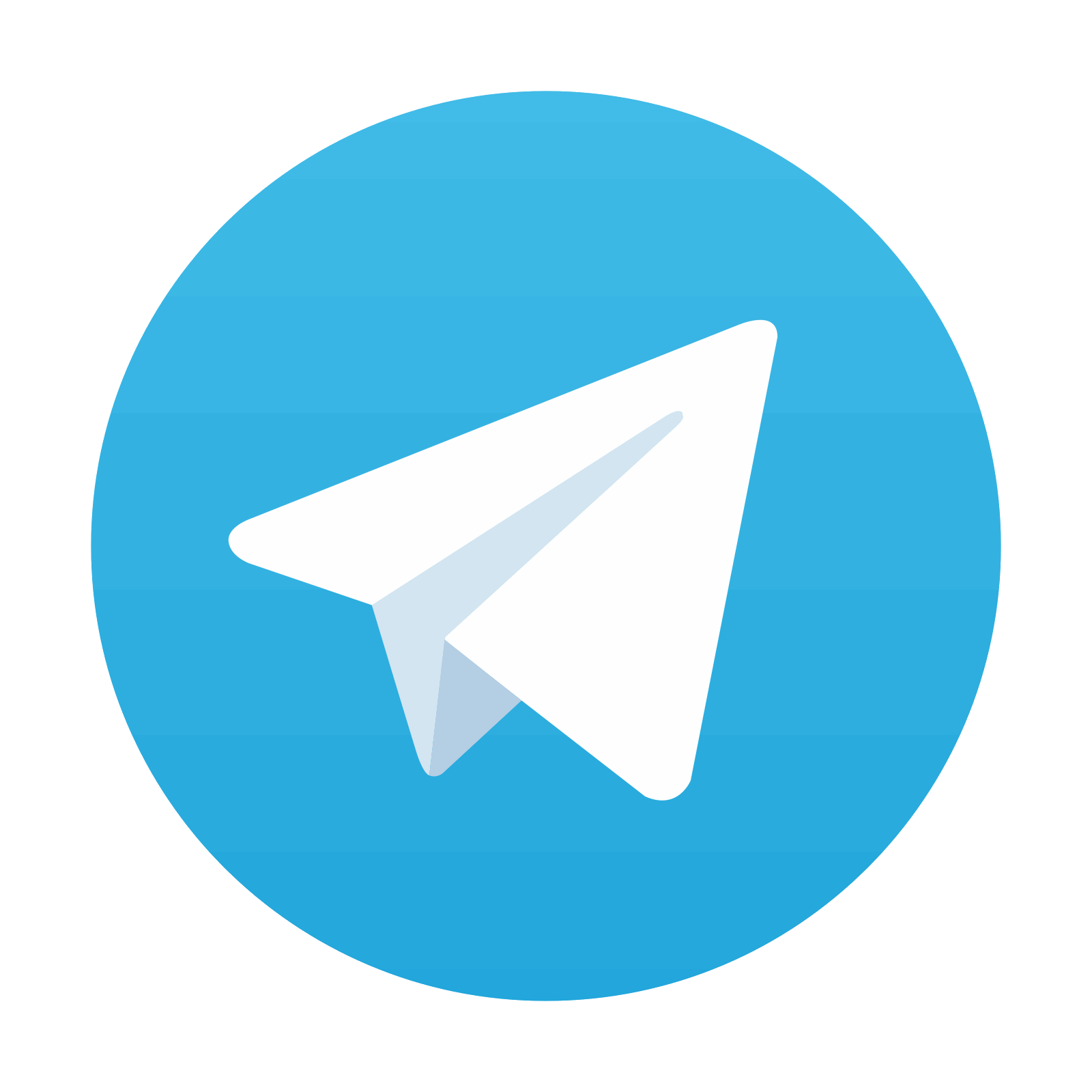
Stay updated, free articles. Join our Telegram channel

Full access? Get Clinical Tree
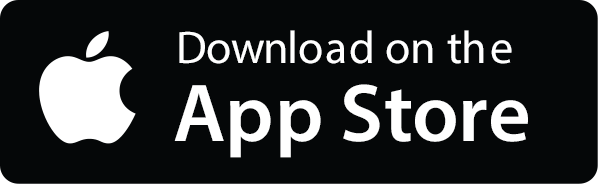
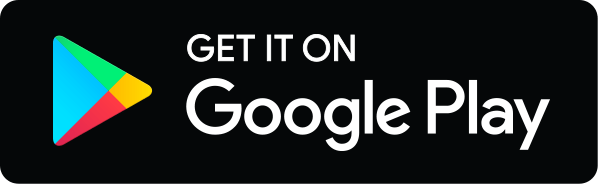
