Chapter 24 Cost-Effectiveness of Myocardial Perfusion Single-Photon Emission Computed Tomography
INTRODUCTION
Since peaking in the mid-1960s, reductions in mortality from coronary artery diseases have approached 50%.1 Although a number of reasons have been put forth for this marked decline in death rates, a proportion of this reduction can be attributed to early and effective diagnostic testing techniques that result in improved outcomes for at-risk patients. Along with marked improvements in outcome, procedural utilization rates have skyrocketed. The current focus on the part of public and private health care payers is to contain “out of control” health care costs. Health care payers have reacted by focusing on programs to decrease reimbursement levels and to manage utilization of major growth procedures such as myocardial perfusion single-photon emission computed tomography (SPECT).2 In fact, myocardial perfusion SPECT has been one of the largest growing procedures and is estimated to encumber approximately 2% of annual Medicare expenditures. To this end throughout the last few decades, there have been shifting foci between marked improvements in disease detection with medical advances in new technology and the reverberating excess expenditures associated with such developments. As a result, there has been a focus on developing rational approaches to containing health care costs and curbing unrestrained spending. The development of evidence-based medicine is an attempt to set guidelines for thresholds of evidence to justify the cost of a procedure. The bar has been set very high for diagnostic procedures where a test must now result in a net health improvement in clinical outcome (i.e., be effective). This is at the heart of cost-effectiveness analysis: striking a balance between spending and value. For a diagnostic test in cardiology to be accepted and receive widespread utilization, using evidence-based standards, it must have a clear and demonstrated economic and clinical incremental value when compared with other modalities. Nuclear cardiology has a clear advantage with regard to setting higher standards of evidence, owing to the large (unparalleled) body of evidence from multicenter, observational series and randomized or controlled clinical trials from statistically powered, diverse patient populations. The current chapter will provide a synopsis of available evidence of gated myocardial perfusion SPECT, as well as review the guiding principles applied in the development of cost-effectiveness evidence.
HEALTH CARE COSTS FOR CARDIOVASCULAR DISEASE
In the United States, total health care costs for cardiovascular disease approached $450 billion in 2007.3 In fact, lifetime costs for one symptomatic patient are reported at $1 million dollars.4 Given that nearly 19 million patients have chronic stable angina, these costs of care are astronomical. It is then no wonder that the Medicare Panel Expenditure Survey ranked ischemic heart disease as the most expensive condition.5 Recent estimates from the American College of Cardiology have noted that approximately 38% of all payments to cardiologists were for echocardiography and nuclear imaging.6 Estimates from the 34th Bethesda Conference on atherosclerotic imaging noted an estimated 40 million noninvasive cardiac tests were performed during each of the past few years,7 with total costs of over $1 billion in the year 2000.3 For example, every year, a total of 6 million exercise electrocardiograms are billed. In the area of imaging, approximately 8.5 million nuclear scans are performed.6 A report from the American College of Radiology reported annual growth rates for myocardial perfusion imaging exceeding 30% for cardiologists.8 The largest growth sectors have been in the outpatient setting where reimbursement strategies have been more favorable. Given our aging population, as well as the dramatic increases in diabetes and obesity, it is estimated that there will be a continuing need for diagnostic imaging services to identify patients at risk for coronary artery disease (CAD). However, the challenge is to adequately service this growing population of at-risk patients with limited financial means within our current environment.
With the recent history of marked growth in nuclear cardiology, payers consider this test a “big target” for focusing cost-reduction efforts—in many cases, encouraging shifting to lower-cost procedures such as stress echocardiography. Thus the current review hopes to frame the utilization as supported by effective evidence to guide medical decision making, as well as to consider the advantages of a more noninvasive center approach to contain large health care costs associated with invasive coronary angiography. Similar to nuclear imaging, growth rates for cardiac catheterization have increased 300%+ with, on average, a third of catheterizations being considered inappropriate or not supported by accepted clinical indications.9 From a clinical point of view, it is easy to envision that an overuse of coronary angiography can lead to higher rates and in some cases the unnecessary use of coronary revascularization procedures, defined as utilization that is not supported by evidence noting substantive improvements in outcome. Although SPECT imaging growth rates have been high, the cost savings resulting from decreased angiographic utilization, as will be illustrated later in this chapter, have been reportedly quite substantial. This is particularly true of patients with stable chest pain symptoms who are referred to diagnostic left heart catheterization.
Prior to discussing the available economic evidence, it will be helpful to define the calculation of cost-effectiveness and related standards for health policy decision making. An incremental cost-effectiveness ratio (ICER) requires not only necessary information on cost but also integrated evidence on diagnostic test accuracy and the ensuing therapeutic benefit of treatment.10 Cost-effectiveness analyses are increasingly favored within health care systems where health care resources are increasingly finite; ICER is a tool to assist decision makers to assess and devise cost containment and value within their health care system. ICER is defined as an incremental or marginal cost-effectiveness ratio because it compares more than one test, therapy, or patient management approach. In general for cardiac imaging modalities, there are a number of published cost analyses.7,11–26
A challenge with this reasoning is that for many comparisons, nuclear cardiology is a more expensive test. However, more expensive procedures can be cost-effective if they reduce induced costs or are substantially more effective. There are several scenarios in which nuclear cardiology may result in a favorable ICER. For the first case, if nuclear cardiology is less expensive and equally effective, then the result could be a favorable ICER. A favorable ICER could also result for nuclear cardiology testing even if it is initially more expensive but results in a reduction in downstream procedures with equally effective outcomes results. And finally, nuclear cardiology testing could be substantively more costly but also have a favorable ICER should it result in decidedly better outcome results. All of the above are simplified examples of how nuclear cardiology could be cost-effective; greater details of the methods for these types of analyses will be provided in the following discussions (Fig. 24-1).
EVALUATING PROCEDURAL COST
Although much has been made of the variability in imaging costs, it is helpful to examine data on the unit operating cost of a variety of cardiac diagnostic procedures.7 There are two methods that can be applied to estimating cost: top-down and bottom-up. A top-down cost approach uses adjusted (technical and professional) charges and is calculated as the charge × the hospital-specific cost-charge ratio (set by the Center for Medicare and Medicaid Services). Recently, bottom-up costs have been employed and involve calculating both the fixed and variable labor costs (e.g., supplies, equipment, and labor costs). Using this type of calculation, procedural cost is predominately driven by laboratory volume, where high-volume laboratories have lower cost per test, achieving economies of scale with increasing volume. For SPECT imaging, cost is also influenced by noncardiac procedural volume. The use of quantitative scoring systems, such as calculating left ventricular ejection fraction, have lower labor inputs and are generally lower cost than systems utilizing greater labor components, especially those including physician labor. In large part, the use of quantitative scoring systems generally not only lowers initial costs but (because of improved reproducibility) also aids physician diagnostic confidence and the impact on induced posttest costs.27
For SPECT imaging, the cost of radioisotopes and pharmacologic stress agents is also included in the unit cost of a test. Recently, the commonly used technetium-99m sestamibi became generic, allowing for lower radioisotope costs to be realized. Factors that also influence test cost include labor and equipment necessary for laboratory standards or certification. A major component of the unit operating cost is equipment, where costs can vary widely from less than $1 million to up to $3 million for magnetic resonance, positron emission tomography, or multislice computed tomography scanners. Despite these high cost estimates, discounted prices and leasing agreements are common and reduce equipment prices substantially for most imaging modalities. The equipment space (e.g., cost at total square footage) and any necessary requirements (e.g., added concrete thickness for magnetic resonance imaging) for the equipment should be included in the equipment or fixed cost estimate. In the final step in calculating costs, estimates are then discounted and inflation-corrected to a given time period in order to establish a common metric for individual procedural estimates and for comparisons to other modalities.28,29
A review of current estimates for diagnostic procedures has been reported in several publications and is depicted in Figure 24-2.7 Cost estimates should be viewed for all the modalities as “guesstimates,” because there have been few attempts to calculate the true unit operating cost of each procedure. New imaging modalities in general have higher initial costs, but with greater experience and efficiency and equipment price reductions, cost estimates will decrease. Inexpensive tests include those that are “low tech” such as exercise electrocardiography. When integrating the available data, nuclear cardiology procedures appear to be in the midrange of diagnostic procedure cost estimates.
Adding Downstream Costs
Although rarely considered, the total cost of a procedure not only should include the upfront costs but should be totaled throughout the episode of care (importantly, including induced costs). Drummond and Jefferson30 have advised that any economic evaluation should consider the impact of test use on downstream resource consumption, including both additional procedure use and treatment costs. From a societal point of view, cost estimates should also consider those consumed by the patient, or indirect costs. By definition, indirect costs include travel time, out-of-pocket expenses, and days lost from work for both the patient and other caregivers. Downstream costs should also consider the induced costs of incidental findings, and this latter point will be a more critical cost component in vascular imaging. More recently, reports have been published on the radiation exposure associated with a number of cardiac imaging procedures. The associated cancer risk is also one component of cost that is rarely included but should be considered as a component of induced cost.31
Although precise delineation of induced costs may be difficult to enumerate, there is an expected proportional relationship between test results and expected costs of care.32 That is, greater costs are expected for higher-risk test results (e.g., significant left ventricular dysfunction and multivessel or severely abnormal perfusion abnormalities), particularly in higher-likelihood patients. As expected, this latter population will require higher rates of coronary angiography, as well as revascularization procedures and (because of elevated, cumulative event rates) greater hospital admissions for acute coronary syndromes and lost life years as a result of premature death. Estimates of induced costs have revealed that the costs of downstream hospital and procedural care can be approximately 10- to 100-fold higher than the initial test cost itself.32
An easy method for estimating downstream care costs is to calculate a test’s negative and positive disease rates, and this may be used to define cost waste induced by the procedure. True negative (lowest cost cohort) and positive tests result in effective resource consumption or costs as a result of their accurate identification or exclusion of disease. Of course, the economics of test misclassification can be affected by changes to the positivity threshold, the prevalence of disease in the population, and the length of follow-up for evaluating, in particular, false-negative results.33 By definition, a false-positive test result is documented when a patient with abnormal SPECT results undergoes coronary angiography revealing normal or insignificant disease (Table 24-1). Of course, the defining of an intermediate stenosis that elicited a flow limitation may be wrongly termed a false positive. Yet it may be viewed as a false positive insofar as the abnormal nuclear finding failed to define revascularizable disease and, as such, from that perspective may be termed a false positive. This latter point suggests that the defining of a false positive should be used cautiously as a measure of cost inefficiency. Despite this, when downstream testing results in a lack of confirmatory findings, then the initial results misclassified the expected disease state in that patient. Repeated, redundant, and unnecessary testing contributes to high costs of care. Although false-positive results can be difficult to assess, many studies do not allow a sufficient time period for follow-up to discern the rate of false-negative test results. A false-negative test result would be expected to occur when a patient with initially normal findings has an adverse event, or disease is confirmed at a later date. There are additional challenges with negative findings: They may change a patient’s health-seeking behaviors, resulting in delays to treatment as well as litigation costs that are rarely considered in an economic analysis.34 It is impossible to expect that any test would perfectly classify patients 100% of the time, and there are no standards for acceptability of false-negative and false-positive findings; however, a low rate of false-negative results is preferable (i.e., perhaps < 10%), because false-negative results are more costly and harmful to the patient.
Table 24-1 Cost Advantages Using Myocardial Perfusion Single-Photon Emission Computed Tomography
High sensitivity excludes disease more accurately and avoids the need for a secondary test if a less accurate primary test is used. |
High sensitivity leads to fewer false-negative test results and avoids the cost of future events in patients with undiagnosed disease. |
High specificity reduces the number of false-positive test results and consequent downstream testing. |
Additional prognostic information avoids the need for further prognostic testing and focuses high-cost interventional care on patients with advanced disease with the most to gain in terms of improvement in clinical outcome. |
DEFINING INCREMENTAL EFFECTIVENESS
In addition to delineating the costs of a diagnostic procedure, there are a variety of methods to define its effectiveness. There have been recent active discussions within health policy arenas as to what is the correct method to define the effectiveness of a diagnostic procedure. Historically, the diagnostic accuracy of a test has been the mainstay for gaining U.S. Food and Drug Administration (FDA) approval. More recently there has been an abundance of evidence on the prognostic accuracy of testing, including a large body of evidence on risk stratification with nuclear cardiology that is discussed in Chapters 15 and 16. A new standard of net improvement in health outcome has been introduced as a standard upon which to guide reimbursement. Where evidence for a given modality meets the criteria of net improvement in health outcome, then reimbursement should be favored for that given test or patient indication. In terms of defining what a net improvement in outcome means, patients must be “better off” after undergoing the diagnostic test when compared to no testing or an alternative modality. This type of examination of the effectiveness of a diagnostic test has to do with how the procedure is used to guide subsequent decisions on starting, stopping, or modifying therapies.35 Other positive improvements in health outcomes following a diagnostic test include:
DEFINING INCREMENTAL COST-EFFECTIVENESS RATIO
For many clinicians, cost-effectiveness analysis can seem a bit obtuse; however, it is simply a ratio that reflects the amount of resources needed to change a patient’s outcome. In other words, it is used to reflect the intensity of management in relation to any given outcome achieved. The aim of a cost-effectiveness analysis is to reflect or mirror clinical decision making where physicians make choices based on the information content and, generally, the invasive nature of the procedure (i.e., a surrogate for cost). A cost-effectiveness ratio (ICER) is most commonly expressed in cost per life year saved or, if adjusted by patient functional gain, in a modification as cost per quality-adjusted life year saved. For ICERs, cost per life year saved is rapidly becoming a common metric for comparisons to other medical interventions. A compendium of ICER data can be compiled in the form of a league table for comparisons to other medical and nonmedical procedures, therapies, and so forth.32–57 Such comparisons may be more relevant to the health care policy analyst, but for the clinician, the link to an ICER for any given diagnostic test is best understood by a combination of factors that integrate accuracy, and resulting treatment efficacy and management intensity and timing. That is, a diagnostic test that is effective at identifying patients whose ensuing risk may be altered by aggressive therapeutic intervention will result in an aversion to more costly, end-stage care, thus resulting in cost-effective care for similar patients.
The theoretical approaches to adding value and improved cost-effectiveness with noninvasive testing are noted in Table 24-2. Tests that are ineffective result in redundant testing with rising cost-ineffective care. Simply stated, diagnostic tests that have high rates of false-negative and false-positive test results have excessive cost waste and result in ICERs that are not economically attractive for the health and well-being of our society. High rates of false-positive tests lead to greater use of unnecessary coronary angiography, and high false-negative rates lead to higher rates of acute coronary syndromes in patients with initially negative results. This inefficiency leads to patient care that does not improve outcome and is cost inefficient.
Table 24-2 Theoretical Approaches to Adding Value and Improved Cost-Effectiveness With Noninvasive Testing
Adding Value but Minimizing Cost Through Improved Test Accuracy Combination of Physiologic/Anatomic Assessment Adds to Cost Efficiency |
For example, gated SPECT imaging, including evaluation of myocardial perfusion and global ventricular function |
Containing Diagnostic Test Costs |
For example, initial exercise electrocardiography followed by a cardiac imaging test (e.g., SPECT) in patients who have indeterminate or mildly positive ST-segment changes; lower overall evaluation costs, especially for patients with a normal resting 12-lead electrocardiogram |
Tiered approach to testing, with a selective use of higher cost test for example, SPECT to those higher-risk patients |
Lower Costs Tests Applied to Non-High-Risk |
Selective coronary angiography in patients with SPECT ischemia |
Using SPECT as a gatekeeper to conventional angiography, especially for patients with stable chest pain symptoms. The results of this strategy could be: |
A reduction in procedural complications, hospital costs, and overall “workup” cost for a diagnostic catheterization; in-laboratory complications are ~1%. |
An incremental or marginal cost-effectiveness ratio includes a comparison of the differences in cost and effectiveness of more than one imaging modality. As previously stated, an ICER includes the calculation of upfront and downstream cost differences as well as near-term and/or long-term (i.e., life expectancy) outcome differences. Based on early work done on the evaluation of renal dialysis programs, the threshold for economic efficiency is set at less than $50,000 per life year saved (LYS), with many countries setting thresholds as low as less than $20,000 per LYS.7,32
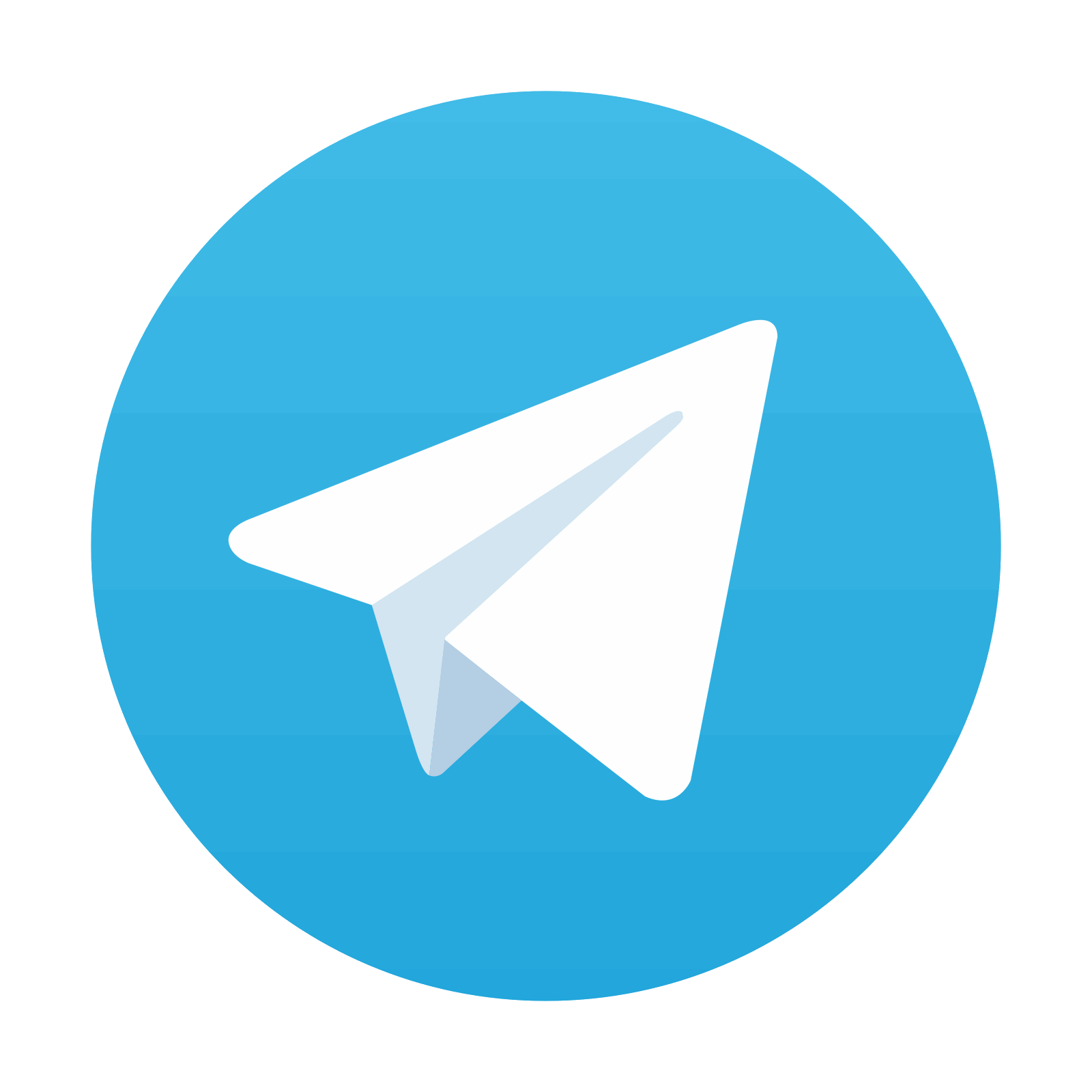
Stay updated, free articles. Join our Telegram channel

Full access? Get Clinical Tree
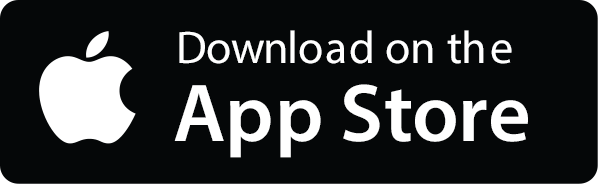
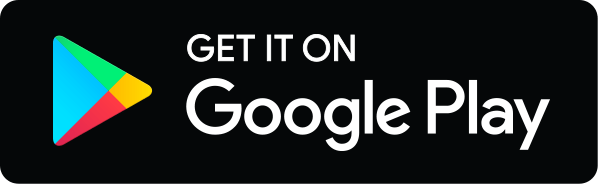