Chapter 14 Coronary Artery Disease Detection
Exercise Stress SPECT
INTRODUCTION
In the United States alone, more than 7.7 million patients are referred yearly for diagnostic cardiac stress testing with radionuclide imaging. Although patients may require pharmacologic stress testing because they are unable to perform adequate physical exercise, dynamic exercise on treadmill or bicycle remains the preferred stress modality. In our nuclear stress laboratory, the percentage of myocardial perfusion studies performed with exercise has remained approximately 50% since 2004. Exercise variables, including maximal workload, duration, hemodynamic response, exercise-induced symptoms, and electrocardiographic (ECG) changes provide invaluable additional information for assessing a patient’s prognosis that are not available with pharmacologic stress. The Duke exercise treadmill score incorporates some of these important exercise variables and allows for risk stratification.1 However, because of the inherent diagnostic limitations of the exercise ECG and subjective symptoms, patients stratified by the Duke Treadmill Score can be further stratified on the basis of (semi)quantitative stress radionuclide myocardial perfusion imaging (MPI).2
PHYSIOLOGY OF EXERCISE STRESS TESTING
Dynamic exercise increases the metabolic demand of the exercising skeletal muscle which can be met only by increasing the blood flow to the exercising muscle. Under conditions of maximal exertion, the cardiac output may increase more than fourfold to meet this need.3 As shown in Figure 14-1, at low levels of exercise, the increase in cardiac output is due to an increase in both stroke volume and heart rate. However, as the intensity of exercise increases, the contribution of the stroke volume to cardiac output reaches a plateau, and the augmentation of cardiac output becomes primarily dependent on the ability to increase the heart rate further. Of note, the inability to appropriately increase the heart rate in response to exercise predicts severity of coronary artery disease (CAD) and mortality.4,5 The increase in cardiac output results in a greater myocardial metabolic demand, inducing coronary vasodilation. A recent positron emission tomography (PET) study in human subjects quantifying the change in myocardial perfusion with exercise demonstrated that myocardial blood flow can increase more than fourfold to meet the metabolic demands of the heart during exercise.6
Metabolic changes occur in association with these hemodynamic adaptations. Specifically, in exercising skeletal muscles, there is a decreasing dependence on fatty acid oxidation and an increasing dependence on glucose oxidation to meet the energy needs until maximum oxygen consumption is achieved. At that point, glucose is the exclusive oxidative substrate. Further increases in the level of exercise above the anaerobic threshold cause the release of lactate from exercising skeletal muscle. Interestingly and in contrast, with increasing exercise, the myocardium continues to oxidize both fatty acids and glucose and also increases the utilization of lactate produced by the exercising skeletal muscle for energy (see Fig. 14-1).
EXERCISE PROTOCOLS
In the United States, most exercise tests are performed using a motorized treadmill and graded Bruce, modified Bruce, or Naughton protocols. In many other countries, the upright bicycle is the preferred exercise equipment. An important aspect of diagnostic exercise protocols is the gradual, linear increase in workload from a very low level to the maximally tolerated workload. This gradual increase in workload is of importance, since the purpose of physical exercise testing is to reproduce symptoms, and one can generally not predict at which workload symptoms will occur. It is therefore important to also evaluate the patient carefully prior to starting a stress test to determine if the patient is appropriate for exercise stress testing (Table 14-1). Exercise protocols are generally terminated because of the onset of symptoms or fatigue, but they may also be terminated because of the development of significant ECG changes or because the patient becomes hypotensive or develops pulmonary edema. Termination of exercise solely because the predicted target heart rate (85% of age-predicted max) was achieved is a commonly made mistake. Many elderly patients, for example, are capable of achieving a considerably higher heart rate and workload at which symptoms may be unmasked.
Table 14-1 Contraindications to Exercise Stress Testing
Absolute Contraindications | Relative Contraindications |
---|---|
Acute myocardial infarction | Aortic stenosis |
Unstable angina | Suspected left main equivalent |
Acute myocarditis or pericarditis | Severe hypertension (>240/130) |
Ongoing ventricular or atrial tachyarrhythmias | Severe outflow tract obstruction |
Second- or third-degree heart block | Left bundle branch block |
Known severe left main disease | |
Decompensated heart failure | |
Acutely ill patients | |
Patients unable to exercise due to neurologic or musculoskeletal limitations |
PREPARATION FOR EXERCISE
Patients should be instructed to wear comfortable clothing and shoes and be in a fasted state on the morning of the exercise test. Moreover, it is prudent to instruct all patients scheduled for stress testing not to consume any caffeine-containing beverages or food on the morning of testing. The reason for this is that it is not uncommon that a patient is not able to perform adequate exercise. If the patient has not consumed caffeine-containing items, it is easy to switch to pharmacologic stress and ensure a diagnostic test result without having to reschedule the patient. On the other hand, a recent study suggested that a small amount of caffeine does not significantly affect the presence and magnitude of perfusion abnormalities. Although it appears prudent to continue to recommend that patients abstain from caffeine usage prior to the test, consumption of a single cup of coffee may not be a valid reason for cancellation of a vasodilator stress test.7
BASIS OF STRESS IMAGING
The conceptual underpinning of radionuclide imaging is the visualization of heterogeneity of regional myocardial blood flow secondary to impaired regional coronary flow reserve downstream of coronary arteries with significant obstructive disease (Fig. 14-2).
In order to visualize such heterogeneity of blood flow reliably, a linear relationship must exist between regional myocardial blood flow and regional myocardial uptake of a radiotracer.8 Although in general this is true for relatively low flow ranges (up to 1 mL/min/g), as is present under resting conditions and resting myocardial ischemia, at the higher flow ranges (>2 mL/min/g) achieved during stress, especially during vasodilator stress, regional myocardial radiotracer uptake may no longer be linear. At higher rates of blood flow, the uptake of many radiotracers presently used in clinical imaging demonstrates a plateau (Fig. 14-3) known as the roll-off phenomenon.
The suboptimal linearity at higher flow ranges is caused by the limited first-pass extraction fraction of many single-photon radiopharmaceuticals used for MPI. Table 14-2 shows the myocardial extraction fractions of commonly used radiotracers. In addition to myocardial extraction fraction, mechanisms involved in the transmembrane transport and myocardial washout and wash-in affect net myocardial uptake of radiopharmaceuticals. Fortunately this roll-off phenomenon does not significantly affect the ability to identify patients with high-degree (>70%) coronary stenoses. However, in patients with relatively mild CAD, the roll-off phenomenon may potentially result in falsely normal images (Fig. 14-4).
Table 14-2 First-Pass Myocardial Extraction Fraction of Various Radiopharmaceuticals
Thallium-201 | 82%–88% |
Technetium (99mTc) sestamibi | 55%–68% |
99mTc tetrofosmin | 54% |
99mTc teboroxime | >90% |
99mTc NOET | 75%–85% |
Thallium (201Tl), a potassium analog, has a relatively high initial extraction fraction and good linearity to flow. After intravenous administration, continuous washout and wash-in occurs in the myocardium, resulting in Tl-201 myocardial redistribution. Technetium (99mTc)-labeled agents, such as sestamibi and tetrofosmin, are lipophilic compounds that have lower first-pass extraction fractions than 201Tl and bind to mitochondrial membranes in a stable manner based on the mitochondrial membrane potential, and demonstrate minimal washout. Consequently the ultimate net retention of 201Tl-labeled and 99mTc-labeled agents in the myocardium is similar.9
INJECTION OF RADIOTRACER
The timing of injection of radiotracer relative to peak exercise and termination of exercise is an important and often not well-appreciated aspect of stress testing. Because the first-pass extraction of the radiotracers is not 100%, myocardial uptake of a radiotracer is not instantaneous at the moment of injection. After injection during stress, the injected bolus reaches the central circulation in about 10 to 15 seconds, and first-pass myocardial extraction takes place. After the initial first transit, recirculation occurs with a rapidly decreasing blood concentration of radiotracer. On average, it takes about 1.5 to 2 minutes for blood radioactivity to decrease to 50% of maximum (Fig. 14-5). This may differ among patients, depending on the level of exercise effort. Furthermore, blood clearance curves are different for various radiotracers (e.g., slightly faster for 99mTc-labeled agents than for 201Tl). The timing of radioisotope injection is based on the fact that it is important to have most of the dose accumulate in the myocardium under conditions in which the heterogeneity of blood flow is maximal, that is, at peak exercise. Termination of exercise at about 1 minute after injection, as is frequently done in clinical practice, may be too early. A substantial amount of radiotracer may still be recirculating at that time. Therefore, it is advisable to encourage patients to continue exercising for at least 2 minutes after injection of radiotracer.
IMAGING PROTOCOLS
Reliable interpretation of stress myocardial perfusion images depends for a large part on optimal quality images. Consistently good quality can be ensured by following image acquisition guidelines published by the American Society of Nuclear Cardiology.10
At present, four image-acquisition protocols are most commonly used: the 1-day or 2-day rest-exercise 99mTc-agent imaging protocol, the exercise-redistribution Tl-201 imaging protocol, and the rest/exercise dual-isotope imaging protocol. Numerous studies have shown that each of these protocols yields equivalent clinical results for detection of CAD and for risk stratification. If many patients referred to an imaging facility are overweight (body mass index > 30 kg/m2), 99mTc-labeled agents and 2-day imaging protocols may be preferred. The most frequently used imaging protocols for 201Tl and 99mTc-labeled agents are schematically shown in Figure 14-6A-D. Technical details of imaging are tabulated in Table 14-3.
Table 14-3 Summary of Typical Acquisition Parameters for SPECT Myocardial Perfusion Imaging with 201Tl and 99mTc-Labeled Imaging Agents
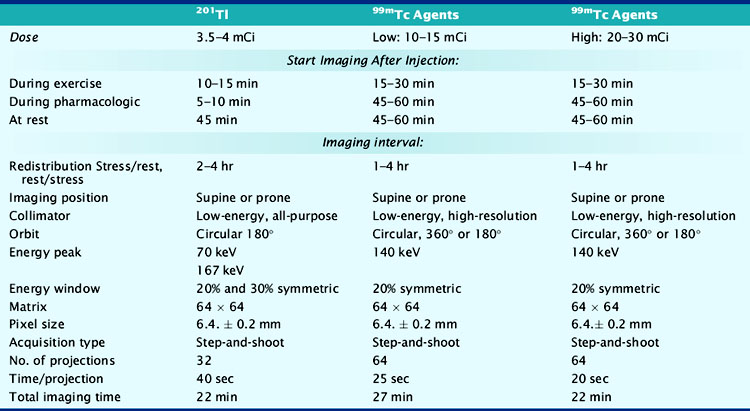
Increasing attention is being paid to the radiation exposure associated with imaging procedures, including myocardial perfusion studies. The exposure and the biological effect of radiation, generally assessed in terms of the effective dose, is dependent not only on the absolute amount of radioactivity that is injected but also the quality of the radiation that is emitted, which is the same for the gamma-photon emitting radionuclides used in single-photon emission computed tomography (SPECT) MPI, the half-life of the agent, and the sensitivities of specific organs to the radiation. Based on these factors, the lowest effective dose is achieved with 99mTc-labeled agents used in a 1-day stress/rest protocol, whereas the highest effective dose is achieved with a dual-isotope protocol (Table 14-4).11 In patients with low to intermediate pretest likelihood of CAD, normal exercise parameters, and normal MPI, it may be possible to perform only stress imaging, thereby reducing the radiation exposure.
Table 14-4 Estimated Effective Doses for Various Myocardial Perfusion Imaging Protocols
Protocol | Effective Dose (mSv) |
---|---|
Thallium stress/redistribution | 22.0 |
One-day stress/rest 99mTc sestamibi | 11.3 |
Two-day stress/rest 99mTc sestamibi | 15.7 |
One-day stress/rest 99mTc tetrofosmin | 9.3 |
Two-day stress/rest 99mTc tetrofosmin | 12.8 |
Based on data from Einstein AJ, Moser KW, Thompson RC, et al: Radiation dose to patients from cardiac diagnostic imaging. Circulation 116:1290–1305, 2007.
DIAGNOSTIC VALUE OF EXERCISE SPECT IMAGING
Because of the proven prognostic value of stress MPI, it is unlikely that angiographic correlation and sensitivity and specificity will continue to be assessed in large numbers of patients using state-of-the-art technology, other than for local quality assurance purposes or to evaluate new technical advances such as attenuation-correction devices. The sensitivity, specificity, and normalcy values for exercise SPECT MPI have been evaluated in multiple studies and confirm the diagnostic power of this modality.12–24 Based on a meta-analysis of 79 studies that included a total of 8964 patients, the sensitivity, specificity, and normalcy rate of SPECT MPI are 86%, 74%, and 89%, respectively.25,26 Figure 14-7 shows representative data for the diagnostic yield for identifying disease in individual coronary arteries.27 Sensitivity for recognizing disease in the left anterior descending coronary artery is higher than that for disease in the right coronary artery or left circumflex coronary artery, with no significant difference in accuracy. The relatively low specificity of SPECT imaging suggests that artifacts due to attenuation and motion are not always recognized. Referral bias has been proposed as another potential explanation for the limited specificity of SPECT imaging.
Through the years, it has also become clear that complete agreement with coronary angiography is an elusive goal, because stress perfusion imaging and coronary angiography evaluate two different aspects of CAD. Whereas contrast coronary angiography visualizes coronary anatomy and the presence or absence of regional coronary luminal narrowings, radionuclide stress MPI provides noninvasive information about the pathophysiologic consequences of coronary atherosclerosis. Specifically, patients with apparently significant angiographic CAD but normal exercise myocardial perfusion images nevertheless have a favorable prognosis.28 In contrast, patients with relatively mild angiographic CAD but markedly abnormal SPECT images have a less favorable outcome. One study29 demonstrated that in patients with apparently “false-positive” 201Tl images and “normal” epicardial coronary arteries, coronary blood flow response to acetylcholine infusion was blunted, indicating endothelial dysfunction (Fig. 14-8). This suggests that “false-positive” images probably were true positives. A better measure of the true specificity of stress MPI is the assessment of normalcy rate in subjects with low (<3%) likelihood of CAD. In our own laboratory, the normalcy rate of 99mTc sestamibi SPECT was 99% using quantitative analysis of exercise myocardial perfusion SPECT images acquired in 40 normal subjects.
ECG-GATED SPECT
Evaluating the left ventricular ejection fraction (LVEF) is important in any cardiac patient. Currently, more than 80% of all SPECT studies are acquired with ECG gating. Gated SPECT provides a means of assessing postexercise resting LVEF, as well as regional wall motion and wall thickening. The routine assessment of LVEF by ECG-gated SPECT has added an important dimension to the overall assessment of patients referred for exercise testing and MPI.30 Although the appearance of motion and the change in count density on cine display of ECG-gated SPECT myocardial perfusion studies is in fact artifactual and caused by improved count recovery during the cardiac cycle due to diminishing partial volume effect, a linear relationship exists with myocardial thickening.31
Assessment of LVEF, either normal or abnormal, in a patient with exercise-induced ischemia has important clinical and management consequences.32,33 Furthermore, it is not rare that a patient is diagnosed as having a previously unknown cardiomyopathy on the basis of the information from a gated SPECT. In some patients, postexercise LVEF may be significantly lower than LVEF on rest SPECT imaging.34 This may indicate postexercise ischemic stunning, which is a marker signifying severe CAD, although the specificity of this finding reflecting stunning may be limited in the setting of a large reversible defect.35
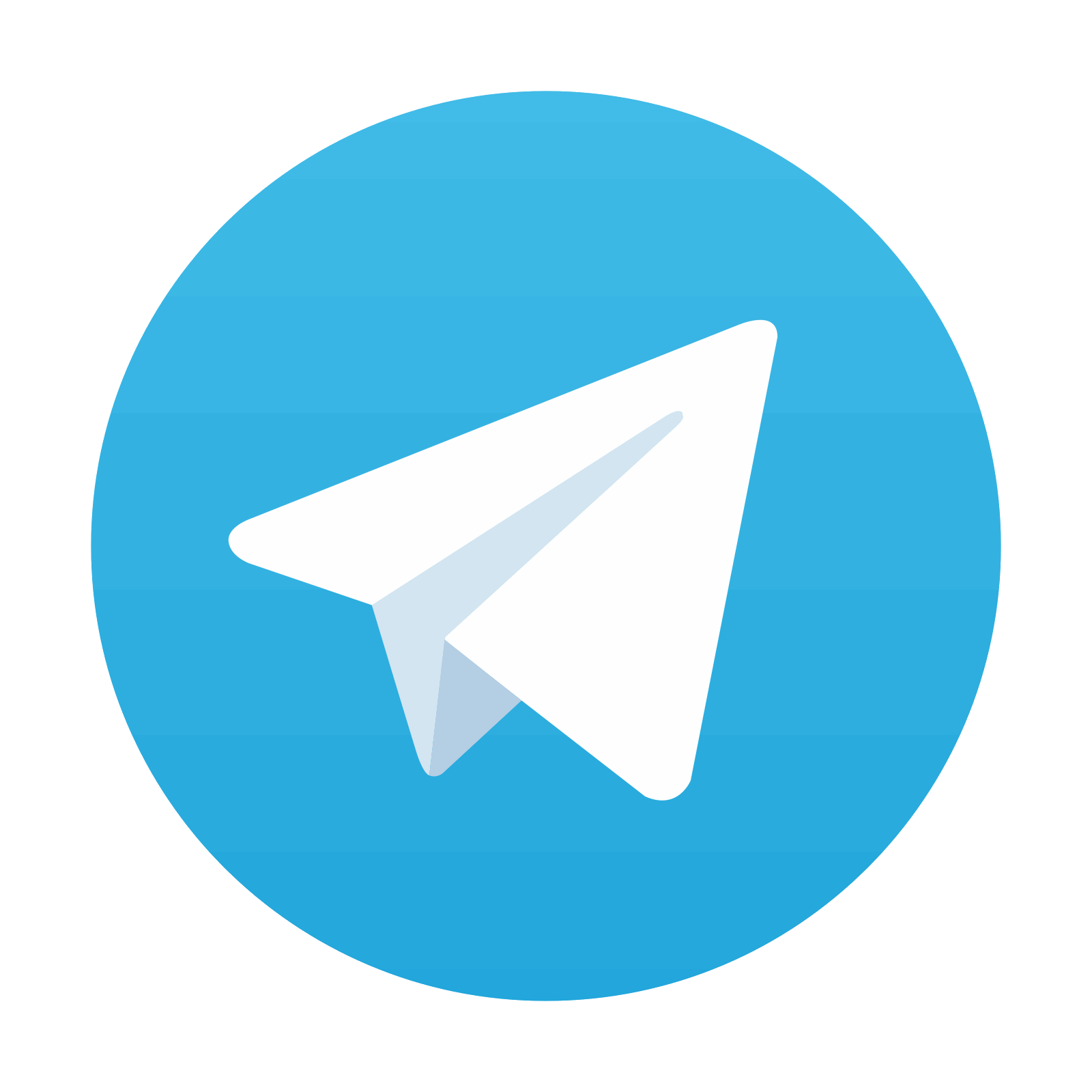
Stay updated, free articles. Join our Telegram channel

Full access? Get Clinical Tree
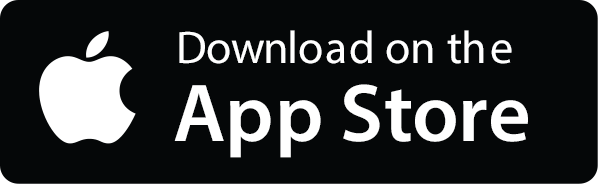
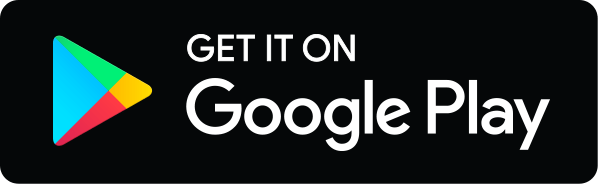