Coronary Artery Disease and Acute Myocardial Infarction
Echocardiography is the most commonly used and most practical imaging technique for the evaluation of patients who have coronary artery disease, chest pain, or acute myocardial infarction. Knowledge of global and regional systolic function and diastolic function is helpful in establishing the diagnosis, management strategy, and prognosis of patients who have coronary artery disease or who have had an acute myocardial infarction. Myocardial perfusion and contractility become abnormal immediately after the onset of ischemia. The resulting perfusion defect and regional wall motion abnormality (RWMA) can be detected readily with echocardiography, even before other ischemic manifestations. The treatment of acute myocardial infarction with thrombolysis or percutaneous coronary intervention (or both) has changed outcome and natural history of myocardial infarction. Without prompt diagnosis and early surgical intervention, mechanical complications of acute myocardial infarction are often fatal. Echocardiography, including transesophageal echocardiography (TEE), should be able to identify most, if not all, mechanical complications of myocardial infarction. Some patients who have an acute myocardial infarction have no abnormalities in the epicardial coronary arteries (1). Echocardiography can still identify structural and functional changes associated with acute myocardial infarction in these patients. Exercise or pharmacologic stress echocardiography is valuable in predicting myocardial viability and prognosis as well as in detecting coronary artery disease (this is discussed in Chapter 11). Therefore, echocardiography has an important role, from the diagnosis of coronary artery disease, early detection of acute myocardial infarction (even in the absence of typical electrocardiographic [ECG] evidence), evaluation of RWMAs and viability after reperfusion therapy, detection of postinfarction mechanical and functional complications, evaluation of unstable hemodynamics, and assessment of myocardial viability to prognostic risk stratification.
Evaluation of Myocardial Wall Motion
The immediate manifestation of myocardial ischemia is a decrease in or cessation of myocardial contractility (systolic thickening), even before the occurrence of ST-segment changes or the development of symptoms. Ischemic myocardium may continue to demonstrate some degree of passive forward motion because of the pulling action of adjacent nonischemic muscle, but the contractility (systolic thickening) of the ischemic myocardial segments is decreased (hypokinesis) or absent (akinesis). Normally, left ventricular (LV) free wall thickness increases more than 40% during systole. In normal subjects, the percentage of thickening of the ventricular septum is somewhat less than that of the free wall of the LV. Hypokinesis is defined as systolic wall thickening less than 30%, and akinesis is defined as wall thickening less than 10%. Dyskinesis is defined as a myocardial segment moving outward during systole, usually in association with systolic wall thinning.
With multiple tomographic imaging planes, two-dimensional (2D) echocardiography allows visualization of all LV wall segments. For purposes of regional wall motion analysis, the LV is divided into several segments. The American Society of Echocardiography has recommended a 16-segment model (2) (see Fig. 7-14). Optionally, the apical tip is added as a 17th segment. Each segment is assigned a score on the basis of its contractility as assessed visually: normal = 1, hypokinesis = 2, akinesis = 3, dyskinesis = 4, and aneurysm = 5. On the basis of this wall motion analysis scheme, a wall motion score index (WMSI) is calculated to semiquantitate the extent of regional wall motion abnormalities:

A normally contracting LV has a WMSI of 1 (each of the 16 segments receives a wall motion score of 1; hence, the total score is 16 and WMSI is 16/16 = 1). The larger the infarct the higher the WMSI because wall motion abnormalities become more severe.
What does the WMSI indicate? Because the echocardiographic analysis of wall motion abnormality is subjective and the reduction of systolic myocardial thickening is not proportional to the incremental amount of infarcted or ischemic myocardial tissue (3), the correlation of the WMSI with the actual size of the myocardial infarct or the perfusion defect may not be good in the case of acute myocardial infarction. When a 2D echocardiographic examination was performed simultaneously with an injection of sestamibi in patients with acute myocardial infarction with ST-segment elevation on the ECG, the overall correlation between the WMSI and the perfusion defect was good (4). Patients with WMSI greater than 1.7 had a perfusion defect larger than 20%. The correlation was better in patients with an anterior wall myocardial infarction than in those with an inferior or lateral wall myocardial infarction with a smaller infarct size. However, it is possible to have relatively normal myocardial contractility when there is a myocardial perfusion defect. Also, the reverse is true, depending on the clinical situation. Without a previous ischemic insult, a small subendocardial perfusion can be present when no visible contractility abnormality is evident. However, the myocardium may remain akinetic for a period of time after coronary reperfusion. Therefore, knowledge of both myocardial contractility and perfusion is vital for the management of the subset of patients with coronary artery disease. Another interesting subset of patients with acute myocardial infarction is the group with normal coronary arteries, for example, patients with apical ballooning syndrome, subarachnoid hemorrhage, or pheochromocytoma or those who have had electroconvulsive therapy (1,5,6,7). In these situations, marked wall motion abnormalities are present acutely but perfusion is normal. These wall motion abnormalities usually resolve in 5 to 10 days.
Technical Caveats
A reliable regional wall motion analysis is among the most challenging tasks in echocardiography. All available windows and tomographic planes should be used to visualize all the LV segments (Fig. 10-1 A). Apical short- and long-axis views are especially useful in evaluating the apical third of the LV (Fig. 10-1 B). Continuous scanning from the apical four-chamber to the apical long-axis to the apical two-chamber view allows complete visualization of all LV segments. In patients who have chronic obstructive pulmonary disease or who are obese, a lower frequency (2.0–2.5 MHz) transducer should be used to optimize the definition of the endocardium, and the subcostal window may provide adequate visualization of the LV segments. A new imaging method that uses the principle of harmonic resonance (native harmonic imaging) can improve visualization of the endocardium (Fig. 10-2). In patients with a good apical window, the use of higher frequency transducers, with adjustment of the focal zone to the near region, may enhance the definition of the apical endocardium, help delineate apical wall motion abnormalities, and differentiate thrombus from apical trabeculation.
The assessment of regional wall motion on echocardiography is limited when visualization of the LV endocardium is not adequate. Several new modalities may enhance the ability to analyze regional wall motion. For example, the intravenous administration of contrast agent may enhance endocardial definition (see Chapter 6), and colorization of 2D echocardiographic images may improve visualization of the endocardial border. Newer ultrasound units hold promise in this regard, with more advanced technology to enhance visualization of the endocardium. Second harmonic imaging, initially created for contrast echocardiography, improves visualization of cardiac structures as well as endocardial definition.
Evaluation of Chest Pain Syndrome
Not all patients with prolonged chest pain resulting from myocardial ischemia or infarction present with typical ECG changes. More than 50% of patients with myocardial infarction have nonspecific findings on the initial ECG. Rapid assay of isoenzymes of creatine kinase (CK-MB) is reliable in detecting myocardial infarction within the first 6 hours after the onset of chest pain (8; however, the sensitivity of CK-MB isoenzymes assays may not be satisfactory if the assay is performed within 4 hours after the onset of chest pain. Troponin T and troponin I are more sensitive markers of necrotic myocardial tissue (9). More recently, plasma myeloperoxidase a leukocyte enzyme, has been found to predict the risk of myocardial infarction in patients presenting to an emergency department with chest pain (10). The level of myeloperoxidase is increased in culprit lesions that
have fissured or ruptured in patients with sudden death from cardiac causes. Also, an increased level of myeloperoxidase independently predicted major cardiac events in the ensuing 1-month and 6-month periods.
have fissured or ruptured in patients with sudden death from cardiac causes. Also, an increased level of myeloperoxidase independently predicted major cardiac events in the ensuing 1-month and 6-month periods.
![]() Figure 10-3 A: Top, Color M-mode image, with yellow indicating systolic shortening (s) and blue-white indicating diastolic lengthening (d) in strain rate imaging. The time point of transition from shortening to lengthening (contraction to relaxation) is indicated by the solid black line. Middle, Strain rate tracing. *, Systolic wave; solid arrow, late diastolic wave; open arrow, early diastolic wave. Bottom, Electrocardiographic trace. An example of the time to onset of relaxation (TR) (electrocardiogram R wave to the transition point) is illustrated at the bottom of the strain rate tracing. The dotted lines (top) separate the septum into apical, mid, and basal segments. B: Strain rate color M-mode image illustrating a decrease in TR from baseline to peak stress in the nonischemic basal segment (black arrow) and minimal TR change in the ischemic apical segment (white arrow). (From Abraham et al [16]. Used with permission.) |
The main advantages of echocardiography are that it can be performed in the emergency department and its findings are available immediately. As mentioned, myocardial contractility diminishes or ceases immediately after ischemia or infarction and is manifested as an RWMA that, in most patients, is readily identified with 2D echocardiography. Therefore, the use of RWMAs as a marker of myocardial infarction in patients with prolonged chest pain and nondiagnostic ECG findings is attractive. The absence of LV wall motion abnormalities usually excludes the presence of myocardial ischemia. Also, although the presence of RWMAs has a high sensitivity for detecting myocardial infarction, the positive predictive value is about 30% because RWMAs are not specific for acute myocardial infarction and some patients have unstable angina without myocardial damage. However, echocardiography may not be helpful or cost-effective if patients have a low to intermediate risk of acute myocardial infarction. Occasionally, echocardiography may be useful in detecting a potentially fatal cause of chest pain syndrome, such as pulmonary embolism, aortic dissection, or cardiac tamponade. The incidence of these findings is small, but anticoagulation or thrombolytic therapy in a subset of these patients may have a disastrous clinical outcome. The approach outlined here requires that the echocardiography examination and interpretation in the emergency department be prompt. Images can be interpreted at a distant site with the use of digital echocardiography, but having appropriately trained personnel available to perform echocardiography in the emergency department is a challenge.
A 2D echocardiographic analysis of RWMAs is helpful diagnostically and clinically even in patients with classic chest pain and ST-segment elevation on the ECG. The amount of myocardium at risk can be estimated by calculating the WMSI. A WMSI greater than 1.7 usually suggests a perfusion defect larger than 20% and increased complications unless the wall motion abnormalities are reversed with reperfusion therapy. Several days after reperfusion therapy, regional wall motion analysis is useful in assessing functional recovery, although myocardial contrast perfusion
echocardiography can predict functional recovery soon after reperfusion therapy (11,12). A biphasic response during exercise echocardiography early after myocardial infarction also predicts myocardial viability (13).
echocardiography can predict functional recovery soon after reperfusion therapy (11,12). A biphasic response during exercise echocardiography early after myocardial infarction also predicts myocardial viability (13).
The amount of myocardium at risk from the affected artery also may determine which patients receive the greatest benefit from acute interventional therapy. Patients with a large amount of myocardium at risk (usually an anterior wall myocardial infarction) will derive more benefit from reperfusion therapy than those with a small amount of myocardium at risk. When a patient presents with an ST-segment elevation myocardial infarction, the affected myocardium is akinetic or dyskinetic. After the myocardium has been reperfused successfully within an appropriate time (usually within 4 hours), it becomes more contractile on subsequent 2D echocardiography studies. Acute myocardial infarction may be aborted in a subset of patients if the ischemic duration is short. Serial echocardiography studies have demonstrated that the improvement in regional myocardial contractility is evident within 24 to 48 hours and that the improvement continues for several days to months. Therefore, follow-up 2D echocardiographic imaging is useful in detecting reperfused myocardial segments and infarct expansion.
Persistent akinesis does not always indicate failed reperfusion. When the myocardium remains akinetic while being viable, low-dose dobutamine or contrast echocardiography may be helpful in demonstrating its viability (see Chapter 11). Enlargement or remodeling of the LV, one of the strongest predictors of a cardiac event after myocardial infarction (14, is readily assessed with serial 2D echocardiography and can be predicted by the lack of myocardial perfusion.
Strain measurements have been used to identify acutely ischemic myocardium. In a study of patients undergoing elective coronary angioplasty, systolic strain was reduced and postsystolic strain was increased but delayed (15). Relaxation of ischemic myocardial segments is impaired and as a consequence, the physiologic early diastolic thinning and lengthening are replaced by ongoing postsystolic thickening and shortening. Therefore, it may be possible that the abnormal regional deformation (i.e., reduction of systolic strain) or strain rate can be used as a marker of acute ischemia if the change in strain or local deformation can be visualized or identified readily (16) (Fig. 10-3).
Acute Myocardial Infarction
In many institutions world-wide, primary percutaneous coronary intervention (PCI) is the treatment of choice for acute myocardial infarction and mortality after acute myocardial infarction has decreased significantly. The role of echocardiography has evolved as the management strategy of acute myocardial infarction has changed. Its current role can be classified as follows: 1) diagnosis and exclusion of acute myocardial infarction in patients with prolonged chest pain and nondiagnostic ECG findings, 2) estimation of the amount of myocardium at risk and final infarct size after reperfusion therapy, 3) evaluation of unstable hemodynamics, 4) detection of infarct complications, 5) evaluation of myocardial viability, and 6) risk stratification. Therefore, at various stages of acute myocardial infarction, echocardiography is important in providing anatomical, functional, and hemodynamic information.
Mechanical Complications and Cardiogenic Shock
Because patients who have cardiogenic shock after myocardial infarction have a poor prognosis unless the cause is reversible, it is of paramount clinical importance to identify promptly the underlying cause so that proper treatment can be given. In a study of an international registry from 19 medical centers, the cause of cardiogenic shock after myocardial infarction in 251 patients was severe LV failure in 85%, mechanical complications in 8%, right ventricular (RV) infarct in 2%, and other comorbid conditions in 5% (17). Two-dimensional and Doppler echocardiography with color flow imaging are useful for promptly identifying the cause in these patients, especially in checking for mechanical complications. Echocardiography performed soon after acute myocardial infarction and shock showed that the severity of mitral regurgitation and LV ejection fraction (LVEF) were the only independent predictors of survival (18). In addition, TEE should be performed promptly in patients in whom precordial echocardiography may not be possible or if images obtained in the intensive care unit are suboptimal (19). The presence of normal systolic function in a critically ill or hemodynamically unstable patient should immediately lead to suspicion of a mechanical complication. Acute and chronic complications of myocardial infarction are listed in Table 10-1.
Left Ventricular Failure and Remodeling
Compared with 10 years ago in our coronary care unit, cardiogenic shock or heart failure due to LV systolic dysfunction is now less common after the first myocardial infarction, thanks to the tremendous clinical success with thrombolytic therapy and PCI. However, if cardiogenic shock develops after myocardial infarction, the mortality rate remains extremely high. Urgent coronary revascularization improves the outcome (20), but the mortality rate is still 40% to 50%. A more common clinical scenario is congestive heart failure and poor outcome due to progressive LV remodeling that is stimulated by the initial mechanical damage to the LV and subsequent neurohormonal modulation. With LV remodeling, the LV becomes larger and more spherical (i.e., more dilatation along the short axis of the LV), LVEF decreases, and mitral leaflets are displaced more apically, allowing increasing degrees of mitral regurgitation: all these features result in a worsening of heart failure and death. Clinical trials have demonstrated that β-blockade, angiotensin-converting enzyme (ACE) inhibitor, and biventricular pacing therapy ameliorate or reverse LV remodeling (21, 22, 23, 24). Ongoing trials are addressing the importance of surgical revascularization, surgical ventricular resection, passive constraint device, and mitral valve procedure in this patient population. Echocardiography is essential in managing these patients, and it assists clinical trials in LV remodeling by providing regional wall motion analysis and detailed information about LV size and volume, myocardial viability, LV filling pressures, severity of mitral regurgitation, and pulmonary artery systolic pressure.
Table 10-1 Complications of myocardial infarction | ||
---|---|---|
|
Right Ventricular Infarct
The RV frequently is involved in acute myocardial infarction; however, a hemodynamically significant RV infarct
is infrequent and almost always associated with inferior wall myocardial infarction (25). However, once a patient develops cardiogenic shock due to RV infarct, mortality is as high as for cardiogenic shock due to LV infarct (26). Revascularization improves survival. Patients who have an RV infarct present with increased jugular venous pressure but clear lung fields. They may become hypotensive after the administration of nitroglycerin or develop shock that requires inotropic support and the administration of fluids. Echocardiographically, the RV is dilated and hypokinetic to akinetic (Fig. 10-4). The apical portion of the RV is supplied by the left anterior descending coronary artery
and may contract normally while the basal to mid-RV free walls become affected in an RV infarct. The right atrium (RA) is also dilated, and tricuspid regurgitation becomes significant as a result of dilatation of the tricuspid anulus. Because RV systolic pressure is not increased, peak tricuspid regurgitation velocity is not high, usually less than 2 m/s. Tissue Doppler imaging of the tricuspid valve anulus may be helpful in identifying depressed RV function in patients with inferior wall myocardial infarction. In patients with a patent foramen ovale (PFO)an RV infarct creates an optimal clinical setting for a clinically significant right-to-left shunt through the PFO because of abnormal RV compliance and markedly increased RA pressure. If a patient presents with hypoxemia after inferior wall myocardial infarction, an RV infarct and right-to-left shunt through a PFO should be strongly considered. This diagnosis can be confirmed with contrast echocardiography (peripheral venous injection of agitated saline). Following opacification of the RA, the contrast medium enters the LA through the PFO. This situation is best assessed with TEE (Fig. 10-4). In this clinical setting, the PFO and shunt can be closed with a closure device.
is infrequent and almost always associated with inferior wall myocardial infarction (25). However, once a patient develops cardiogenic shock due to RV infarct, mortality is as high as for cardiogenic shock due to LV infarct (26). Revascularization improves survival. Patients who have an RV infarct present with increased jugular venous pressure but clear lung fields. They may become hypotensive after the administration of nitroglycerin or develop shock that requires inotropic support and the administration of fluids. Echocardiographically, the RV is dilated and hypokinetic to akinetic (Fig. 10-4). The apical portion of the RV is supplied by the left anterior descending coronary artery
and may contract normally while the basal to mid-RV free walls become affected in an RV infarct. The right atrium (RA) is also dilated, and tricuspid regurgitation becomes significant as a result of dilatation of the tricuspid anulus. Because RV systolic pressure is not increased, peak tricuspid regurgitation velocity is not high, usually less than 2 m/s. Tissue Doppler imaging of the tricuspid valve anulus may be helpful in identifying depressed RV function in patients with inferior wall myocardial infarction. In patients with a patent foramen ovale (PFO)an RV infarct creates an optimal clinical setting for a clinically significant right-to-left shunt through the PFO because of abnormal RV compliance and markedly increased RA pressure. If a patient presents with hypoxemia after inferior wall myocardial infarction, an RV infarct and right-to-left shunt through a PFO should be strongly considered. This diagnosis can be confirmed with contrast echocardiography (peripheral venous injection of agitated saline). Following opacification of the RA, the contrast medium enters the LA through the PFO. This situation is best assessed with TEE (Fig. 10-4). In this clinical setting, the PFO and shunt can be closed with a closure device.
Free Wall Rupture and Pseudoaneurysm
Cardiac free wall rupture usually is a fatal complication of acute myocardial infarction (Fig. 10-5 A) that occurs in about 1% of all patients with myocardial infarction. It accounts for up to 7% of all infarct-related deaths. Typically, the rupture produces a sudden hemodynamic collapse due to cardiac tamponade (from hemopericardium) and electromechanical dissociation. Most ruptures occur within the first week after the infarction (median time, 4 days), and they are more common in women and elderly patients. Patients with rupture have less severe coronary artery disease and usually have a small myocardial infarction. Another clinical situation that potentially enhances cardiac rupture is the use of thrombolytic therapy (usually more than 10 hours after the onset of chest pain), presumably because of a hemorrhagic infarct.
Although patients with infarct expansion are at higher risk for cardiac rupture, no specific echocardiographic features have been found to predict this highly fatal complication. Echocardiographic diagnosis of cardiac rupture depends on a high degree of clinical acumen because a subset of patients may present subacutely with syncope, hypotension, recurrent chest pain, or emesis (or some combination of these) (27). A meticulous search by the echocardiographer for the site of rupture is mandatory if a region of thin myocardium or a small amount of pericardial effusion is present, particularly if a loculated effusion or clot is detected (Fig. 10-5 B). Detection of a free wall rupture in these patients allows surgical repair, with a survival rate greater than 50%.
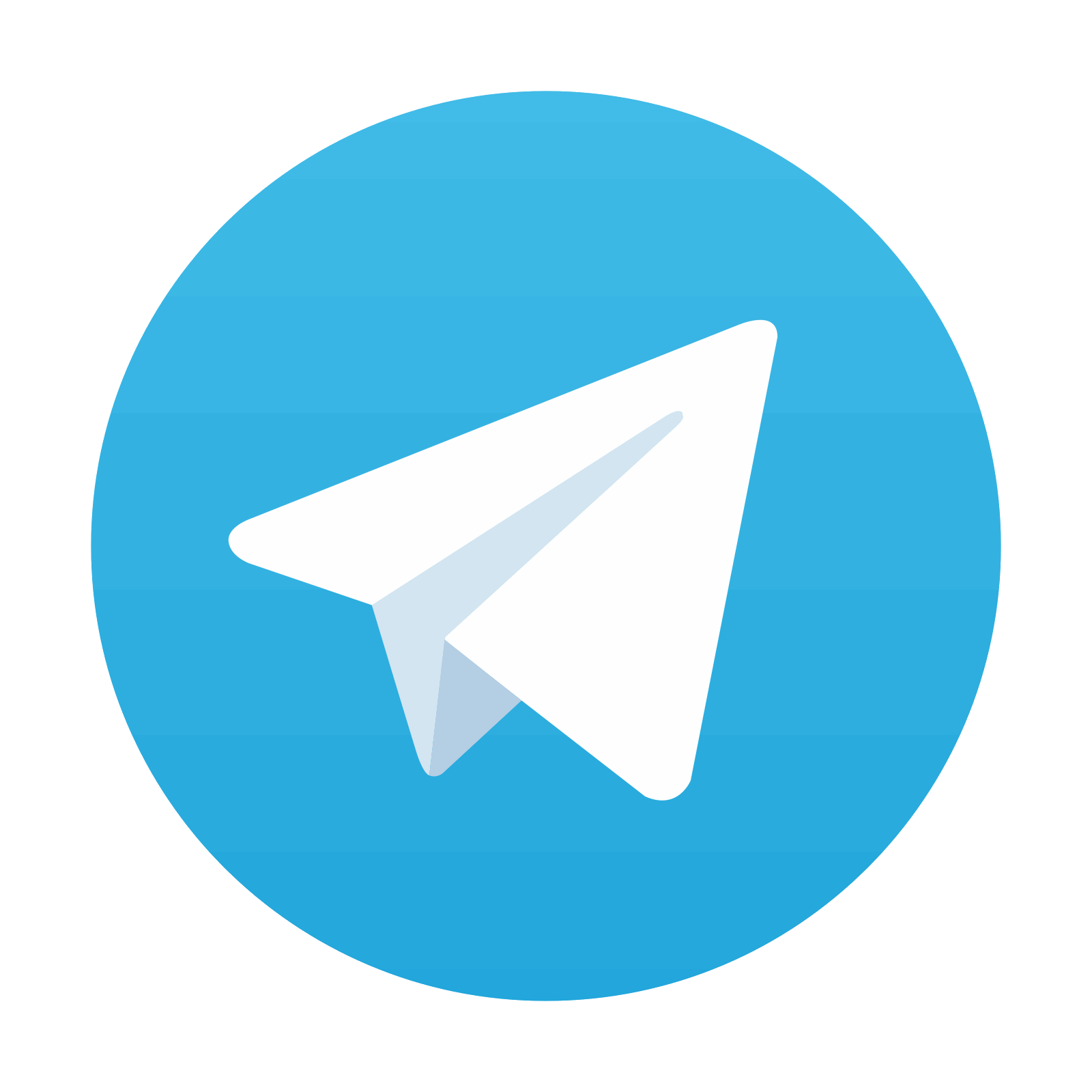
Stay updated, free articles. Join our Telegram channel

Full access? Get Clinical Tree
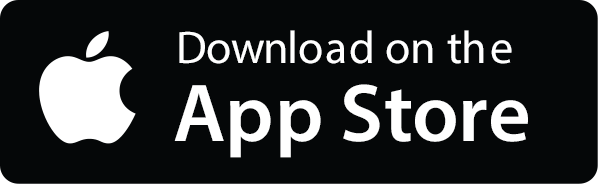
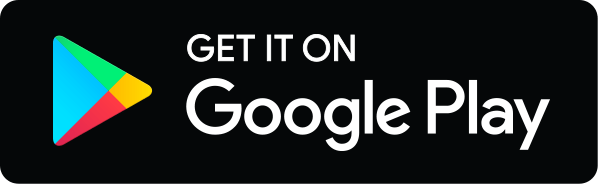