The diagnosis of cardiac amyloidosis (CA) often necessitates invasive myocardial biopsy. We sought to evaluate whether late gadolinium enhancement (LGE) of the atrial myocardium by cardiac magnetic resonance imaging was associated with impaired left atrial (LA) function and whether the extent of LA LGE may enhance diagnostic differentiation of CA from other cardiomyopathies. Twenty-two patients with biopsy-proven CA, 37 with systemic hypertension (SH), and 22 with nonischemic dilated cardiomyopathy (NIDC) underwent cardiac magnetic resonance imaging and echocardiographic evaluation. Patients with CA had greater minimal LA volume (57 ± 53 vs 24 ± 18 in SH and 19 ± 25% in NIDC, p = 0.003), and significantly lower total LA emptying function (19 ± 14 vs 40 ± 14 in SH and 33 ± 20% in NIDC, p = 0.0006). The mean proportion of atrial enhancement (LGE LA %) was significantly greater in patients with CA than with SH and NIDC (59 ± 36% vs 7.4 ± 2.1 and 2.9 ± 9.0%, p <0.0001, respectively). There was also a strong inverse correlation between both active and total atrial emptying ( r = −0.69, p = 0.001; r = −0.67, p = 0.01, respectively) with LGE LA % for patients with CA. In multivariate regression analysis, LGE LA % was the strongest adjusted predictor for CA diagnosis. Using receiver operating characteristic analysis, LGE LA % ≥33% produced the greatest diagnostic utility for CA (sensitivity 76%, specificity 94%). Patients with CA may have extensive LGE of the LA myocardium, which is associated with marked reduction in LA emptying function. The extent of LA LGE was highly predictive for the diagnosis of CA.
Recently, there has been considerable interest in noninvasive diagnosis of cardiac amyloidosis (CA) using novel cardiac imaging modalities, such as contrast-enhanced cardiac magnetic resonance imaging (CMR). CMR may reveal a characteristic pattern of left ventricular subendocardial late gadolinium enhancement (LGE) that reflects the transmural histologic distribution of amyloid protein within the ventricular myocardium. Previous studies of CMR in patients with histologically confirmed amyloidosis have revealed suboptimal nulling despite use of multiple inversion times (because of gadolinium uptake from the blood pool) and atrial enhancement. From a prognostic standpoint, the presence of LGE and hypointense signal on T2-weighted images may serve as important prognostic risk factors for mortality in amyloid patients. Amyloid protein deposition may also occur within the atrial endocardium and has been associated with adverse pathophysiologic consequences, including both atrial failure and thromboembolism. The extent of LA enlargement, a surrogate marker for diastolic dysfunction, has been implicated as an independent predictor for long-term mortality. In this study, we sought to determine whether LGE of the LA myocardium in CA is associated with impaired LA emptying function. We also evaluated whether LGE extent within the left atrium may enhance diagnostic differentiation of CA from other cardiomyopathies.
Methods
This was a single-center study performed at our medical center. Twenty-two patients with endomyocardial biopsy-proven cardiac amyloidosis (CA) were recruited for this study. No patient had undergone any amyloid-specific therapy before either echocardiographic or CMR evaluation. One patient with CA was excluded because of claustrophobia during CMR. Two additional groups of 37 patients with systemic hypertension (SH) and 22 patients with nonischemic dilated cardiomyopathy (NIDC), who were clinically referred for CMR, were included as controls. Exclusion criteria were the presence of any clinical history of myocardial infarction and/or evidence of significant valvular heart disease. The Institutional Ethical Review Board at Brigham and Women’s Hospital approved the study.
All CMR examinations were performed with a 1.5-T scanner (Signa CV/i; General Electric, Milwaukee, Wisconsin) with the patient in a supine position and a 4- or 8-element phased-array coil placed over the chest. Images were acquired during breath-holds with electrocardiographic gating. We used a segmented k-space steady-state free-precession (SSFP) sequence (repetition time 3.4 ms; echo time 1.2 ms; in-plane spatial resolution between 1.5 to 1.8 mm and 1.8 to 2.1 mm, depending on the field of view) for cine imaging in parallel short-axis (contiguous slices of 8-mm thickness covering the base to apex) and 3 radial long-axis views (60° apart) of the left ventricle. Temporal resolution of cine SSFP images were approximately 46 ms. With a previously described inversion recovery pulse sequence (repetition time 4.8 ms; echo time 1.3 ms; in-plane spatial resolution between 1.5 to 1.8 mm and 1.8 to 2.1 mm), LGE images at matching cine image slice locations and slice thickness (8 mm) were acquired 10 to 15 minutes after intravenous gadolinium–diethylene triamine pentaacetic acid administration (0.15 to 0.20 mmol/kg; Magnevist; Berlex Pharmaceuticals, Wayne, New Jersey). We optimized the inversion time (250 to 350 ms) to null the normal myocardium gauged by obtaining signal intensity <10 in the basal ventricular septum. LGE images were obtained with views per segment (range 8 to 24 lines) and trigger delay (350 to 550 ms) adjusted according to the patient’s heart rate to maximize image quality. LGE characteristic for amyloidosis was defined as circumferential subendocardial enhancement. Focal areas of LGE (subepicardial, subendocardial, and midwall) were excluded.
Echocardiography with tissue Doppler was performed in patients with CA. Transmitral flow profiles, including peak early diastolic flow velocity (E), late diastolic flow velocity (A), and mitral E-wave deceleration time were assessed. Pulse-wave tissue Doppler imaging (TDI) was performed using spectral pulse-wave Doppler signal filters and minimum optimal gain. In apical views, a pulse-wave Doppler sample volume was placed at the level of the mitral annulus over the septal and lateral borders. Pulse-wave TDI results were characterized by a myocardial systolic wave, an early diastolic wave (E′), and an atrial contraction diastolic wave (A′). The pulse-wave TDI tracings were recorded over 5 cardiac cycles at a sweep speed of 100 mm/s and used for off-line calculations. The average E′ of the septal and lateral mitral annuluses was chosen to estimate LV diastolic function.
Cine SSFP and LGE imaging were performed in matching 3-radial planes to assess LA function and segmental infiltration of the LA myocardium. Tracing of the LA volumes were performed by a single experienced reader (KS) blinded to the patient’s name, underlying group diagnosis, and results from other examinations. LA volumes were calculated by the validated biplane area–length method and were assessed at each of the 3 diastolic time phases (end-ventricular systole, preatrial contraction, and postatrial contraction). End-ventricular systole, preatrial contraction, and postatrial contraction were marked by aortic valve closure, second diastolic opening of mitral valve leaflets, and mitral valve closure, respectively. At each time phase, LA volume was calculated by the biplane area–length method as follows: LAvolume(ml)=(8/3π)×A2C×A4C/L
LA volume ( ml ) = ( 8 / 3 π ) × A 2 C × A 4 C / L
, where A2C and A4C were the LA areas on the 2-chamber and 4-chamber views, respectively, and L was the shorter long-axis length of the LA from either the 2-chamber or the 4-chamber views ( Figure 1 ). The LA volumes quantified at end-ventricular systole, preatrial contraction, and postatrial contraction, therefore corresponded to maximal LA volume (LAV max ), preatrial contraction LA volume (LAV ac ), and minimal LA volume (LAV min ), respectively. Consistent with the recommendation of published reports, all LA volumes were normalized to the patient’s body surface area.
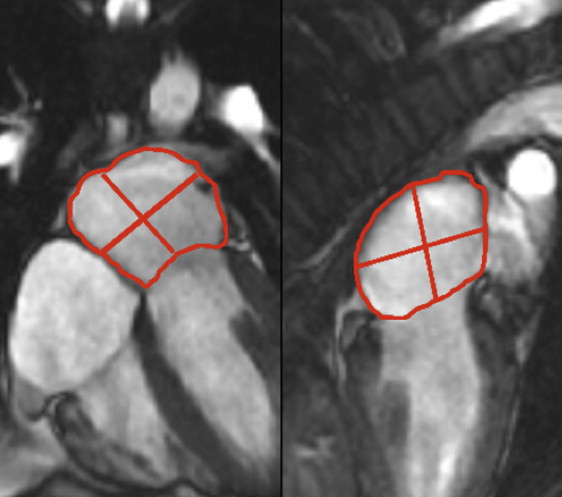
LA emptying functions were derived from the percent reduction in LA volumes between the varying diastolic time points outlined previously. LA total, passive, and active emptying functions (LAEF Total , LAEF Passive , and LAEF Contractile ) were calculated according to the following formulas, respectively:
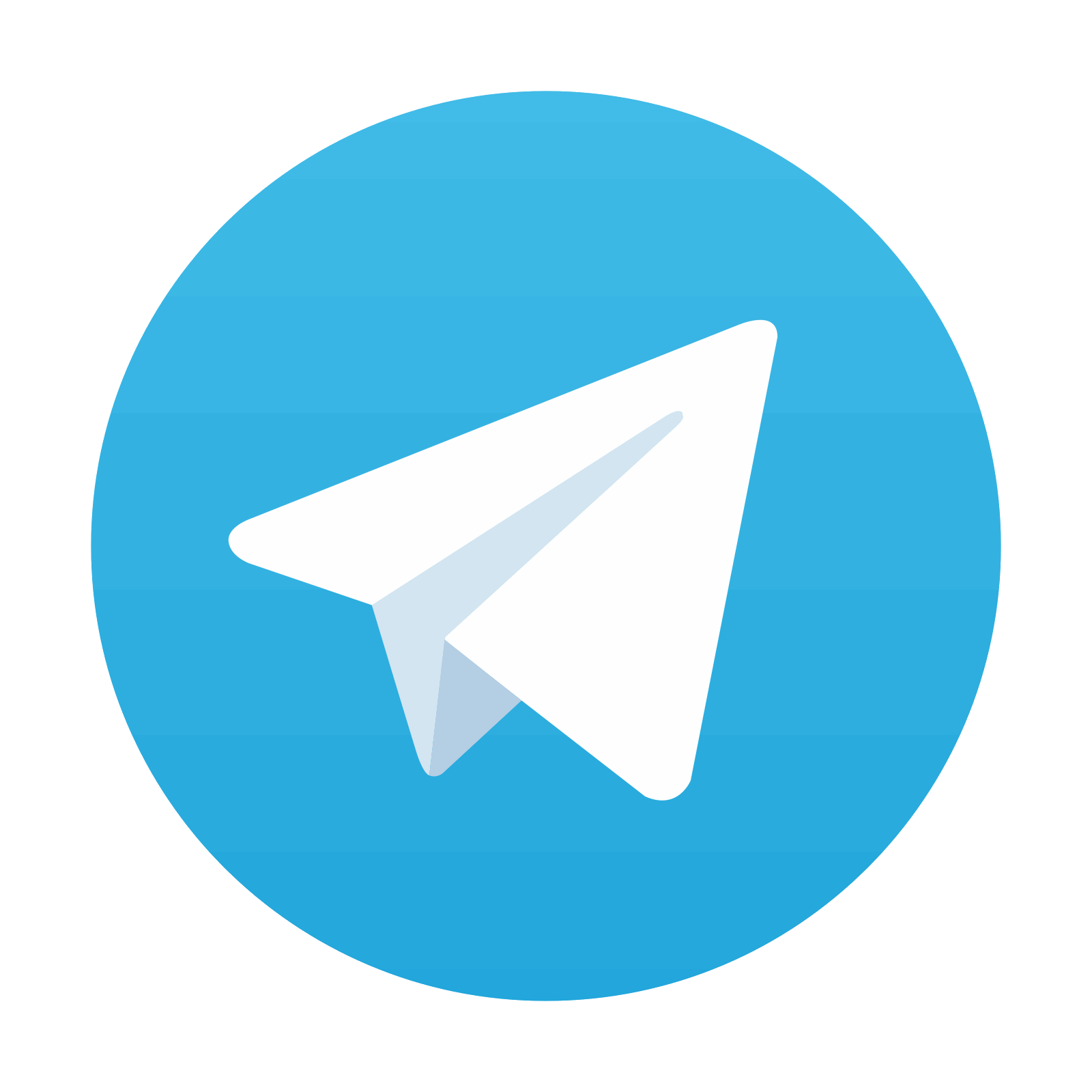
Stay updated, free articles. Join our Telegram channel

Full access? Get Clinical Tree
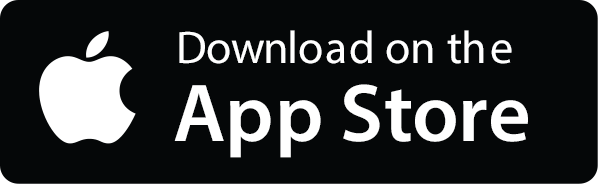
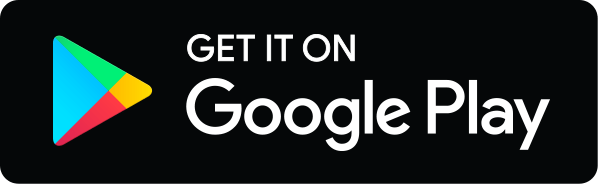