Introduction
Cardiac arrest may complicate any type of heart disease, but is particularly common in coronary heart disease; one half of all deaths caused by the condition present as sudden cardiac death.1 Approximately 400000–460000 individuals in the USA2 and 700 000 in Europe3 experience sudden cardiac arrest each year, making the condition a major health problem (Level C).2,3 Unexpected cardiac arrest also remains an important problem in hospital with an incidence of 0.174 ± 0.087 per bed per year reported in one recent large American multicenter cohort study.4
The modern era of resuscitation began with the development of defibrillators suitable for clinical use5,6 and the demonstration of the effectiveness of expired air ventilation and closed chest compression that extended the time available for successful defibrillation.7,8 The development of the portable defibrillator made resuscitation outside hospital a practical proposition. Particular credit goes to Pantridge in Belfast who recognized that hospital-based coronary care units would have limited impact on mortality in a condition where 60% of deaths occurred within one hour of the onset of symptoms.9 He developed the first mobile coronary care unit in the world and the report of 10 patients admitted alive to hospital following defibrillation10 acted as a stimulus to the development of similar strategies for managing cardiac arrest worldwide.
Establishing an evidence base for resuscitation practice
The development of effective resuscitation strategies led to a demand for standardized guidelines for clinical practice.
A commitment to foster an international evidence base for resuscitation practice and publish consistent guidelines for practice led to the foundation of the International Liaison Committee on Resuscitation (ILCOR). A regular five-year cycle of evidence review and publication of guidelines is now well established.11–14
The ILCOR methodology is based on a rigorous evaluation of all the available evidence relating to a specific topic using standardized worksheets,15,16 which remain accessible at www.c2005.org.17 In 2005, guidelines for practice were published with only minor differences between those of the European Resuscitation Council18,20 and the American Heart Association.19,21
In this chapter, the basis for the practice of resuscitation of adult subjects is reviewed in the light of the evidence evaluation process described above. Common to nearly all resuscitation attempts is the application of basic life support to maintain breathing and a circulation until definitive treatment with defibrillation or other advanced techniques can be implemented. It is one of the few strategies proved to improve outcome from cardiac arrest and is the subject of the first section.
The remainder of the chapter is concerned with advanced life support topics. Defibrillation is considered in detail in the second section; it is the only definitive treatment for the majority of patients with cardiac arrest, and one of the few techniques with an unequivocal evidence base for its effectiveness. The third section is concerned with other advanced life support techniques that aim to maintain breathing and the circulation while treatment to restore the circulation is given.
Recognition of cardiac arrest
The prompt recognition of cardiac arrest is the key to starting resuscitation with the minimum of delay; recent studies have helped clarify the most accurate methods for diagnosing cardiac arrest. The traditional method of palpating the carotid pulse has now been shown to be unreliable22,23 (Level B) although there is no convincing evidence that checking for movement, breathing or coughing is superior24 (Level C). Agonal gasps are common after the onset of cardiac arrest and bystanders often report that such subjects are breathing when interrogated by ambulance call takers. This can result in CPR being withheld or delayed25 (Level C). It is recommended therefore that rescuers should start CPR in an unresponsive (unconscious) victim who is not moving or breathing. Even if the victim is taking occasional gasps, cardiac arrest should be suspected and CPR started (Class IIa).
Airway and ventilation
Opening the airway
Several prospective studies using clinical or radiologic indicators of airway patency have shown that the head tilt – chin lift method is practicable, safe and effective26–28 (Level C). This was confirmed in a case series29 (Level C). It is recommended that rescuers should use this method to open the airway (Class IIa). No study has evaluated the use of routine finger sweeps to clear the airway in the absence of an obvious obstruction; finger sweeps should only be employed if a visible obstruction is present in the oropharnyx (Class IIb).
Ventilation
Manikin studies30 and one human study31 showed that when no advanced airway (e.g. a tracheal tube) was present a tidal volume of 1 L produced significantly more gastric distension than 500mL (Level C). In anethetized patients with no advanced airway in place, ventilation with 455 mL of room air was associated with acceptable although reduced oxygen saturation when compared with 719 mL. There was no difference in oxygen saturation between volumes of 624 mL and 719 mL32 (Level C). Another study compared patients in cardiac arrest mechanically ventilated with oxygen at 12 L per minute. Smaller volumes of 500 mL were associated with a higher arterial PCO 2 and worse acidosis but no differences in PaO 2 than with volumes of 1L33 (Level B).
Small case series34 and an animal study35 showed that hyperventilation is associated with increased intrathoracic pressure, decreased coronary and cerebral perfusion and (in the animal study) decreased return of spontaneous circulation (ROSC). A case series of patients showed that ventilation rates of >10min-1 and inspiratory times of > 1 s were associated with poor survival37 (Level C). In summary, larger tidal volumes and higher ventilation rates may be detrimental while the adverse consequences of smaller volumes appear acceptable.
The recommended technique for mouth-to-mouth ventilation using exhaled air or bag-valve-mask ventilation using room air or oxygen is to give one breath within an inspiratory time of 1 s (Class IIa) sufficient to make the chest rise (Class IIa). After an advanced airway is in place (e.g. tracheal tube, Combitube ™, laryngeal mask airway, etc.), the patient should be ventilated with supplementary oxygen sufficient to make the chest rise. In such patients the lungs should be ventilated at a rate of 8 – 10 ventilations min-1 without pausing during chest compressions to deliver ventilations (Class IIa). There were insufficient data to recommend for or against the use of mechanical ventilators.
Chest compressions
The crucial importance of chest compressions has been increasingly recognized in recent years (for review of the subject see Chamberlain, Handley and Colquhoun).36 Unfortunately there is increasing evidence that compressions are performed particularly badly in practice. In recent treatment recommendations, particular emphasis is placed on ways of increasing the number and quality of chest compressions during resuscitation attempts.
Hand position
There is insufficient evidence to recommend any one specific hand position when performing chest compressions in adults. Based on manikin studies in healthcare professionals demonstrating shorter pauses between ventilations and compressions37 and improved performance38 it is recommended that the hands should be placed ‘in the centre of the chest’ with the dominant hand in contact with the sternum (Class IIa, Level C).
Rate and depth
Studies in humans have given conflicting results; in one study a rate of 120 min-1 provided improved hemodynamics over standard cardiopulmonary resuscitation (CPR)39 (Level C). Studies that employed mechanical compression, however, have showed no improvement with rates of up to 140min-1 compared to 60 min-140,41 (Level C). Observational studies show that hospital healthcare providers42,43 and emergency medical services (EMS) personnel44,45 give fewer compressions than currently recommended (Level C). These studies43,44 also showed that compression depth is often inadequate.
In animal models of cardiac arrest, deeper compressions correlated with increased ROSC and 24-hour survival than standard compression depths46–48 (Level C).
Decompression of the chest
In one animal study incomplete chest recoil was associated with increased intrathoracic pressure, decreased venous return and decreased coronary and cerebral perfusion.49 There are no comparable human data. Lifting the hands slightly but completely off the chest between compressions allowed full recoil of the chest in a manikin study50 (Level C). Observational studies in human CPR35 and manikin studies50 have shown that incomplete chest recoil is common during CPR (Level C).
Duty cycle
This term describes the time spent in compression as a proportion of the time between the start of one compression and the next. Coronary blood flow is determined in part by duty cycle (reduced when > 50%) and the extent of chest relaxation at the end of each compression51 (Level C). There is a lack of clinical evidence from human studies, but data from animal or mathematical models suggest a cycle of 20 – 50% is optimal46,52–55 (Level C).
A duty cycle of 50% is much easier to achieve than cycles where compressions constitute a smaller percentage of the time,56 an important practical issue for both performance and training (Level C).
Following analysis of this evidence, it is recommended that chest compressions should be performed with the hands placed in the center of the chest (Class IIa) at a rate of at least 100 min-1 (Class IIa), compressing the sternum to a depth of at least 4 – 5 cm. Rescuers should allow complete recoil of the chest between compressions (Class Ilb). It is reasonable to use a duty cycle of 50% (Class Ilb). Rescuers should minimize interruption of chest compressions for checking the pulse, analyzing rhythm or performing other activities (Class IIa).
A manikin study showed that compression depth deteriorated within one minute, but rescuers only became aware of fatigue after five minutes57 (Level C). In consequence, it is recommended that where feasible, rescuers should rotate the duties of compression regardless of whether they feel fatigued (Class Ilb).
Compression ventilation sequence
Interrupting compressions
Observational studies and secondary analysis of randomized trials have shown that interruption of chest compressions is commonplace42,44,58 (Level C). Animal studies consistently show that interruptions in chest compression are associated with reduced ROSC, survival and postresuscitation myocardial dysfunction59–61 (Level C). One retrospective study that employed ventricular fibrillation (VF) waveform analysis to predict the probability of defibrillation showed that interruptions in chest compression were associated with a reduced chance of conversion to another rhythm62 (Level C).
On the basis of this evidence it is recommended that rescuers should minimize interruptions to chest compression (Class IIa).
Compression/ventilation ratio during CPR
The number of compressions delivered per minute is determined by the compression rate, the compression/ventilation ratio and the time required to provide ventilation. Evidence from clinical studies shows that rescuers tend to ventilate too quickly and perform too few chest compressions when observed in actual resuscitation attempts. One observational study showed that paramedics perform ventilation at excessive rates in intubated patients and the same was found in a study of hospital responders both with and without an advanced airway in place35,42,44 (Level C). A study of downloads from automated external defibrillators (AEDs) used by EMS personnel showed that chest compressions are frequently delivered at rates other than those recommended in guidelines. Adherence to recommended rates appeared to be associated with improved outcome45 (Level C). A comparable study in trained lay responders in public access defibrillation (PAD) schemes showed widely differing rates of compression, often given too quickly, but pauses were also commonplace.63 The compression/ventilation ratios employed also varied considerably. The AED algorithms (that require a “hands-off” period during rhythm analysis) were responsible for some of the pauses, but many were prolonged and unexplained. The outcome was that the number of compressions given was very much lower than recommended in guidelines (Level C).
Animal studies consistently show that hyperventilation is associated with lower coronary and cerebral perfusion and poorer survival while chest compressions given continuously or with minimal interruptions are associated with improved hemodynamics and improved survival59,60,64–66 (Level C).
Various compression/ventilation ratios have been applied in animal models of cardiac arrest but without a consistent outcome on which to recommend any one particular ratio.67–69 There are no adequate data from human studies. A mathematical analysis suggested that a ratio of 30/2 would provide the best blood flow and oxygen delivery70 (Level C).
To optimize the number of compressions delivered, to reduce interruptions and simplify instruction (and skill retention), it is recommended that a single compression/ ventilation ratio of 30/2 be used by the lone rescuer of infants, children and adults (Class IIa). Compressions should be delivered at a rate of at least 100 per minute (Class IIa).
Initial steps in the resuscitation sequence may include:
- opening the airway while assessing the patient
- giving 2-5 breaths while initiating resuscitation
- continuing resuscitation with a compression/ventilation ratio of 30/2.
Chest compression-only CPR
There have been no prospective human studies of compression-only CPR. A randomized prospective trial of compression-only CPR prior to EMS arrival in Seattle (a city with a very rapid EMS response time) showed a trend towards improved survival in those receiving compressions only, but the difference was not statistically significant71 (Level B). Animal studies have shown compression-only CPR to be as effective as conventional CPR with ventilation in the first few minutes after the onset of cardiac arrest.64–66 A compression/ventilation ratio of 30/2 maintained arterial oxygen saturation at two-thirds of normal whereas compressions only resulted in oxygen desaturation within two minutes.69
In observational studies of adult cardiac arrest treated by lay persons trained in standard CPR, survival with compression-only CPR was better than when victims received no CPR, but not as good as when victims received both compressions and ventilations72,73 (Level C).
On the basis of this evidence, the recommendation is that rescuers should provide compression-only CPR if they are unwilling to perform airway and breathing maneuvers or if they are untrained and uncertain how to undertake CPR (Class IIa).
Advanced life support: defibrillation
Procedures before defibrillation
The precordial thump
There are no prospective studies of the precordial thump. Case series have reported VF or pulseless ventricular tachycardia (VT) converted to a perfusing rhythm following a precordial thump.74–76 The likelihood of success was higher in VT74–78 but decreased rapidly with time.76 Observational studies also report that other arrhythmias including unstable supraventricular tachycardia (SVT) were terminated by a precordial thump79,80 (Level C).
Observational studies show that an effective precordial thump is delivered by a closed fist from a height of 5–40cm.75,76,78 Adverse events include acceleration of the rate of VT, conversion of VT into VF, the production of complete heart block and asystole.75,77,78,81–84 Current data do not allow these events to be reliably predicted (Level C).
The recommendation for practice is that one immediate precordial thump may be considered after a monitored arrest if a defibrillator is not immediately available (Class Indeterminate).
CPR before defibrillation
One study that employed a retrospective control group85 (Level C) and one prospective study86 (Level B) showed that 1.5 – 3 minutes of CPR before attempted defibrillation improved ROSC and survival in adults with out-of-hospital VF or VT when the response interval was ≥4–5min. Another randomized trial reported that adults with VF or VT outside hospital did not benefit from 1.5 min of CPR87 (Level B). Animal studies of VF of ≥5min duration report that CPR before defibrillation (very often with concomitant adrenaline) improved survival rates88–91 (Level C).
The ILCOR recommendation reflects this evidence: 1.5 – 3 min of CPR before attempted defibrillation may be considered in adults with out-of-hospital VF or VT where EMS response times are greater than 4 – 5 min (Class Ilb). There was no evidence to support or refute the strategy in hospital.
Defibrillator electrode–patient interface
No studies have investigated the influence of electrode position on the outcome of human cardiac arrest. The subject has been investigated during conversion of stable atrial fibrillation or by the use of surrogate endpoints such as transthoracic impedance (TTI). Cardioversion of perfused myocardium in stable atrial fibrillation (AF) may not be the same as defibrillation of ischemic myocardium and caution is required when extrapolating from such studies. Many studies in AF have investigated the influence of electrode position and have lead to current recommendations. For references see92 (Level B or C).
One human study documented higher success with larger electrodes93; 12.8 cm pads were superior to 8 cm (Level B). Several studies have confirmed lower TTI with increased electrode size (Level B or C). One animal study reported increased myocardial damage in dogs with smaller electrodes (4.3 cm compared with 8 or 12 cm)94 (Level C).
The recommendation is that electrodes should be placed on the exposed chest in an anterolateral position (Class IIa). Acceptable alternatives are the anteroposterior and apex-posterior position (Class IIa). Placing the electrode on the female breast increased TTI95 and in consequence the electrode should be placed underneath or lateral to the left breast (Level C).
Self-adhesive defibrillation pads versus paddles
One randomized trial96 and two retrospective series97,98 showed that the TTI was similar with both types of electrode (Level B, C). One randomized study showed similar effectiveness in converting AF99 (Level C).
Self-adhesive pads are safe and effective and are an acceptable alternative to standard defibrillation paddles (Class IIa). There are important practical advantages with pads for routine monitoring and for perioperative or prehospital use.
Waveform analysis
Analysis of the VF waveform has the potential to improve the timing and effectiveness of countershocks. Evidence comes from retrospective analysis of human series and animal models (Level C). The technology is advancing rapidly but is not yet available in routine clinical practice.
Defibrillator shock waveform
A major advance in defibrillator technology in recent years has been the introduction of biphasic waveforms. Randomized clinical studies in human cardiac arrest100–102 (Level B), reanalysis of the data in one study,103 two observational studies104,105 (Level C) and many animal studies show that biphasic waveforms using equal or lower energy levels are superior to or at least as effective as monophasic waveforms for terminating VF. A meta-analysis of seven randomized trials in patients in the electrophysiology laboratory105 concluded that biphasic waveforms defibrillate with similar efficacy at lower energies than monopha-sic 200 J shocks, and greater efficacy than monophasic shocks of the same energy (Level A). No specific biphasic waveform has yet been demonstrated to be superior. One retrospective study106 showed a higher hospital discharge rate with a monophasic truncated exponential (MTE) waveform compared to a biphasic truncated exponential (BTE) waveform. Survival was a secondary endpoint, however, and there were many confounding factors, not least the higher incidence of bystander CPR in the MTE group.
The recommendation for practice is that biphasic shocks are safe and effective for the termination of VF when compared with monophasic defibrillators (Class IIa).
First shock energy levels
Many clinical studies have compared initial biphasic shock energies of 100 – 200 J without clearly showing an advantage for either100–102,104,106,107 (Level B, C). These studies also investigated subsequent energy levels ranging from 150 J to 300 J for VF refractory to the initial shock but without clearly demonstrating an optimal level. Other laboratory studies of stable patients evaluated termination of induced VF by energy levels of 115 – 200 J No clinical or laboratory study has convincingly shown greater benefit or harm from any currently used energy level. A hospital study compared first shock efficacy of monophasic damped sinusoidal (MDS) waveforms of low (100 – 240J), intermediate (300–320J) and high (400–440J) without demonstrating a statistically significant benefit for any level.108
There is currently insufficient evidence to recommend a specific energy level for the first or subsequent biphasic shock. ILCOR recommend that with a biphasic defibrillator it is reasonable to use 150 – 200 J with a BTE waveform or 120 J with a rectilinear waveform (Class IIa). For a mono-phasic defibrillator an initial shock of 360 J is considered a reasonable option (Class IIa).
Second and subsequent shocks
Fixed versus escalating energy levels
Only one small human study compared fixed energy with escalating energies for subsequent shocks from a biphasic defibrillator. There was no clear benefit for either strategy109 (Level C). No recommendation for either strategy could therefore be made.
One shock versus three shock sequence
No clinical or animal studies have compared a protocol that employs a single shock with one that employs three successive shocks before the resumption of chest compressions. Many studies have investigated the efficacy of first or subsequent shocks; success is defined as the termination of VF for >5s after the shock. This is an assessment made on the ECG, however, and does not necessarily indicate the return of the circulation.
The efficacy of the first shock given by a variety of waveforms and energy levels has been investigated in several trials of out-of-hospital VF.92 A summary of the results is as follows.
- With a 200 J MDS waveform first shock success was 77–91%.
- With a 200J MTE waveform, the rate was 54-63%.
- With a 150J BTE waveform (four studies) and one using a 200 J BTE waveform, first shock success was 86-98%.
- With a 120J rectilinear biphasic waveform first shock success rate was 85%.
Although the first shock success at converting the rhythm was relatively high, the average rate of ROSC with the first shock for all three waveforms was only 21% (range 13-23%).
Second and third shock success rate
Where the patient remained in VF after the first shock:
- with the MDS waveform with increasing energy levels (200 J to 200-300 J to 360 J), the combined success of the second and or third shocks was 68-72%
- with the MTE waveform with increasing energy levels (200 J to 200-360 J) the combined success of the second and/ or third shocks was 27-60%
- with the fixed (non-escalating) 150 J BTE waveform, the combined success of the second and or third shocks was 50-90%.
The single study that used a rectilinear escalating waveform (120 J to 150-200 J reported combined success of second and/or third shocks of 85%.
In the absence of any evidence for a clearly superior strategy, ILCOR recommended that practice should concentrate on procedures that optimize the chances of successful defibrillation regardless of the precise energy, waveform or shock sequence. These priorities were to recognize the potential need for defibrillation as soon as possible, provide CPR until a defibrillator is available and minimize interruptions to chest compressions (Class I).
A one-shock strategy may improve outcome by reducing interruptions in chest compressions. A stacked sequence of three shocks can be optimized by the immediate resumption of effective chest compressions after each shock (irrespective of the rhythm) and by minimizing the “hands-off” periods for rhythm analysis or other procedures.
Advanced life support: other considerations
Airway and ventilation
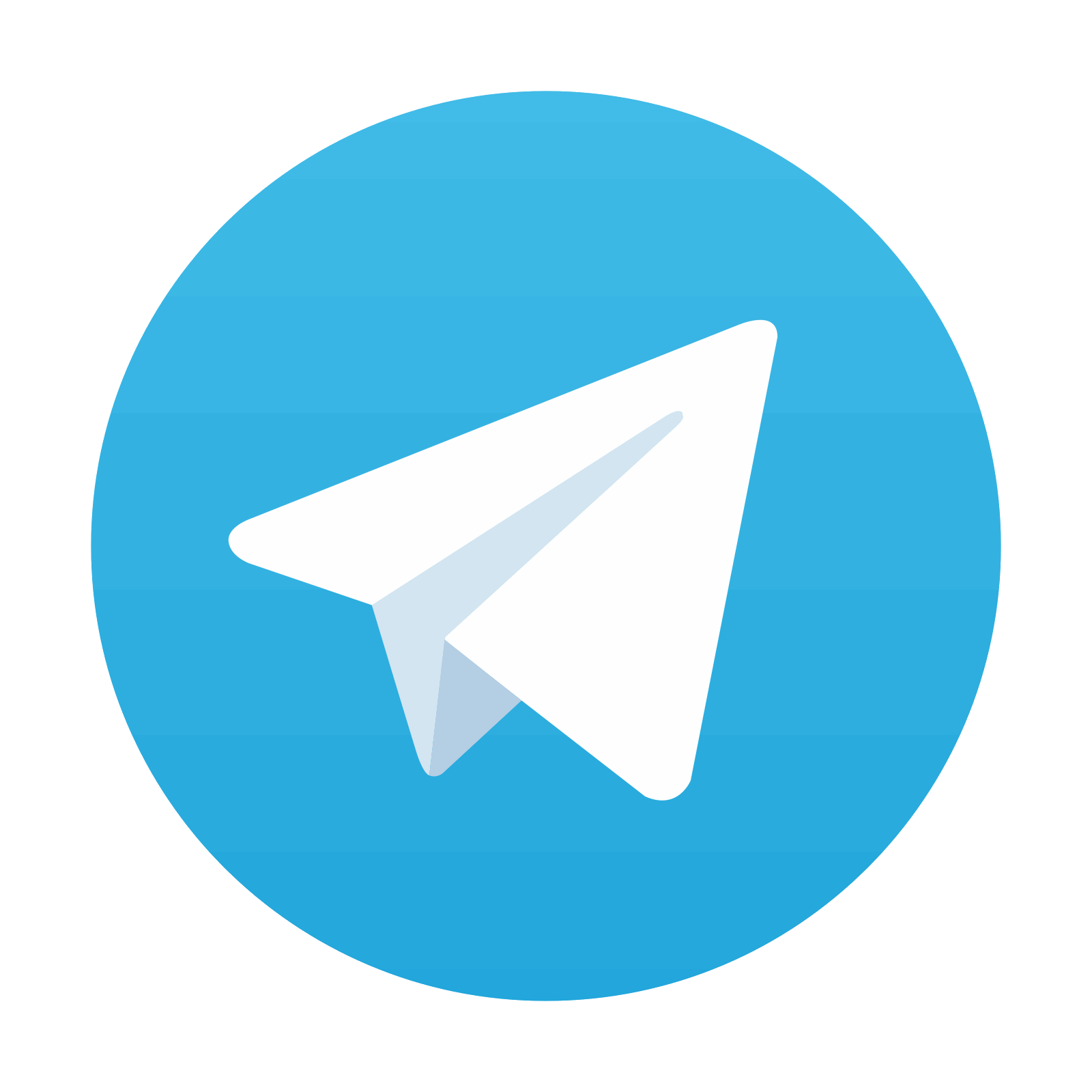
Stay updated, free articles. Join our Telegram channel

Full access? Get Clinical Tree
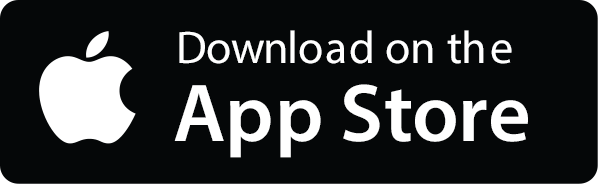
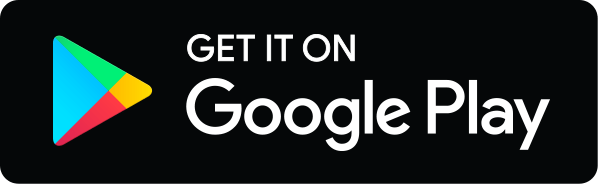