and Daniel P. Credeur3
(1)
Pediatric Center for Respiratory, Exercise and Sleep Medicine, Athletic Training Facility Football Operations, Louisiana State University, Baton Rouge, LA, USA
(2)
Louisiana Healthcare Connections, 8585 Archive Blvd Suite 301, Baton Rouge, LA 70809, USA
(3)
Department of Medical Pharmacology and Physiology, University of Missouri, 1 Hospital Dr., Medical Sciences Bldg. M415, Columbia, MO 65212, USA
Abstract
The use of exercise testing is an important tool for the pediatric pulmonologist. Physical stress often reveals cardiorespiratory abnormalities that are not apparent on conventional static tests. There is little doubt the information acquired from exercise tests have diagnostic and prognostic value, but exercise tests may also be critical to determine success or failure of treatment strategies. The purpose of this chapter is to provide the pediatric specialist with an overview of exercise evaluation that may assist in the diagnosis of and management of disease in pediatric and adolescent patients with physical activity intolerance, especially those with cardiorespiratory diseases. The specific aims of this chapter are to: (1) describe various exercise tests and the biomechanical and physiological principles of those tests necessary to assess patients; (2) discuss methods to assess the physical fitness profile of patients with cardiorespiratory diseases and physical limitations; (3) provide specific strategies for an exercise prescription based on the fitness and clinical profile of the patient and; (4) assist the provider in developing a center for exercise evaluation. Although each aim provides unique information, the overall goal of this chapter is to stimulate the pediatric pulmonologist to develop an understanding of indications for cardiopulmonary exercise testing (CPET) and implement strategies to systematically assess each patient’s fitness profile; to track patient’s disease or training progression or alternatively to monitor responses of medical interventions; and to prescribe a well-rounded exercise prescription to maximize functional ability and wellness of individual patients. This chapter is not intended to be an in depth review for exercise physiologists, but is designed to assist the practicing clinician in the logistics of developing a program and a comfort in interpreting studies.
Keywords
Exercise testingExercise evaluationPediatric pulmonologyTreatment strategiesCardiorespiratory diseasePhysical fitness profileCardiovascular exercise testingIntroduction
Exercise testing consists of an analysis of the functional capacity to engage in activities of daily living (ADL) and higher levels of physical activity and or exercise. Humans engage in various forms of physical activity through integrated skeletal muscle movements requiring energy. Any physical activity in which work is performed by skeletal muscle can be regarded as exercise. It is important to differentiate between resistance and endurance exercises. Resistance exercise consists of brief periods of muscle contraction, such as weight lifting, and endurance exercise consists of rhythmic contractions for extended periods of time, such as in walking or running. The energy used for skeletal muscle use is derived from the chemical breakdown of carbohydrates, fats, and protein to energize mitochondria within muscle cells to contract and relax. This energy pathway is composed of a system of enzymes, substrates, and oxygen that eventually result in the production of (adenosine triphosphate) ATP. There are many good textbooks and reviews which describe the processes on bioenergetics of muscular activity and concepts of clinical exercise testing for diagnostic purpose [1–8]. The authors especially recommend the chapter written by Morton in Pediatric Pulmonary Medicine [5]. This chapter (Morton) is an outstanding overview of exercise physiology and applies these principles to pediatrics.
The use of physical stressors often reveal cardiopulmonary abnormalities that are not evident on resting pulmonary function or cardiovascular evaluations like electrocardiograms (ECG), echocardiograms, and spirometry to name a few. This chapter is not intended to be a footprint of previous textbooks and but is to assist clinicians in developing a program to utilize exercise techniques and equipment for the purpose of evaluating exercise intolerance and developing exercise prescription. Understanding the type of evaluation needed allows the clinician to develop organization and logistics to determine which personnel and equipment will be required to perform the tests, execution of the evaluation, and to interpret results to develop an individualized exercise prescription to treat exercise intolerance in the pediatric or adolescent patient.
Understanding the basic concepts of cardiopulmonary exercise testing (CPET) has never been more important than present. Reduced physical activity and a sedentary lifestyle are associated with chronic conditions including coronary artery disease, musculoskeletal and metabolic disorders such as type-II diabetes and obesity [9]. Childhood obesity rates continue to climb and physical inactivity in children and adults present significant challenges to public health [10]. Obesity and physical inactivity usually begin in childhood and often become a lifelong issue with a plethora of health disparities as illustrated in Fig. 12.1. Unfortunately, we deal with many outside influences which confuse the true science and medicine around these problems. Such interferences include the Internet, blogs, social media, sports news, and journalists. There are many practitioners in the field of exercise and training with no significant education in physiology and biomechanics of exercise, who often recommend techniques and diets which are unhealthy and generate revenue [11]. This is especially true in the supplement market which has potential to do more harm than improve performance [12]. The aware clinician interested in providing a comprehensive exercise evaluation and prescription should also be informed of dietary and sleep needs of patients as these may affect physical activity and capacity [13]. This is especially true of those who are dealing with performance athletes [14]. Performance athletes are susceptible to these external stimuli as the level of competition has much higher stakes including scholarships and professional contracts. Performance athlete coaches and trainers and sometimes team physicians have little to no experience in exercise science and may encourage behaviors and prescribe methods that are clearly incorrect and can actually lead to disability of their athlete. Prohibited and dangerous methods of training, dietary supplementation, and drug use are clearly defined by organizations like the National Collegiate Athletic Association (NCAA) and others listed in Table 12.1, but very few high school athletic associations regulate or investigate inappropriate use of supplements and training practices [15]. Organizations such as those listed in Table 12.1 study the effects of exercise in all age groups and provide education, training, and certification in the principles of exercise testing and prescription.
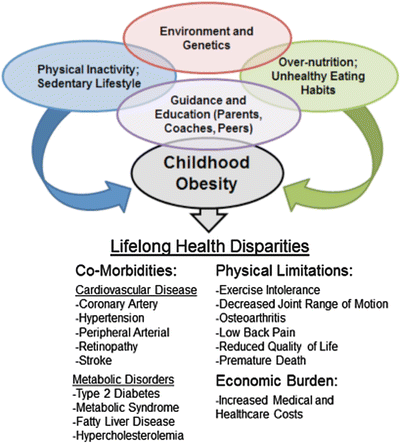
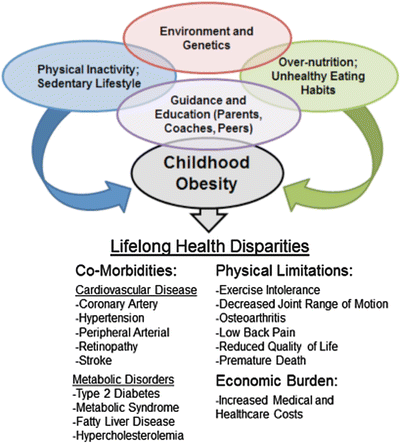
Fig. 12.1
Health disparities. Integration of comorbidities resulting in childhood obesity and lifelong health disparities of impairment and risk
Table 12.1
National, international, and regional organizations providing education, regulation and/or certification of sports and exercise training
Organization | URL |
---|---|
NCAA | |
WADA | |
IOC | |
NASM | |
NSCA | |
ACSM | |
PES | |
USUHS |
This chapter is not intended to discuss in detail the exercise science beyond that required to develop a suitable laboratory environment and interpret clinical studies. However, some understanding of exercise physiology is needed to evaluate physical activity intolerance. The heart and lungs work together as a unit to supply oxygen to tissues in relation to their metabolic needs. As tissue needs for oxygen increase (as they do in contracting muscles during exercise), there are certain predictable responses in individuals with a normal functioning heart, lungs, and vasculature. When exercise intolerance develops, disease, or deconditioning may be present in either the (1) cardiovascular, (2) respiratory, or (3) musculoskeletal system. Behavioral problems can also limit exercise capacity and a well conducted exercise test can also provide valuable information for initiating cognitive behavior therapy [16]. Adverse responses to exercise and physical activity are predictable deviations from the normal. Exercise tests, with measurements of both cardiovascular and respiratory function, are often called cardiorespiratory or cardiopulmonary exercise (CPET) testing. The CPET can provide valuable noninvasive information about the functioning of both the cardiovascular and respiratory systems. To assist the clinician in understanding the indications and interpretation of exercise intolerance, important concepts in exercise physiology and testing are briefly reviewed in Table 12.2.
Table 12.2
Exercise testing components and description
Component | Description |
---|---|
1. VO2 (maximal oxygen uptake) | – Maximal amount of oxygen taken up by the respiratory system, distributed by the cardiovascular system and utilized by metabolically active tissue (i.e., contracting skeletal muscle) during heavy aerobic exercise |
2. Importance of oxygen uptake | – Ensures adequate supply, delivery, and utilization of oxygen to sustain metabolic processes and muscular work |
3. Measurement of VO2 | – Measuring the volume of oxygen consumed through spirometry, arterial-venous oxygen differences, and or using validated estimation equations based on heart rate and workload (i.e. Bruce Treadmill protocol, YMCA cycle test) |
4. Variables obtained during CPET testing | – Volume of oxygen consumed (VO2) – Volume of carbon dioxide produced (VCO2) – Pulmonary ventilation (L/min) – Ventilatory threshold (VT) – Lactate threshold (LT) – Respiratory exchange ratio (RER) – Rating of perceived exertion (RPE) • Borg scale • Dyspnea scale • Angina scale |
5. Exercise testing for diagnostic purposes | – ECG recordings • Monitor heart rate and myocardial electrical disturbances • Blood pressure responses |
6. Methods for CPET | – Bruce treadmill protocol – YMCA cycle test |
7. Methods to determine exercise intolerance in performance athletes | – Sport-specific exercise tests • Shuttle runs • Burpees • Box jumps • Beep tests |
8. Age influences on physiological adjustments to exercise with respect to age appropriate physiology | – Cardiorespiratory responses • Increased cardiac output (Blunted in elderly, greater in children and adolescents) • Increased arterial–venous O2 difference(Blunted in elderly, greater in children and adolescents) • Increased skeletal muscle blood (Blunted in elderly, greater in children and adolescents) • Increased ventilation (Blunted in elderly, greater in children and adolescents) • Increased arterial blood pressure (Increased in elderly) |
Defining Maximal Oxygen Uptake
The relationship between the amounts of oxygen consumed and work performed is predictable, and since the body has limited capacity for storing oxygen, the rate of oxygen uptake at the lung represents rate of cellular oxygen consumption. Oxygen uptake (VO2) is proportional to the external work of physical activities. Maximal oxygen uptake is defined as the maximal amount of oxygen distributed and used by the body while performing heavy exercise. A high VO2 requires the respiratory system to exchange oxygen and carbon dioxide within blood; the cardiovascular system to pump and distribute oxygen-laden blood (hemoglobin) to active skeletal muscle; and the ability of the skeletal muscle to convert stored substrates to power muscular contraction. Maximal oxygen uptake is abbreviated VO2 max; where V represents the volume of oxygen per minute, O2 represents oxygen, and max represents maximal amount or conditions. Thus, VO2 max is the maximal volume of oxygen used by the body per minute [1–8]. This value is usually expressed in absolute terms as liters per minute (L min−1) or in relative terms as milliliters per kilogram of body weight per minute (mL/kg min−1). VO2 max is considered the gold standard measure of maximal aerobic capacity or aerobic power. Because VO2 max is a measure of energy transfer, it is also considered a measure of aerobic power rather than just volume or capacity [1–8]. The by-product of aerobic power production is carbon dioxide (CO2), and the volume of CO2 eliminated per minute from the body is the VCO2. This variable is important and provides information regarding cardiopulmonary efficiency especially at the onset of nonaerobic or “anaerobic” metabolism (anaerobic glycolysis or lactic acid system) [1–8]. Except for steady state exercise, there is an additional variable of total metabolism from anaerobic processes. Indeed, aerobic metabolism persists in longer duration physical events; however, it is important to recognize that aerobic metabolism is only one component of metabolic rate during maximal exercise. At maximal exercise, metabolism (rate of energy transfer) is the sum of maximal aerobic power (VO2 max) and maximal anaerobic power. Some commonly used calculations to estimate VO2 are listed in Table 12.3. However, one should be cautious when using normative calculations in the pediatric population; especially for maximal heart rate calculations [17, 18]. Children are born with a set number of muscle cells, but development of strength which is characterized by increased diameter of muscle fibers (cells) is dependent upon changes into puberty. The emergence into Tanner 4–5 stages can be variable and use of age related calculations can be problematic.
Table 12.3
VO2 max prediction equations
Modality of exercise | Equation |
---|---|
1. Treadmill (walking) | – VO2 = 0.1(speed in m/min) + 1.8(speed in m/min) (grade %) + 3.5 |
2. Treadmill (running) | – VO2 = 0.2(speed in m/min) + 0.9(speed in m/min) (grade %) + 3.5 |
3. Stepping | – VO2 = 0.2(Step rate in steps/min) + 2.4(Step height) (Step rate in steps/min) + 3.5 |
4. Leg ergometry | – VO2 = 1.8(Work rate)/bodyweight in kg + 7 • Work rate (kg × m/min) = force setting in kg × pedal distance × pedal rate |
5. Arm ergometry | – VO2 = 3(Work rate)/bodyweight in kg + 3.5 • Work rate (kg × m/min) = force setting in kg × pedal distance × pedal rate |
6. Recovery HR (BPM) | – Following stepping: • 15 s count HR × 4 = BPM ° Male, VO2 max = 111.33 − (0.42 × HR) ° Female, VO2 max = 65.81 − (0.1847 × HR) – Walk/run tests • 12 min walk/run (male and female) ° VO2max = 3.126 x (meters covered) – 11.3 • 1.5 mile run test (male and female) ° VO2 max = 3.5 + 483/time |
Historically, the measurement of VO2 max can be a costly and time consuming operation confined to research laboratories. With the development of rapidly responding gas analyzers and volume measuring devices, along with advances in computer technology, this measurement is now accessible to scientists, clinicians, and fitness personnel. In fact there are now portable and even hand held VO2 testing devices available on the market [19–22]. When work rate increases on an ergonometric device (e.g., speed and grade on a treadmill or cycle ergometer) or during any physical activity, so does VO2 as long as the subject is not limited or deficient in any of the aforementioned physiological systems (i.e., cardiovascular, respiratory, hematologic, and musculoskeletal system). When approaching VO2 max work rate will usually peak or plateau. After the plateau, work rate may continue to increase with no further increase in VO2 (Fig. 12.2). Therefore, VO2 max represents the physiological limit of aerobic power. Although work rate may continue to increase following a plateau of VO2, this increase may be a product of other energy systems such as anaerobic power or metabolism [1–8]. Nonetheless, sustained work output will soon subside as muscular fatigue sets in. The magnitude increase in work rate beyond plateau does not affect the measurement of VO2 max, but instead can be used as an index anaerobic power as well as psychological motivation and persistence (Fig. 12.3) [1–8]. Table 12.4 lists important variables obtained during a CPET.
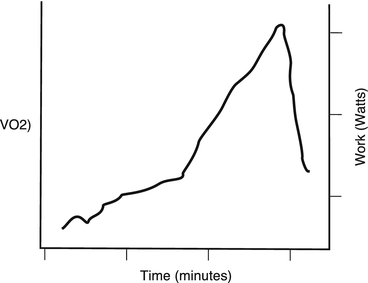
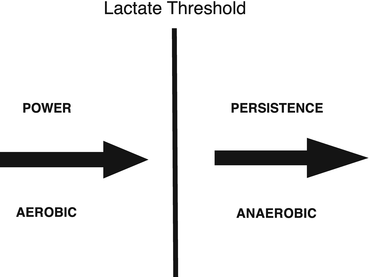
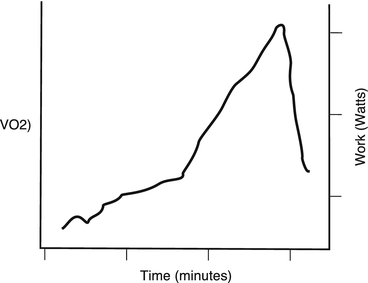
Fig. 12.2
VO2 max (peak). At VO2 max (peak) work rate will usually peak or plateau. After the plateau, work rate may continue to increase with no further increase in VO2 and this represents the physiological limit of aerobic power
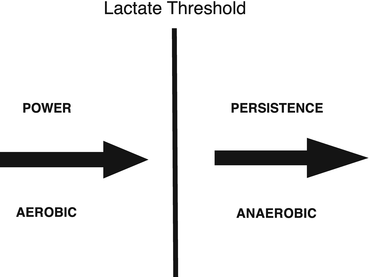
Fig. 12.3
Power and persistence. Once LT is achieved, power (work) is more a measure of anaerobic capacity and theoretical persistence or desire to continue
Table 12.4
Key variables of a CPET assessment
Peak VO2: (max) | Oxygen utilization (mLO2⋅kg−1⋅min−1): A measure of aerobic capacity; normal values are influenced by age and sex |
VT (AT estimate) | VO2 at the ventilatory threshold (mLO2 kg−1⋅min−1): A measure of submaximal exercise tolerance. Anerobic threshold (AT) |
Peak RER: | The ratio of exhaled CO2 to inhaled O2 |
VO2/w (mL/min/w): | Characterizes the ability of exercising muscle to extract oxygen (power) Low VO2/w relationship suggests cardiac or pulmonary impairment |
O2 pulse (mL O2/heart beat): | Approximates stroke volume |
PetCO2: | End-tidal CO2 or the level of CO2 in the air exhaled from the body (measured in mm Hg). Reduced values indicate VQ mismatching, and is consistent with worsening cardiac or pulmonary disease severity, and worse prognosis |
VE (L O2/min):Ventilation | (based on tidal volume and respiratory rate) during exercise. Peak VE can be assessed relative it can help determine if exercise intolerance or dyspnea relate to a pulmonary limitation |
VE/MVV: | Assessment of the maximum minute ventilation during exercise relative to maximum voluntary ventilation which is determined during PFTs at rest. The VE/MVV ratio is normally 80 % (and consistent with the premise that the pulmonary system is not limiting the exercise capacity |
VE/VCO2 slope: | Measurement of ventilatory efficiency (ie, minute ventilation relative to CO2 exhalation). Whereas VE/VCO2 slope is normally <30, efficiency decreases with cardiomyopathy intrinsic lung disease, and/or pulmonary hypertension, VE/VCO2 slope increases in each instance |
VD/VT | Dead space ventilation |
Pulse oximetry (% O2 saturation) | Decline in hemoglobin oxygenation levels, 90 % indicative of diminished ability to adequately increase alveolar-pulmonary capillary oxygen transfer during exercise |
Determining VO2 max
In the classic definition, VO2 max was presumed to occur when there was less than a 2.1 mL/kg min−1 increase in VO2 with an increase in treadmill grade of 2.5 % at speed of 7 mph. Thus, there would be an increase of less than 0.6 METs (metabolic equivalent of task) or about 25 % of the expected increase of 2.5 METS [1–5]. A metabolic equivalent is a physiological measure of the energy cost of physical activity defined as the ratio of metabolic rate (rate of energy consumption) during a specific physical activity to a reference metabolic rate, set by convention to 3.5 ml O2/kg min−1.
Other criteria for achievement of VO2 max in well-motivated subjects include (1) a respiratory exchange ratio (RER) greater than 1.0 and (2) a blood lactate concentration greater than 8 mmol L−1 (Table 12.5, [1–3]). In several studies involving both younger and older subjects, less than 50 % of these individuals could reach a plateau in VO2 at maximal work rates. Åstrand was the first to document that many children and adolescents complete a progressive exercise test to exhaustion without a plateau in VO2. Subsequent studies have confirmed only a minority of young people exhibit a classical VO2 plateau [23].
Table 12.5
Criteria for achievement of VO2 max
Criteria | Description |
---|---|
1. Plateau in VO2 | – Oxygen consumption plateaus or decreases despite an increase in workload |
2. Blood lactate > 8 mmol | – Indicates increased glycolytic production of lactic acid |
3. RER > 1.0 | – Respiratory exchange ratio, or respiratory quotient (VCO2/VO2) indicative of carbohydrate utilization (i.e. muscle glycogen) for continued energy production |
4. Age predicted maximum heart rate | – Traditional, 220—Age (max heart Rate declines with age) – More recently, 206.9—(0.67 × Age) |
5. Perceived exertion = 20 | – Subjective Perception of maximal exercise |
6. Rate pressure product = Systolic BP × heart rate (fivefold) | – RPP is an index of myocardial O2 demand |
7. ST-segment shifts | – Indicative of cardiac ischemia and/or injury |
Lactate or Anaerobic Threshold (LT or AT)
After plateau, work rate may continue to increase with no further increase in VO2. Therefore, VO2 max represents the physiological limit of aerobic power. Although work rate may continue to increase following a plateau of VO2, this increase is a product of anaerobic power or metabolism [1–4]. Nonetheless, sustained work output will soon subside as muscular fatigue develops. As previously mentioned, the magnitude increase in work rate beyond plateau does not affect the measurement of VO2 max, but instead can be used as an index anaerobic power as well as psychological motivation or persistence as represented by Fig. 12.3 [1–8]. The easiest method to determine the lactate threshold is known as the V-slope method by determining the ventilator anaerobic threshold (Fig. 12.4). The upward inflection in VCO2 is the VO2 at which ventilatory anaerobic threshold (VAT) is determined. The increase in VECO2 occurs when ventilatory buffering is needed to compensate for increased lactate driven metabolic acidosis. The magnitude of cardiac and ventilatory effort that a task represents and its tolerability to the patient are affected by whether the work is above or below lactate or anaerobic threshold. Therefore, Lactate threshold (LT) is a level of effort that is relevant to exercise tolerance or intolerance and the ventilatory threshold is a physiologic change in CO2 elimination that approximates LT. The rate of rise in lactate is also dependent upon the type of muscle fiber activated (type I—slow oxidative or type II—fast glycolytic) along with power need. In other words, the intensity and load duration can hasten the emergence of anaerobic metabolism. This concept is very important when testing children and adolescents, especially those in sporting activities. Specific sports and age groups often exercise in a burst patterns and performing a graded CPET may not be an adequate representation of their activity patterns and need for power to perform a sport or other ADLs [24].
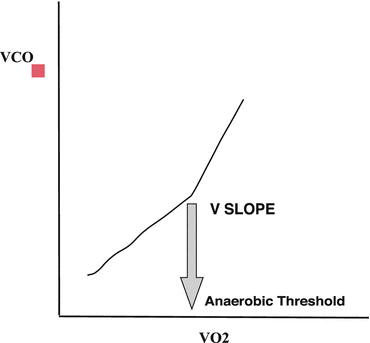
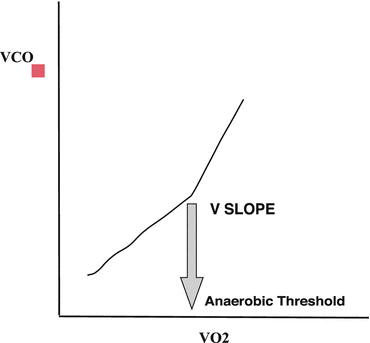
Fig. 12.4
LT measurements. There are several methods to estimate lactate threshold. The V-slope method is determined by break in linearity of the VCO2–VO2 graphical relationship
Lactate is the product of anaerobic glycolysis and is an available source of ATP, but is an inefficient energy source for sustained exercise as the energy produced is small compared to full aerobic oxidation. There is much confusion regarding the physiologic role of lactate. During anaerobic glycolysis the cofactor nicotinamide adenine dinucleotide (NAD) is regenerated during the conversion of pyruvate to lactate. The accumulation of lactic acid cannot be sustained; however, lactate is not what solely causes the muscle to fatigue. There are a multitude of reasons that muscle fatigues (i.e., pH drop due to Hydrogen ion accumulation) [25]. Lactic acidosis is also important for exercise performance as the fall in pH causes rightward shift of the oxy-hemoglobin dissociation curve facilitating the release of oxygen from hemoglobin (Bohr Effect; Fig. 12.5). In summary, muscular fatigue is not reflected alone on lactate level, but is a signal when energy sources have changed and fatigability (muscle inefficiency) is present. But muscle fatigue begins before the onset of anaerobic glycolysis (VO2 max).
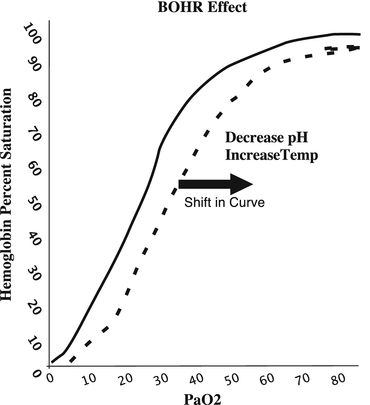
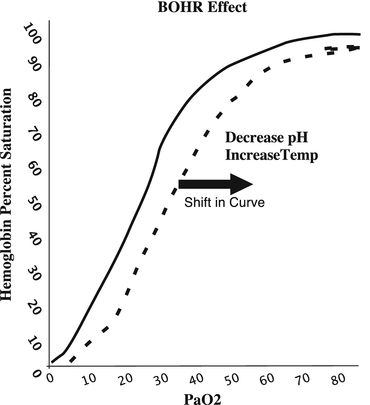
Fig. 12.5
Bohr Effect. An effect by which an increase of carbon dioxide in the blood and a decrease in pH results in a reduction of the affinity of hemoglobin for oxygen. At the muscle level the combination of heat and lower pH increases oxygen delivery to the mitochondria of contracting muscle
Purpose of Exercise Testing
The purpose of exercise testing is to assess cardiopulmonary, metabolic musculoskeletal power, and motivation/effort during physical stress. Physical stress magnifies the pathophysiology and physical limits, thus allowing the clinician to diagnose and develop interventions which are sport or activity specific. By considering time dimensions, the faster the heart and respiratory rate, the more samples available for analysis. The concept that a classical laboratory based CPET is needed is not necessarily true as exercise intolerance should also be addressed to include the biomechanics which trigger the event. The core of this evaluation is to determine functional capacity of the cardiopulmonary system and power of metabolic-muscular activity while reproducing symptoms or signs of the complaint. This process and the adaptations that occur with training or any exercise are listed in Table 12.6. The goal of laboratory based exercise testing is to address four key issues; (1) cardiovascular limitations, (2) ventilatory limitation, (3) metabolic or musculoskeletal limitation (biomechanics of activity) and (4) motivation or effort limitations or perceptions. The psychological aspects and expectations associated with exercise by the patient, and parents or coaches, can be a major contributor to the complaint [26].
Table 12.6
Adaptations that occur with training or any exercise
Parameter | Result | Magnitude | Variable measured CPET |
---|---|---|---|
Cardiac output | Increase | 4–5 | Pulse O2 |
Heart rate | Increase | 3 | HR |
Stroke volume | Increase | 2 | Pulse O2 |
Oxygen extraction | Increase | 3 | P (A − a)O2 |
O2-Hgb dissociation | Increase | – | |
BP systolic | Increase | 1.5 | BP |
BP diastolic | Minimal change | 1.1 | BP |
Pulse pressure | Increase | 1.3 | BP |
PVR | Decrease | 4.5 | – |
MVO2 | Increase | 4 | ECG |
Activity Intolerance and Exercise-Activity Fitness
Activity intolerance and exercise-activity fitness in the pediatric population and evaluation of those components is very age defined and can be difficult. Use of static volumes, resistances, and flows derived from conventional pulmonary functions, even if performed pre and post exercise, tell us little in regard to function during periods of increased physical demand (i.e., exercise). This includes newer modalities involving impulse oscillometry. Importantly, the younger the population the more difficult is the technique for measurement. In addition, some of the techniques presently used in the infant population are technologically challenging and require significant technical skills. There are relationships between classical pulmonary function tests and functional capacity, but there is no predictive consistency as these measurements do not take into account the individuals power needs. Evaluation of cardiac function by ECG and echocardiogram in a non-exercise study do not give us a clear depiction of higher level dynamics and functional risks. These cardiopulmonary measurements lack specific enough information to assess impairment or risk when the system is placed under stress. The absence of this data limits a clinician’s ability to fully assess the effectiveness of pharmacologic and or physical therapies designed to promote health and encourage activity.
Intolerance to exercise may have nothing to do with the cardiopulmonary system but may reflect muscle fatigue, fatigability, or incomplete recovery. The delivery of oxygen and elimination of carbon dioxide is most the time normal for the pediatric patient’s capacity. The associated dyspnea with exertion is really a reflection that the system is working well and that the problem is more related to power efficiency which is being perturbed by intrinsic muscle inefficiency or neurologic/psychological causes. The measurement of VO2 provides the clinician with information at a very fundamental level representing only a pathway into asking if the cause of activity intolerance is neuropsychological, nonneurologic (cardiopulmonary), or peripheral (muscle or local neural adaptations).
Evaluation and Assessment of the Infant and Pre-school Child with Observed Activity Intolerance
Infants and pre-school children are active in various ways and the activity intensity is dependent upon their neurodevelopmental age. Simple evaluation of child’s neurodevelopment may be a valuable starting point for infants and toddlers and ultimately determines in many cases the activity capacity, power needs, and ability for future participation at any level. Physical evaluation can identify issues related to musculoskeletal abnormalities and dysplasia which in exercise-activity evaluation involves either (1) muscle strength (hypo or hypertonia), (2) skeletal development of the limbs especially including skeletal dysplasia of the chest wall, extremities and/or spine and (3) cardiopulmonary performance. These three areas of exercise physiology are determined by muscular strength, endurance, power and leverage, and ability to provide cardiopulmonary aerobic and anaerobic output to meet power requirements. In reality, the efficiency of performance is the result of metabolic-mitochondrial biochemistry where energy is produced to meet power needs. The first objective data to be gathered is the cardiopulmonary exam. When examining the infant or toddler it is best to think in terms of the following physiologic concepts; (1) neurodevelopmental level of ability and agility, (2) chronotropy, (3) inotropy, (4) strength, (5) anatomy/anomaly, (6) chest wall kinetics, and (7) breathe sounds-airflow (gas exchange). While this may seem to be obvious, the evaluation often times is under-appreciated and key predictors of present and possibly future activity tolerance are missed. When a parent complains that their child cannot keep-up, the provider can use these concepts to follow and discuss the issue in an organized fashion. This also provides a base for longitudinal evaluations regarding activity/exercise tolerance.
Chronotropy is not only about the heart rate appropriate for age and activity level, but is the heart rate variability that is expected to meet the changing metabolic demand at all levels of activity [27–34]. The autonomic nervous system function can be assessed in clinical settings by measuring resting heart rate (HR), heart rate variability (HRV), or heart rate recovery-reserve (HRR) following exercise. During the last decade, heart rate variability (HRV), that is, a marker of parasympathetic heart rate (HR) modulation has also been used in exercise physiology to evaluate the fitness level and the physiological responses to physical activity. Regulating cardiovascular function to satisfy the metabolic demand of working muscles, the autonomic nervous system has been investigated during recovery after physical exercises performed at different intensities. Low heart rate variability, especially during sleep suggests lack of autonomic input or responsiveness resulting in decreased cardiac output and places burden for activity on inotropic response [35]. Chronotropic and cardiopulmonary inefficiency in general may be elucidated in part by a sleeping overnight oximetry study. Some information can also be derived from holter monitors. Overnight oximetry can identify periods of poorly compensated hypoxemia and or excessively high or low heart rate variability. Overnight oximetry may also provide information about autonomic modulation as periods of sleep require activation of the sympathetic and parasympathetic systems. Inefficiencies in this output especially in the face of transient hypoxemia-desaturations can demonstrate chronotropic demand problems. When desaturations occur, the auto-resuscitation response should consist of increase heart rate and minute ventilation. In addition, there should be an arousal response to hypoxemia-desaturation. Absence of any these responses is a sentinel for possible abnormal or immature autonomic loop-gain processes and these mechanisms are important for development of activity tolerance. Increased oxygen consumption associated with activity requires modulation of heart rate and changes in vascular resistances. Failure of these mechanisms results in decreased perfusion to areas most involved in the activity and decreased functional capacity. While seemingly simple, overnight oximetry and especially polysomnography is a physiologic window into the capacity for activity-exercise as sleep is not a period of energy storage or biophysical restoration, but a period of reorganization and growth that requires energy consumption and strategic delivery by changing vascular flows to specific areas of the body [34]. These simple techniques are easily performed (especially overnight-sleeping oximetry) but are not well understood and interpreted by clinicians. The difficulty in interpretation is really made more challenging by the quality of the recording and this is very dependent on the type of pulse oximeter used. The signal-to-noise ratio and signal variance and toleration of movement are important to consider in regards to obtaining an optimal signal for study. This is especially true of probe and placement on the body. For example, placement of digits may not be the best choice and often times the headband probe delivers a more consistent signal. In summary, there are no gold standard assessments of activity intolerance in infants and toddlers with the exception of observation, examination, and vital signs. There are pulmonary functions that can provide some information that are discussed in other chapters of this text book. Measurement of HRV by overnight oximetry and polysomnography may be of some value but there are no studies to provide any evidence based recommendations. The most important part of this evaluation is to potentially identify those children with the potential for activity intolerance and at risk for long-term sedentary behavior. This allows the clinician to develop a long-term strategy for evaluation and intervention which ultimately can impact the future health of these patients.
Early School Age
School-age children become much more “in tune” with their exercise or activity based symptoms and can give some age level description of potential problems. One technique that can be used in 5–6 year olds is to have them draw or select an illustration or picture of what happens when they participate in exercise or activity [36]. We use an I-pad or other tablet with a standard stylus when possible, but paper and pen work as well (Fig. 12.6). Use of an I-pad or tablet device allows us to annotate on the picture and makes it easy to import pictures into a report. Child participation allows the clinician to begin probing into more specifics and may also trigger responses from parent(s) which can add to or create confusion regarding the true issue. Well meaning parents can present their biases into the symptoms and signs which can often lead the clinician to a conclusion that the parent is in part facilitating the complaint or may have push levels of activity and skill (training) upon the child which is not age appropriate. The opposite may also occur where parents limit the child’s activity for fear of inducing or triggering an event or the assumption that the child cannot be active. In a way, the parent may be indirectly participating in the limitation or they may just be uninformed or have been given incorrect information by others. Pushing children and adolescents to unachievable levels of physical activity occurs at all ages and is the most common cause of exercise intolerance and avoidance of exercise in pediatrics [37]. Sports specific specialization with development of skill really occurs during teenage years. The amount of practice in early age groups does not necessarily increase the probability a child will become a performance level athlete in a particular sport. Furthermore, teens that participate later in sport specialization can make up for less practice time and experience [38]. Unfortunately, the clinician cannot often openly express their concern that the patient-child is being asked to participate at a level far above (overtraining) or below their physical age. This conclusion requires clinicians to investigate all physical causes first which may require some objective testing (CPET or ACPET) which can show parents that biomechanics and physiology of exercise at their child’s age level are very intact and the child is capable of engaging in some form of physical activity. The opposite may occur where pathology is demonstrated as previously reviewed. This also allows clinicians to develop a prescription plan which involves parental participation. This objectified evaluation and prescription is the core principle which allows us to promote healthy development in our children.
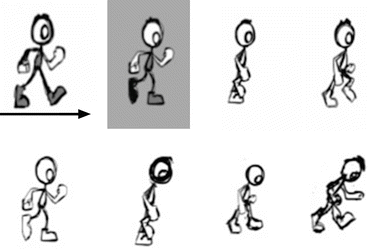
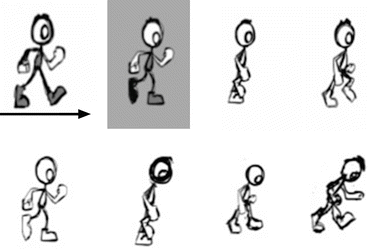
Fig. 12.6
PCET. Pictorial exercise intolerance screening using an tablet device. The child pick the animation that best describes how they feel during exercise. Which one is you when chasing your friend. The top (green) pictures show the animation increasing in power while the lower panel is consistent with decreasing power.
Initial Evaluation to Determine the Study Type and Procedure in School Age Children, Adolescents; Including those Participating in Organized Sports
The initial evaluation of a child or adolescent and/or caregiver complaining of physical activity intolerance is to define the characteristics of the observed or perceived events. This physical activity history assists in the study design and directs a purpose for the evaluation. The indications for CPET or other types of field exercise capacity testing (ACPET) are listed in Tables 12.7 and 12.8. Once again, the ultimate goal is to provide the patient with an individualized exercise evaluation, plan, medical intervention, psychological counseling, and educational training to counter-balance their limitations and/or pathology. Secondary goals are to assist in longitudinal planning for interventions by assessing functional capacity at intervals to provide information about exercise training or disease progression. Longitudinal exercise evaluations are often used to (1) assess the effectiveness of exercise training programs, (2) plan surgical interventions (transplantation-lung volume reduction-bariatric surgery), (3) assist in evidence based interventions for chronic diseases, (4) evaluation of occupational injury and (5) for research development of new therapies or training modalities. CPET can be used to reassure the patient and/or parent that the biomechanics and cardiopulmonary systems are functioning well or demonstrate pathophysiology. CPET is also used to assist the patient and family toward developing healthy attitudes about the quantity and quality of the patient’s physical activity/exercise program (i.e., frequency, intensity, time, and type (Table 12.9). Reassuring the parents and patients that the physiological systems are working efficiently will allows the clinician to investigate deeper into the physical activity/sport execution-performance, attitudes of physical activity, and sport-specific movements and expectations. Addressing these issues can be challenging and our laboratory asks the will often ask parents to not be present during the study, CPET or other challenges are performed. Video recording the study can be helpful allowing the laboratory technicians and clinicians to review the events (or the absence of events) with parents and concerned coaches and trainers. We also ask parents and observers to video events they observe in real time at sporting events or during physical activity events. The team trainer and or physician should also be asked to record their observations. Video is a valuable tool and in some circumstances, we provide relatively inexpensive video cameras for home if smart phones are not available. The videographer is asked to share the files by uploading the movie to a secure site or we download the device in the office or lab. In some difficult high profile cases or circumstances clinicians should try if possible to attend the sporting event to make observations and perhaps plan interventions. Coaches are usually very willing to grant access to sidelines and welcome input. This is especially true in performance athletes whom are candidates for scholarships, participate in professional or collegiate athletics or are Olympians. Most training sessions and events (competitions) at this level are recorded and with permission the videographers that film these events are happy to provide footage of the observations. It is important the exercise team obtains written consent from the participant, parent or guardian, coaches, and institution. Patient confidentiality and consent must be followed as the ramifications of disclosure of this information can have significant monetary and psychosocial consequences. One of the most important aspects when dealing with any competitive athlete is to be positive when addressing the issues. Instilling confidence that the exercise limitation is solvable or self-limiting is extremely important for the success of any intervention. Performance athletes are often suspicious and susceptible to negative thoughts or demeanor. Negativity will not be received well by the patient and definitely not by good coaches. Persistence of negativity by the coaching staff and/or parents may in itself be a cause of perceived exercise limitation from overtraining or sports related anxiety. Always ask the coaches, parents or guardians, and patient about recent head injury or symptoms of sports related concussion regardless of the sport. While these recommendations may seem excessive, the purpose for collecting data in this manner is to be thorough, to instill confidence in the patient and family and be positive that exercise is possible in anyone with the right modifications.
Table 12.7
Indications for cardiopulmonary exercise testing
Evaluation of exercise tolerance check sheet (check all that apply) |
---|
□ Determination of functional impairment or capacity (peak VO2) |
□ Determination of exercise-limiting factors and pathophysiologic mechanisms |
□ Evaluation of undiagnosed exercise intolerance |
□ Assessing contribution of cardiac and pulmonary etiology in coexisting disease |
□ Symptoms disproportionate to resting pulmonary and cardiac tests |
□ Unexplained dyspnea when initial cardiopulmonary testing is nondiagnostic |
□ Evaluation of patients with cardiovascular disease |
□ Functional evaluation and prognosis in patients with heart failure |
□ Selection for cardiac transplantation |
□ Exercise prescription and monitoring response to exercise training for cardiac rehabilitation |
□ Special circumstances; i.e., pacemakers |
□ Evaluation of patients with respiratory disease |
□ Functional impairment assessment (see specific clinical applications) |
□ Chronic obstructive pulmonary disease |
□ Establishing exercise limitation(s) and assessing other potential contributing factors, especially occult heart disease (ischemia) |
□ Determination of magnitude of hypoxemia and for O2 prescription |
□ When objective determination of therapeutic intervention is necessary and not adequately addressed by standard pulmonary function testing |
□ Interstitial lung diseases |
□ Detection of early (occult) gas exchange abnormalities |
□ Overall assessment/monitoring of pulmonary gas exchange |
□ Determination of magnitude of hypoxemia and for O2 prescription |
□ Determination of potential exercise-limiting factors |
□ Documentation of therapeutic response to potentially toxic therapy |
□ Pulmonary vascular disease (careful risk–benefit analysis required) |
□ Cystic fibrosis |
□ Exercise-induced bronchospasm |
□ Specific clinical applications |
□ Preoperative evaluation |
□ Lung resectional surgery |
□ Lung volume resectional surgery for emphysema |
□ Exercise evaluation and prescription for pulmonary rehabilitation |
□ Evaluation for impairment–disability |
□ Evaluation for lung, heart–lung transplantation |
□ Evaluation of performance athletes or sports participants |
□ Other ______________________________________ |
Signature: |
Table 12.8
Contraindication review checklist
Absolute (adult) checklist (check all that apply) |
---|
□ Changed resting ECG suggestive of ischemia—recent |
□ Infarction in past 48 h |
□ Unstable angina-chest pain |
□ Uncontrolled arrhythmias
![]() Stay updated, free articles. Join our Telegram channel![]() Full access? Get Clinical Tree![]() ![]() ![]() |