Cardiopulmonary and Cardiocerebral Resuscitation: Introduction
Cardiac arrest may be defined as the abrupt cessation of cardiac function leading to a precipitous decrease in cardiac output and ensuing circulatory collapse. Sudden cardiac death is a leading cause of mortality, with three million annual deaths worldwide and more than 300,000 annual deaths just in the United States.1,2 One-quarter of these patients are younger than 65 years of age.3 The most common cause of sudden cardiac death remains out-of-hospital cardiac arrest (OHCA). Ventricular fibrillation (VF) is a common dysrhythmia that presents as cardiac arrest and is the most common malignant arrhythmia in adults with OHCA.2 As expected, diagnosis and management of this lethal disease has remained a priority for decades, with cardiopulmonary resuscitation (CPR) being advocated as a dependable treatment for OHCA. The CPR guidelines were established in 1966, and the most recent version was released in 2005.4-8 Nevertheless, the reality remains that survival rates from OHCA are abysmal, hovering around 1% to 7% in the major cities of the United States despite repeated changes in the emergency cardiac care (ECC) guidelines.
This chapter outlines our current state of understanding of the disease process and the management strategies that are currently available to us. We will also discuss a new therapeutic modality: cardiocerebral resuscitation (CCR), which has recently been shown in some reports to have improved outcomes compared with traditional CPR. CCR emphasizes uninterrupted chest compressions as a priority but offers an inclusive alternative that attempts to enhance survival from this lethal disease.
Historical Background
The initial attempts to treat cardiac arrest focused on chest compressions. Closed chest defibrillation and closed chest cardiac massage were first described in the 1960s.9-11 These initial reports suggested survival rates in excess of 70%; therefore, they were rapidly adopted for in-hospital cardiac arrests and OHCAs.4 Interestingly, in this initial publication, some patients were treated with chest compression without positive pressure.9 However, with time, ventilation gradually became ingrained as an essential pillar of cardiopulmonary resucitation.12 There were several reasons that positive-pressure ventilation was touted as being indispensable to CPR:
Volunteers who underwent respiratory arrest with temporary administration of paralytics were noted to have rapid deterioration of the blood gases.13
Gasping was not thought to be common in patients with cardiac arrest.
There was an assumption that the lay public could not reliably distinguish respiratory arrest from cardiac arrest (and would therefore fail to ventilate the victim who did undergo respiratory arrest).
Historical data derived from resuscitation attempts on drowning victims.12
Given the prevalence and lethality of the disease, the American Heart Association (AHA) spearheaded the dissemination of CPR and ECC information to health care professionals and the lay public in the 1970s. In 2000, the Guidelines for CPR and Emergency Cardiac Care were published by the AHA in collaboration with the International Liaison Committee on Resuscitation (ILCOR).6 It was the first time that national and international guidelines were identical. Guidelines were again agreed upon and published in 2005, although separate statements were issued by the AHA and ILCOR.7,8
Despite the rapid distribution of this information and the utilization of CPR techniques, survival from OHCA remained disappointingly static. A 2005 report revealed a rate of neurologically intact survival from OHCA of 1.4% in Los Angeles, similar to survival rates in Chicago, New York City, and Detroit.14,15 These numbers are substandard and more importantly are a powerful argument that CPR as a treatment for sudden cardiac arrest is not optimal. However, other cities have much better survival rates; these are areas that have a high incidence of bystander CPR and rapid emergency medical systems (EMS response times).16
The Pathophysiology and Hemodynamics of Cardiac Arrest
In adults, the common causes of respiratory arrest include drowning, drug overdoses, neuromuscular diseases, suffocation, carbon monoxide poisoning, and airway obstruction. Respiratory arrest leads to arterial hypoxemia, myocardial hypoxia, and consequent secondary cardiac arrest. This secondary cardiac arrest usually manifests as pulseless electrical activity (PEA) or asystole. Resolution of the arterial hypoxemia would treat myocardial hypoxia and improve chances of resuscitation. A rapid application of this principle is in the setting of a ‘café coronary‘. Here aspiration of a large piece of food results in obstruction of the trachea and alveolar hypoxia secondary to absent ventilation. The Heimlich maneuver (Henry J. Heimlich MD, Xavier University, Cincinnati, Ohio), or similar maneuver rapidly increases the intrathoracic pressure, causing a forceful expulsion of air from the lungs, leading to a relief in the obstruction. On a similar note, temporary assisted ventilation may be all that is necessary in a patient with respiratory arrest secondary to an opiate drug overdose.
However, when secondary cardiac arrest has set in for an extended period, it becomes the dominant factor affecting the patient’s survival. At this point, both chest compression and assisted ventilation are needed. In a swine model of pediatric asphyxia, Berg et al17 produced hypoxic arrest by clamping the endotracheal tube until the loss of arterial pressure, a process that took a variable period of time averaging 9 minutes. In this study, the swine with hypoxic arrest were randomized to one of four different interventions: (1) chest compressions plus ventilations, (2) chest compressions only, (3) assisted ventilations only, or (4) no CPR. Arterial blood gas oxygen saturation after 1 minute of intervention was 87% ± 6% in the chest compressions plus assisted ventilations group compared with only 17% ± 5% in the chest compression only group. The 24-hour neurologically normal survival was 7 of 10 for the group receiving both chest compressions and ventilations, but only 1 of 14 for those treated with chest compressions only.17 These results support the assertion that chest compression alone is ineffectual in patients who undergo respiratory arrest and secondary cardiac arrest. In another experimental animal model, resuscitative measures were instituted early on in the course of respiratory arrest, when the peak systolic aortic pressures had decreased to only about 50 mm Hg. Survival was similar with chest compression plus ventilation, chest compression only, or assisted ventilation only, but each was significantly better than no CPR.18 Last, in a pediatric population of respiratory arrest, whereas 13.6% who received bystander CPR survived and were neurologically normal, only 2% of patients who did not receive bystander CPR before EMS arrival survived.19 Evidently, any resuscitative attempt is better than no attempt, even if it just chest compression. Hence, the contention of the AHA guidelines to “do something, as something is better than nothing.”7
Children comprise a special population because respiratory arrest encompasses the majority of cardiac arrests in children. This distinction is important because children seldom have underlying coronary artery or (primary) cardiac disease. Therefore, in a case of pediatric cardiac arrest, restoration of oxygenation assumes paramount significance, and ventilation must be established as a priority. However, primary cardiac arrest can occur in young individuals with conditions such as prolonged QT syndrome, arrhythmogenic right ventricular dysplasia, Brugada syndrome, anomalous coronary arteries, or commotio cordis, in which a blow to the chest wall timed at the peak of the electrocardiographic (ECG) T wave may result in VF. These children will experience primary cardiac arrest and should be managed as such.
On the other hand, in adults, most cardiac arrests are of primary cardiac origin, with a large percentage of them being VF or pulseless ventricular tachycardia (VT)2, usually a consequence of myocardial ischemia secondary to coronary artery disease (CAD). This malignant arrhythmia results in uncoordinated myocardial contractions; cessation of the cardiac pump function; and an instantaneous decrease in stroke volume and cardiac output and therefore systemic perfusion. One minute into persistent VF, coronary blood flow (myocardial perfusion) declines to zero, and by 4 minutes, carotid blood flow (cerebral perfusion) is also nil.20
In the absence of resuscitative efforts, progressive equalization of arterial and venous pressures occurs, leading to circulatory standstill. Some degree of antegrade blood flow will continue until equalization of systemic pressures in the arterial and venous systems takes place, the so-called mean circulatory filling pressure.21 In animal models, it takes about 5 minutes for shift of the arterial blood into the capacitance venous system.20 The morphologic result of this phenomenon is the acute distension of the right ventricle (Fig. 50–1). The acute increase in right ventricular volume and the consequent initiation of ventricular interdependence physiology lead to decreased left ventricular preload and therefore stroke volumes. Progressive decrease in left ventricular volumes occurs until a state of extreme myocardial contraction develops. These changes have been nicely demonstrated by magnetic resonance imaging (MRI) in closed chest animal models of VF.22–24 The end stage of this ischemic contractile state of the left ventricle has been described as “stone heart” (Fig. 50–2).
Figure 50–1
Magnetic resonance images of swine chest at baseline and during the first minute of ventricular fibrillation (VF). Note that the right ventricular (RV) volume increases by approximately 50% within 1 minute of the onset of VF. From Sorrell VL, Bhatt RD, Berg RA, et al. Cardiac magnetic resonance imaging investigation of sustained ventricular fibrillation in a swine model: with focus on the electrical phase resuscitation. Resuscitation. 73(2);279-286. 2007.
Figure 50–2
Magnetic resonance images of swine chest at baseline and periodically until 30 minutes of cardiac arrest as a consequence of ventricular fibrillation. Note the development of “stone heart.” LV, left ventricle; RV, right ventricle. From Sorrell VL, Bhatt RD, Berg RA, et al. Cardiac magnetic resonance imaging investigation of sustained ventricular fibrillation in a swine model: with focus on the electrical phase resuscitation. Resuscitation. 73(2);279-286. 2007.
In these patients with a primary arrhythmogenic cardiac arrest, the pulmonary alveoli and the left heart are filled with oxygenated blood that does not require immediate replenishment. The circulatory standstill ensures that there is no peripheral tissue extraction of oxygen and the alveoli and the arterial blood remains replete with oxygen, without any discernible A–a (alveoli to arterial) gradient. Moreover, some of these patients continue to gasp for a brief period of time postarrest because the medullary respiratory center is still active and continues to fire. This is responsible for the gasping phenomenon seen in up to 50% of individuals with OHCA.25 They stop gasping only when the respiratory center finally succumbs to hypoxic injury and shuts down its phasic discharge activity. This is the theoretical rationale for relegating ventilation as a distant secondary target in CCR.
Treatment of patients with circulatory standstill is accomplished by closed chest compressions. Chest compression halts the progression of circulatory arrest and its downstream consequences by providing phasic arterial blood flow. Of the factors identified as being responsible for forward flow with chest compression, perhaps the most important is the duration between the onset of VF arrest and initiation of chest compressions.26 Other important factors are the technique used for chest compressions and the patient’s chest wall configuration.27 It is important to remember that even with optimal chest compressions, total coronary flow is only around 20% to 40% of pre-arrest values.
Two distinct theories attempt to explain the mechanism by which chest compression manages to provide systemic perfusion. When closed-chest cardiac massage was introduced in the 1960s, it was assumed that the mechanism of blood flow was much the same as with open-chest cardiac massage, wherein the heart was grasped in the hand and the flow was produced by squeezing the fibrillating left ventricle. According to the cardiac compression theory, direct compression of the left and right ventricles between the sternum and the vertebral column creates a pressure gradient between the ventricular cavity and the great vessels. This pressure gradient closes the mitral and tricuspid valves and ejects blood forward out of the ventricles. The ventricles then refill during the decompression phase.
This theory suffered a setback when a study in humans at Johns Hopkins University suggested that the mechanism of blood flow during chest compression was the thoracic pump theory.28 According to this theory, external chest compression increases intrathoracic pressure, forcing blood to flow from the thorax. Retrograde flow from the right heart to the systemic veins is prevented by the jugular venous valves at the thoracic outlet. According to this model, the heart acts merely as a passive intermediary without having a pump function. The hypothesis that the thoracic pump mechanism was of major importance gained traction with studies that showed that patients in cardiac arrest were able to stay awake by repetitively coughing and thereby moving blood through the circulation, simply based on their intrathoracic pressure fluctuations.29,30 A major criticism of the Johns Hopkins study was that it was performed late in cardiac arrest after the house staff had decided that resuscitation was unlikely.28 Thus, these hemodynamic measurements were made after prolonged cardiac arrest, when one would expect the natural progression to the “stone heart.”22,31 At this advanced stage of cardiac arrest, the thoracic pump is the likely mechanism for the blood flow, but successful resuscitation and survival rates are extremely low.
Transesophageal echocardiography has shed further light on this debate. A study in humans undergoing cardiac arrest and CPR has shown that both mechanisms are in play—whereas the cardiac compression mechanism was more likely to be seen in patients studied early on after the arrest, the thoracic pump mechanism predominated when cardiac arrest patients were studied later.32 As expected from the temporal trends, survival only occurred among those studied early, in whom the cardiac compression mechanism was active; there were no survivors among those who demonstrated the thoracic pump mechanism.32 It is plausible that in younger individuals with compliant chest walls or narrow anterior posterior chest dimensions, the cardiac pump is the predominant mechanism and that they are more likely to be resuscitated. In patients with severe emphysema and a “barrel chest,” the mechanism of blood flow is likely because of the thoracic pump mechanism, but these patients are also unlikely to be revived by closed-chest compressions.
The true means of forward blood flow during closed-chest compression resuscitation is of considerable significance because guidelines for CPR have been propounded based on what was considered to be the predominant operative mechanism. The “thoracic pump” theory led to the 1980 AHA guidelines that recommended a chest compression rate of 60 per minute. The guidelines stated that “compression should be sustained for 0.5 second, with relaxation given for equal period.33” In hindsight, this was a failure because it resulted in too few compression per minute for single rescuers to successfully resuscitate cardiac arrest victims. This recommendation of 60 compressions per minute was not readily accepted by many observers. Investigators from The University of Arizona and Duke Medical Schools performed studies in animals and found that the optimal chest compression rates for survival from VF arrest were 80 to 120 per minute.34 Because a chest compression rate of 120 per minute was thought to be too fatiguing for the general public, the 1992 AHA guidelines compromised and recommended rates of 80 to 100 per minute.5 The 2005 guidelines recommended a compression rate of 100 per minute.
In some cases of optimal CPR, either manual or with mechanical devices, the forward blood flow is so good that the patient awakes, and sedation is necessary. This needs to be emphasized because all too often when the patient begins moving, manual chest compressions are stopped until the patient becomes unresponsive. This is a potentially perilous action that could have serious consequences for the patient’s survival.
Coronary perfusion pressure (CPP) is defined as the difference between the aortic and right atrial pressure during the release phase (“diastole”) of closed chest cardiac compression. It is the pressure gradient that is responsible for the flow of blood into the coronary tree. This pressure is built up slowly with chest compressions, such that the first compressions do not generate a significant CPP, but the last compressions do35 (Fig. 50–3). The CPP is a surrogate for myocardial perfusion and has been shown in animal models to be a determinant of survival in prolonged VF36-39 (Fig. 50–4). When chest compressions are interrupted (for rescue breathing, tracheal intubation, placement of an intravenous (IV) line, or even rhythm and pulse analysis), the CPP decays and, with it, the chances for survival. This gradient and therefore the CPP have to be reestablished again when compressions are restarted (Fig. 50–5). This is a key argument in understanding why continuous chest compression (CCC) is better in primary cardiac arrest. It addresses two important issues pertinent to CPPs: (1) the gradient, after it is established, does not have to be reestablished and (2) coronary perfusion is maintained better without interruption of chest compressions. Cardiocerebral resuscitation improves survival because the perfusion pressures are constantly preserved. The same principle extends to cerebral perfusion and CCR and by extension neurologically intact survival.
Figure 50–3
Simultaneous aortic (red) and right atrial (blue) pressure tracings from a swine undergoing the initiation of continuous chest compressions for cardiac arrest as a consequence of ventricular fibrillation. Note that with the initiation of chest compressions, it takes some time for the coronary perfusion pressures (aortic diastolic minus the right atrial diastolic pressure) to increase. The chest compression rate is 100/min.
Figure 50–4
Coronary perfusion pressure (CPP) was predictive of survival in this experimental dog protocol. CPP was higher in 24-hour survivors compared with animals that were resuscitated but subsequently expired. From Kern KB, Ewy GA, Voorhees WD, Babbs CF, Tacker WA. Myocardial perfusion pressure: a predictor of 24-hour survival during prolonged cardiac arrest in dogs. Resuscitation. 1988;16:241-250.
Figure 50–5
Simultaneous aortic (red) and right atrial (blue) pressure tracings from a swine undergoing cardiopulmonary resuscitation during prolonged ventricular fibrillation arrest. Two ventilations are provided for every 15 chest compressions. There is a realistic 16-second interruption of chest compression to simulate a single bystander delivering “rescue breathing.” The chest compression rate is 100 per minute. Notice how the coronary perfusion pressure builds up and then drops when chest compression is interrupted. It must be built up again with the next round of chest compressions.
Survival rates decrease by about 7% to 10% for every additional minute that a patient remains in VF.6 After about 12 minutes, defibrillation for VF is rarely effective in the absence of chest compressions.6 The three-phase concept for VF, proposed by Weisfeldt and Becker39a in 2002, elegantly explains the pathophysiologic reasons behind this survival trend. This model divides VF into an electrical phase (~ 0-4 minutes), circulatory phase (~ 4-10 minutes), and a metabolic phase (>10 minutes). The first two phases were initially described by Kouwenhoven,40 who pointed out in his animal experiments that defibrillation was successful if applied during the first 2 or 3 minutes.
In this early phase of VF, there is enough myocardial adenosine triphosphate (ATP) reserve that defibrillation alone (without chest compressions) is adequate to restore a perfusing rhythm. The most well know application of this tenet is the implantable cardioverter-defibrillators (ICDs) that deliver therapy within seconds of an unstable VT or VF and are accordingly highly effective. Automated external defibrillators (AEDs) are also exceptionally advantageous in the electrical phase of VF. Public access AEDs have demonstrated dramatic improvements in survival in patients who have collapsed in casinos or airports.41,42 These patients are defibrillated within minutes because of their proximity to an AED and because they are in the electrical phase, often return to a perfusing hemodynamically stable rhythm. It should be noted that the duration of the electrical phase can be prolonged by initiation of bystander CPR.43 In Seattle, Washington, the average EMS response time is 5 minutes, with a bystander CPR rate of 67%; this translates to patients being defibrillated in the electrical phase and consequently having some of the best survival rates from OHCA anywhere in the United States.16
Conversely, in the circulatory phase of VF, the persistent and ineffectual myocardial contractions coupled with the now prolonged lack of coronary blood flow results in depletion of the myocardial high-energy phosphate stores. In addition, prolonged tissue hypoxia results in lactic acidosis at the cellular level. Electrocardiographically, this is manifested by the decreasing amplitude of the VF wave form with a transition to “fine” fibrillatory waves on the ECG.44 ECG analysis of spectral and waveform characteristics45,46 and proteomic signaling markers47 may help in deciphering if the patient is actually in this phase of VF. Defibrillation in the absence of chest compressions is rarely successful in this phase because even if the VF is terminated, the resultant rhythm is PEA or asystole.48 Successful resuscitation in this phase requires the restoration of myocardial energy stores. It is here that chest compressions play a pivotal role in restoring some degree of coronary (and cerebral) perfusion. Reestablished coronary flow presumably results in the formation of new myocardial energy stores and makes the myocardium more responsive to defibrillation. Morphologically, postcompression VF waveforms show an increase in amplitude, a higher median frequency, and a “coarser” rhythm.44 VF mean and median frequencies have been shown to be predictors of successful defibrillation49 and are associated with neurologically intact survival.50
Most OHCA patients are found in the circulatory phase of VF upon arrival of EMS personnel. For instance, the average time to response in the city of Tucson, Arizona, is 6 minutes, 34 seconds,51 placing the patient precisely in the circulatory phase and mandating initial chest compression. A defibrillation-first strategy in these patients would likely lead to a refractory rhythm such as PEA or asystole.48,52 Pre-shock chest compressions have been demonstrated to increase the likelihood of successful defibrillation in a swine model.50 A randomized study from Seattle in humans showed increased survival when chest compressions were uniformly performed for 90 seconds before defibrillation.53 A more recent study from the Resuscitation Outcomes Consortium also confirmed improved survival in humans with pre-shock chest compression.54 Finally, a Norwegian report showed that compared with standard care for VF, CPR before defibrillation offered no advantage in improving outcomes for this entire study population or for patients with ambulance response times shorter than 5 minutes (electrical phase). However, the subset of patients with VF and ambulance response intervals longer than 5 minutes (circulatory phase) had better outcomes with CPR before defibrillation.55
This third and terminal phase is universally associated with diminishing odds of successful defibrillation and neurologically intact survival. End-organ damage has already set in with irreversible cellular impairment. Ischemic and reperfusion injuries are believed to predominate at this stage. Strategies that may delay the onset of this phase of irremediable damage include mild therapeutic hypothermia. However, with the possible exception of young, healthy patients and cold water drowning, very few patients survive after more than 15 minutes of untreated cardiac arrest.
One of the limitations of the model is that accurate determination of the VF phase at the time of resuscitation. This becomes easier if the cardiac arrest is a witnessed event. Nonetheless, most OHCA patients receive resuscitation in the circulatory phase, hence the justification for restoration of coronary perfusion and myocardial oxygenation with chest compressions before attempted defibrillation.
These rhythms are important to recognize because they are not amenable to electrical defibrillation therapy. When VF is the initial rhythm, the chance of survival is significantly higher. In a review of more than 49,000 patients from the National Registry for Cardiopulmonary Resuscitation (NRCPR), having a shockable rhythm (VF or pulseless VT) was significantly associated with survival.56 Although VF in an adult is persuasively associated with CAD, PEA, and asystole usually are not. The exception is when they are seen in the terminal phase of VF arrests. When VF is defibrillated in the circulatory phase without predefibrillatory chest compressions, these postdefibrillation rhythms are usually PEA.48,52 Predefibrillatory and postdefibrillatory chest compressions have been shown to be effective in preventing the emergence of post-VF PEA.52
One has to consider secondary causes of cardiac arrest when PEA is otherwise the primary rhythm—the established five Hs (hypovolemia, hypoxia, hydrogen ion [acidosis], hyperkalemia or hypokalemia, and hypothermia) and the five Ts (tension pneumothorax, tamponade, tablets [drug overdose], thrombosis coronary, and thrombosis pulmonary).7 Identifying and remedying the underlying cause are vitally important in the successful resuscitation of these patients.
Asystole is frequently a secondary and typically a terminal rhythm. It is commonly a result of both non-intervened PEA and VF. Patients often have longer duration of untreated VF and consequently a poorer prognosis. The same causes of PEA can also sometimes present initially with asystole and should therefore be investigated and treated if found. Recognition of Life Extinct (ROLE) guidelines in England and Wales deem 20 minutes of asystole, despite advanced resuscitative measures, as grounds for termination of resuscitative efforts.57
Two possible pitfalls in diagnosing and treating asystole are (1) VF masquerading as asystole58 (2) and fine VF mistaken to be asystole.46 In these cases, it would be sensible to make sure that the cables are connected and the gain is turned up and to look at other ECG leads (especially perpendicular leads) to see if the VF pattern can be discerned. If the ECG leads are not available and fine VF is suspected, the defibrillatory paddles can be turned by 90 degrees to confirm that it is not VF. If fine VF is suspected, the arrhythmia should be defibrillated. There is no credible evidence that a mistake here (ie, defibrillation of asystole) negatively affects the patient’s chance of survival.
These rhythm trends are reversed in the in-hospital setting. In a prospective observational study from the National Registry of Cardiopulmonary Resuscitation (NRCPR), 51,919 patients were studied.59 The first documented pulseless rhythm was VT in 7%, VF in 17%, PEA in 37%, and asystole 39%. However, survival to hospital discharge was still more likely after first documented VT or VF than PEA or asystole.59
Gasping is common after the onset of cardiac arrest. It can be found in 39% to 55% of cardiac arrest victims, and the presence of gasping has been found to correlate with survival.25,60 Others have shown similar results in the animal laboratory.61-63 Animal research laboratories that use paralysis as part of the protocol when studying CPR eliminate this physiologically important reflex, which may be one reason that some investigators have not found improved survival with chest compression–only approaches to cardiac resuscitation.
When gasping occurs with OHCA, it is both fortunate and unfortunate. It is fortunate because if chest compressions are promptly initiated, the subject is likely to continue to gasp and provide self-respirations. However, gasping may be unfortunate because most lay individuals interpret this as an indication that the individual is still breathing. Accordingly, they are likely to neither initiate bystander CPR nor activate the EMS as soon as they should. Public education on this issue is essential for improving prompt initiation of bystander chest compressions. Unfortunately, about 20% of patients who gasp are thought to be not in cardiac arrest and end up not receiving chest compressions.64
The 2005 AHA and Ilcor Guidelines
7,8The significant changes that were instituted in 2005 compared with 2000 are summarized in Table 50–1.
2000 Guidelines | 2005 Guidelines | ||
---|---|---|---|
1 | Alerting EMS (only children) | Phone EMS first and then do CPR | CPR for 2 min and then call EMS |
2 | Unwitnessed adult | Shock first | 200 CC, then shock |
3 | Rescue breath | Take a deep breath | Take a normal breath |
4 | Rescue breath duration | 1–2 s | <1 s |
5 | Ventilation rate | 12-15/min | 8-10/min |
6 | CC Ratio | 15:2 | 30:2 |
7 | Two-person CPR | Switch when fatigued | Every 2 min or 5 cycles (150 CC) |
8 | Defibrillation | Three shocks without CC | One shock followed by CC |
9 | Defibrillation energy | Three monophasic stacked | 360 J monophasic or 150-200 J biphasic |
10 | Postdefibrillation | Pulse and rhythm analyses | Immediate CC |
11 | Intubated patients | Pause CPR to give breaths | Give breaths during CC |
12 | Drug delivery | Drug–CPR–shock | Drugs not to interrupt CC |
13 | High-dose epinephrine | May be used | Not recommended |
14 | Hypothermia | Not recommended | Recommended for VF arrest |
The chief changes of 2005 American Heart Association Guidelines for Cardiopulmonary Resuscitation for lay rescuers were to start CPR as soon as collapse occurs, activate the EMS system by calling 911, and use an AED if available. Lay rescuers should push hard, push fast, allow full chest recoil, and minimize interruptions of chest compressions. Specific 2005 guidelines changes included the recommendation of “a single (universal) ventilation: chest compression ratio of 2:30 for single rescuers of victims of all ages (except newborn infants).” This recommendation is designed to “simplify teaching and provide longer periods of uninterrupted chest compressions.” The 2005 guidelines recommendation is for each breath to be administered within 1 second and delivered with enough volume to cause a visible rise in the anterior chest wall. In certification sessions, it is stressed that administration of the two rescue breaths should be completed quickly, interrupting chest compression for only 10 seconds. Although this recommendation is well intended to minimize interruptions of chest compression, providing two respirations without interrupting chest compressions for more than 10 seconds is nearly unachievable for a single lay rescuer.65,66
Minor changes in the 2005 guidelines include the elimination of the recommendations that lay individuals assess the pulse or check for other for “signs of circulation” before initiating CPR. The recommended hand position for chest compressions is also simplified and is “the center of the chest at the nipple line.”
Several important changes were made in the 2005 guidelines for advanced cardiac life support (ACLS). Chest compression before defibrillation results in improved survival during the circulatory phase of ventricular fibrillation. Therefore, the 2005 guidelines recommend that “Emergency Medical Services (EMS) providers may consider the provision of about 5 cycles (or about 2 minutes) of CPR before defibrillation for unwitnessed arrest, particularly when the interval from the call to the EMS dispatcher response at the scene is more than 4 to 5 minutes.”
A single defibrillation shock (rather than the three stacked shocks previously recommended) is to be followed immediately by about 2 minutes of chest compressions before rhythm checks. Specifically, rescuers “should not check the rhythm or a pulse immediately after shock delivery—they should immediately resume CPR, beginning with chest compressions, and should check the rhythm after 5 cycles (or about 2 minutes) of CPR.”
The recommendation to administer only one shock followed immediately by CPR (beginning with chest compressions) instead of three stacked shocks for the treatment of ventricular fibrillation or pulseless VT is “based on the high first shock success rate of new defibrillators and the knowledge that if the first shock fails, intervening chest compressions may improve oxygen and substrate delivery to the myocardium, making the subsequent shock more likely to result in defibrillation.”
Studies show that a period of chest compressions to produce blood flow before and after defibrillation could improve defibrillation success and patient outcome.55,67 Also, these changes were made as a result of reports that have shown that excessive no-flow time can be consumed while AEDs analyzed the cardiac rhythm before and after shock delivery.68 Newer algorithms are being tested in which ECG analysis that can occur without the requirement for interruption of chest compressions, thereby potentially bypassing this problem in the future69.
After the implementation of the 2005 guidelines, OHCA survival rates were significantly higher in the postguidelines (9.4%) than in the preguidelines period (6.1%). Multivariate regression analysis in this observational study, adjusting for significant predictors of survival, showed that OHCA in the postguidelines period was associated with 1.8 greater odds of survival than in the preguidelines period.70 Nevertheless, a meta-analysis published in 2010 did not show any difference in survival.71
Return of spontaneous circulation (ROSC), being alive at hospital admission, or being alive at some later designated time were all used previously as criteria for survival for OHCA. Most such “survivors” ultimately died before being discharged from the hospital, making these definitions inappropriate. In addition, different definitions of survival made comparisons of different approaches to CPR difficult. A more clinically relevant definition of “survival” has been introduced: neurologically normal survival at hospital discharge or at a specified time after discharge for humans or neurologically intact survival at 24 or 48 hours for experimental animals.72,73
Both the 2000 and 2005 guidelines have placed a premium on human randomized, controlled trials.6,7 However, high-quality human subject randomized trials in the arena of cardiac arrest are few and far between because they are hampered by the requirements for blinding, randomization, and consent in the emergent setting of sudden death. Lower levels of evidence, including high-quality animal data, are often downgraded in these guidelines. Recommendations from previous guidelines are only changed by randomized, controlled trials, but the initial standards and guidelines were themselves not the result of randomized, controlled trials. This dichotomous approach has led to older recommendations being grandfathered in without robust evidence supporting their use.
Another disconcerting problem with the guidelines is the fixed schedule of the AHA and ECC guidelines, which are updated only every 5 to 8 years and are therefore unable to adapt to the rapid inflow of information in the field of resuscitation. One of the reasons for this is that the guidelines have tended to become more inclusive, thereby increasing the expense that goes into the development of these guidelines.
When definitions of survival such as neurologically normal or near normal are used, it is clear that survival rates for OHCA with cardiopulmonary resuscitation have been very low and, with the exceptions of early application of an AED, have remained stagnant over the past few decades.14 One study that did show improvement with the 2005 guidelines showed a survival of only 9.6% with the 2005 guidelines.70 However, a 2010 meta-analysis of more than 100,000 patients showed an aggregate survival rate of 7.6%, which has not significantly changed in almost 3 decades.71 In this study, predictors of survival were being witnessed by a bystander, being witnessed by EMS personnel, receiving bystander CPR, being found in VR or VT, or achieving ROSC.71
Most survivors of OHCA are found in the subset of patients with a bystander-witnessed arrest and a shockable rhythm on arrival of EMS with a defibrillator.74,75.In this subset of patients, survival in 2002 in Los Angeles was 6%; survival from 1992 to 2002 in Tucson, Arizona, averaged 10%, and survival in Rock and Walworth Counties, Wisconsin, during 2000 to 2003 was 20%.51,76 These survival differences probably reflect differences in EMS response times. A large Medicare database of more than 400,000 elderly patients revealed that even in-hospital survival after CPR has been unchanged for the period from 1992 to 2005.77
Abella et al78 reported that higher chest compression rates were significantly correlated with initial ROSC in in-hospital cardiac arrest. (Although survival rates were not reported, there would be no survival in those without ROSC.) In this study, ROSC was significantly higher for patients receiving more than 87 compressions per minute than those receiving fewer than 72 compressions per minute. In the out-of-hospital situation, in which a single bystander is performing CPR, if every set of 15 compressions is interrupted for 14 to 16 seconds to deliver the recommended two ventilations, the individual will not receive enough chest compressions for ROSC. In fact, if every set of 30 compressions is interrupted 14 to 16 seconds for single bystander CPR, the delivered chest compressions would still fall short of the near 90 compressions needed for ROSC in this in-hospital population. Several studies have suggested improved survival with greater compressions-to-ventilation ratios.72,79
The most troubling aspect of the 2005 guidelines has been its emphasis on ventilation. From their inception, the standards and guidelines for a bystander’s response to OHCA have emphasized the imperative of mouth-to-mouth ventilation, ironically called “rescue breathing.”4-8,33,80 Although mouth-to-mouth ventilation may rescue an individual with respiratory arrest, this approach actually decreases the likelihood of a “rescue” in a much larger group of patients—those with a primary arrhythmogenic cardiac arrest.81
There are several problems with mouth-to-mouth breathing as it is currently advocated. The most compelling argument against it is the widespread bystander reluctance to deliver direct mouth-to-mouth breathing. Bystander CPR has repeatedly been shown to be associated with increased survival and is a central part of the AHA “chain of survival.”82 A 2007 Japanese study, which included health care providers, showed only a 10% to 30% willingness to perform compressions plus rescue breathing as opposed to a 70% to 100% willingness to perform chest compression only.83 An anonymous bystander survey from Tucson, Arizona, revealed that only 15% of respondents would “definitely” do both rescue breathing and chest compressions; 68% would be willing to do just chest compressions.84 In a report from Los Angeles, bystander-assisted CPR was only seen in 28% of the 2021 patients in the study.14 Even certified basic life support (BLS) instructors and physicians have indicated reluctance to perform mouth-to-mouth resuscitation on strangers.85,86
Even with the new 30:2 compression ratios, there are excessive delays in initiating chest compressions, prolonged interruptions in chest compression, and excessive pre-shock pauses. Observations of hospital-based responders to cardiac arrest, as well as those made on paramedics, found that both professionals almost always performed assisted ventilation at excessively high rates.87 They both delivered an average of 37 ventilations per minute rather than the 10 to 12 per minute recommended by the guidelines. Even after retraining, they ventilated at 22 per minute. This translates into lesser instances in which any CPR is performed despite strong data suggesting that CCC is far better than no CPR at all, even for primary respiratory arrests.88 A swine study from the University of Arizona with 64 animals comparing CCC to the 2005 30:2 compression ratios showed significantly improved survival in the CCC group.89 Another 2007 study in humans showed that CCC was effective as 30:2 CPR.90
From a practical perspective, the bystander who will not give rescue breaths at 2:15 ratio are unlikely to give rescue breaths at 2:30 ratios. Also, rescue breathing, even when performed correctly by a single rescuer, takes time away from chest compression, which is the most significant generator of coronary and cerebral perfusion pressures.
Positive-pressure ventilation diminishes venous return in what is already a precarious cardiac output generated by chest compression. Decreased venous return to the chest further compromises the already marginal coronary and cerebral blood flow during cardiac arrest and resuscitation.91-93
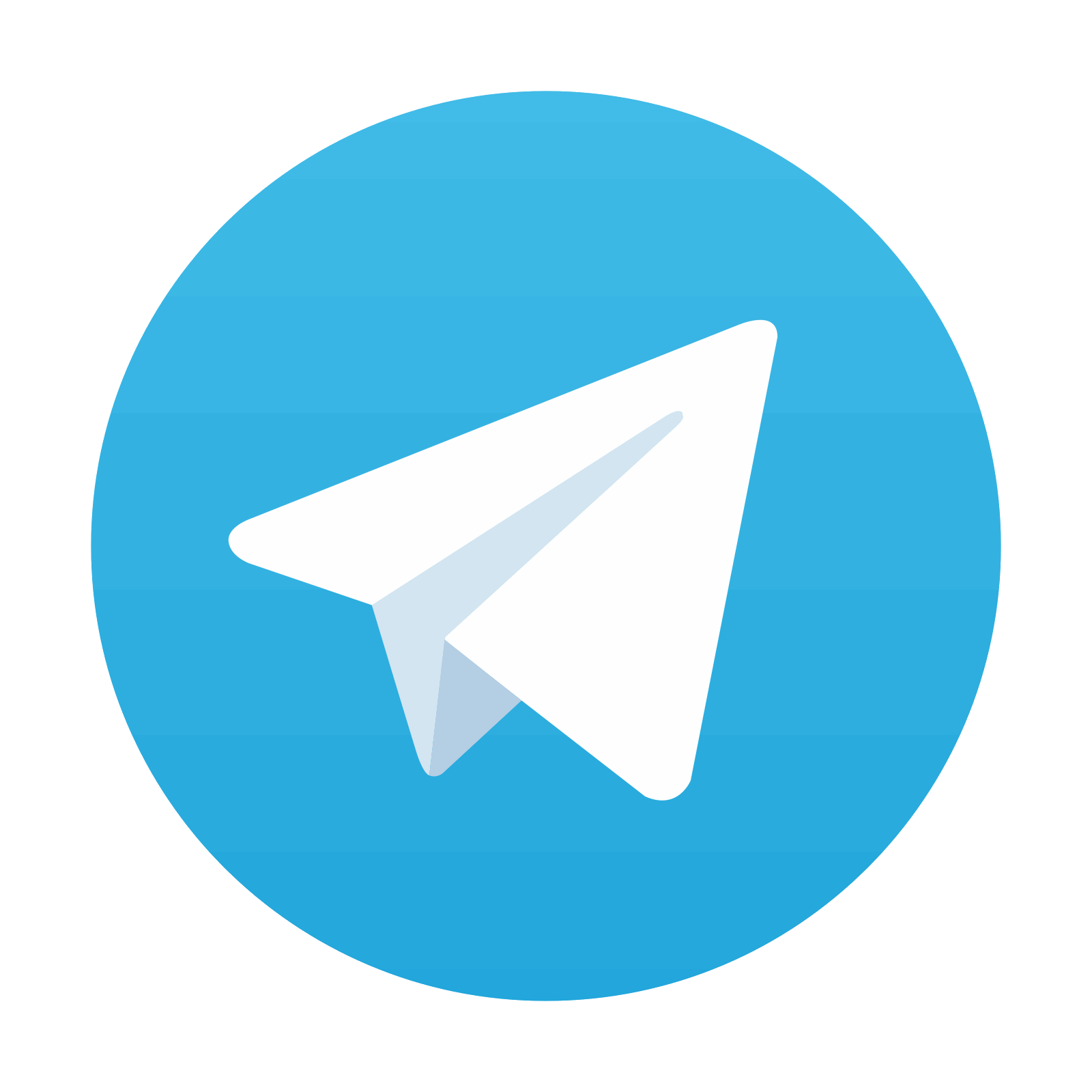
Stay updated, free articles. Join our Telegram channel

Full access? Get Clinical Tree
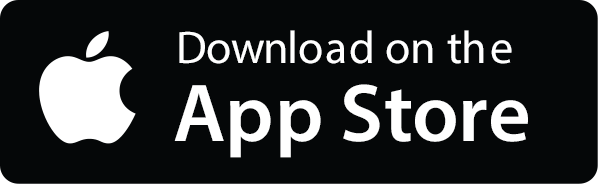
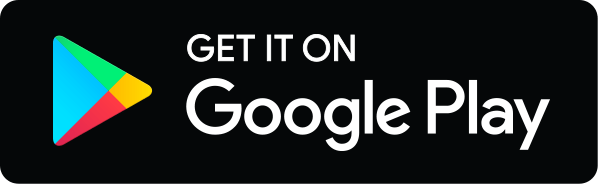
