Cardiomyopathies
Cardiomyopathy is defined as “disease of the myocardium associated with cardiac (systolic and/or diastolic) dysfunction” (1). The World Health Organization/International Society and Federation of Cardiology Task Force recommended that cardiomyopathies be classified by the dominant pathophysiologic mechanism or etiologic/pathogenic factor (Table 15-1), thus the following classification: 1) dilated cardiomyopathy, 2) hypertrophic cardiomyopathy, 3) restrictive cardiomyopathy, and 4) arrhythmogenic right ventricular (RV) dysplasia or cardiomyopathy. Noncompaction of the ventricular myocardium or left ventricular (LV) hypertrabeculation is another form of cardiomyopathy; it has been described recently (2,3,4) and has not yet been classified as a distinct entity by the World Health Organization. Each of these classes of cardiomyopathy has distinctive morphologic and functional characteristics with salient echocardiographic features:
Dilated cardiomyopathy is characterized by dilatation and reduced contractility of the LV or both the LV and RV.
Hypertrophic cardiomyopathy is characterized by hypertrophy of the LV; the hypertrophy is usually asymmetric and involves the ventricular septum. The LV cavity size is usually normal or decreased; a subset of the patients has obstruction of the LV outflow tract (LVOT) (5).
Restrictive cardiomyopathy is characterized by restrictive diastolic filling and decreased diastolic volume of either or both ventricles, with normal or near-normal systolic function. The atria are dilated because the noncompliant ventricles with abnormal myocardium cause restrictive diastolic filling (6).
Arrhythmogenic RV cardiomyopathy (ARVC), or arrhythmogenic RV dysplasia (ARVD), is caused by progressive fibrofatty replacement of the RV myocardium; occasionally, the LV is involved, with relative sparing of the septum (7).
Although cardiomyopathy can be classified in most patients on the basis of the clinical features and echocardiographic findings, the morphologic and functional features overlap, especially in patients with cardiomyopathy of advanced stage. Diastolic function becomes “restrictive” (grade 3 to 4 diastolic dysfunction) during the decompensation or end stage of all cardiomyopathies, regardless of the underlying lesion or classification. Many forms of cardiomyopathies are genetically inherited. The unusual as well as the typical echocardiographic features of different classes of cardiomyopathy are described in this chapter.
The Task Force also recommended that specific cardiomyopathies be used to describe heart muscle diseases that are associated with specific cardiac or systemic disorders (Table 15-1). Echocardiographic findings in systemic illnesses, including infiltrative cardiomyopathy, are described in Chapter 16.
Dilated Cardiomyopathy
Two-Dimensional Echocardiography
Dilated cardiomyopathy is characterized by a dilated LV cavity and decreased global systolic function (Fig. 15-1). Both end-diastolic and end-systolic dimensions and volumes are increased, and the variables of systolic function (ejection fraction, fractional shortening, systolic longitudinal motion of the mitral anulus, stroke volume, and cardiac output) are uniformly decreased. With gradual dilatation more along the short axis of the LV, the LV cavity becomes more spherical, with a sphericity index (long-axis dimension/short-axis dimension) nearing the value of 1 (normally ≥1.5). Wall thickness varies but typically is within normal limits; however, the LV mass is uniformly increased. Contractility usually is reduced
globally, but superimposed regional wall motion abnormalities can also be present. Similar findings occur in patients with extensive myocardial ischemia, myocarditis, alcoholic cardiomyopathy, hemochromatosis, peripartum cardiomyopathy, sarcoidosis, acute catecholamine crisis, acquired immunodeficiency syndrome, severe sepsis, or doxorubicin (Adriamycin) toxicity.
globally, but superimposed regional wall motion abnormalities can also be present. Similar findings occur in patients with extensive myocardial ischemia, myocarditis, alcoholic cardiomyopathy, hemochromatosis, peripartum cardiomyopathy, sarcoidosis, acute catecholamine crisis, acquired immunodeficiency syndrome, severe sepsis, or doxorubicin (Adriamycin) toxicity.
Table 15-1 Classification of cardiomyopathies by pathophysiologic mechanism or etiologic/pathogenic factor and by associated specific disorder | ||||||
---|---|---|---|---|---|---|
|
Secondary features in dilated cardiomyopathy include dilated mitral anulus and incomplete coaptation of the mitral valve leaflets responsible for the associated functional mitral regurgitation, evidence of low cardiac output (decreased excursion of the mitral leaflets), enlarged atrial cavities, RV enlargement, and apical mural thrombus. Interventricular conduction delay (left or right bundle branch block) is common and contributes further to cardiac dysfunction by causing intraventricular mechanical dyssynchrony (9,10).
It is recommended that immediate family members of a patient with dilated cardiomyopathy undergo echocardiographic screening because of the high incidence (20%–50%) of familial dilated cardiomyopathy (11). Clinical attributes of this familial disease have not been identified. In some cases of dilated cardiomyopathy, LV size and function may normalize with proper treatment (reverse remodeling).
Doppler (Pulsed Wave, Continuous Wave, Tissue Doppler) and Color Flow Imaging
Doppler echocardiography is useful for determining cardiac output, pulmonary artery pressure, mitral inflow filling pattern, LV filling pressure, and mechanical dyssynchrony. Cardiac output is routinely measured with use of the LVOT time velocity integral (TVI) and area (see Chapter 4). Mitral inflow filling patterns, tissue Doppler imaging, and color M-mode provide insight into LV filling pressures as well as into diastolic function (Fig. 15-2). Both mitral anulus systolic velocity (S′) and early diastolic velocity (E′) are decreased in dilated cardiomyopathy (12).
Although patients with dilated cardiomyopathy have a similar global systolic dysfunction, their clinical symptoms and hemodynamic status (diastolic filling pattern, pulmonary artery systolic pressure, and severity of mitral regurgitation) can differ markedly. One group of these patients may be minimally symptomatic, but another group may have symptoms of chronic heart failure. Doppler and color flow imaging provide important hemodynamic information that is helpful in assessing LV filling pressures, management strategy, and prognosis.
Patients with hemodynamic compensation have near-normal stroke volume and cardiac output and an impaired relaxation pattern (grade 1 diastolic dysfunction) as the diastolic filling abnormality (Fig. 15-3 A). Patients who have more advanced and decompensated dilated cardiomyopathy have decreased stroke volume (LVOT TVI is decreased) and a restrictive diastolic filling pattern (grade 3–4 diastolic dysfunction) (Fig. 15-3 B) because of decreased compliance and increased LV filling pressures. The evolution of diastolic dysfunction from an impaired relaxation pattern to a restrictive filling pattern was demonstrated elegantly in an animal model with tachycardia-induced dilated cardiomyopathy (13). Various diastolic filling variables (deceleration time of E and E/E′), which have a good correlation with pulmonary capillary wedge pressure (PCWP), have an incremental prognostic power over what the ejection fraction can provide. The shorter the deceleration time (DT) (i.e., more restrictive diastolic filling) and the higher E/E′ (i.e., higher PCWP), the worse the prognosis (14,15). As a patient’s heart failure is treated, diastolic filling becomes less restrictive and DT increases. The persistence of restrictive filling after therapy is associated with a high mortality and transplantation rate. Patients with reversible restrictive filling have a high probability of improvement and excellent survival (16). Mitral regurgitation is common in dilated cardiomyopathy and is related to the LV remodeling process. As the mitral leaflets become more tented because of apical displacement of the papillary muscles and regional remodeling, mitral regurgitation becomes worse. A mitral valve tenting area of 6 cm2 or more is usually associated with severe mitral regurgitation (Fig. 15-4). Treatment directed toward reverse remodeling improves mitral regurgitation as well as LV systolic function.
The status of pulmonary artery pressure estimated from tricuspid regurgitation velocity is also prognostic in dilated cardiomyopathy (17). Patients with a tricuspid regurgitation velocity greater than 3 m/s have a higher mortality rate, a higher incidence of heart failure, and more frequent hospitalizations than those with a lower tricuspid regurgitation velocity. High tricuspid regurgitation velocities
are seen usually in patients who have a restrictive diastolic filling pattern. Therefore, a restrictive diastolic filling pattern and high tricuspid regurgitation velocity identify patients who are at increased risk for death and heart failure.
are seen usually in patients who have a restrictive diastolic filling pattern. Therefore, a restrictive diastolic filling pattern and high tricuspid regurgitation velocity identify patients who are at increased risk for death and heart failure.
|
Natriuretic peptide levels have been found to be elevated in patients with dilated or ischemic cardiomyopathy and increased filling pressure. The N-terminal pro–B type natriuretic peptide (NT-proBNP) level was analyzed in the Carvedilol Prospective Randomized Cumulative Survival (COPERNICUS) trial, and baseline NT-proBNP levels above median (>1,767 pg/mL) were associated with an increased risk for all-cause mortality (7.0% vs. 21.6%) (18). Because of the good correlation between Doppler diastolic filling variables and NT-proBNP, the combination of information may have a more powerful prognostic implication than information from either source alone.
Echocardiography in the Management of Dilated Cardiomyopathy
Proper timing of diastolic filling is important in optimizing cardiac output (Fig. 15-5). If the PR interval becomes prolonged (>200 milliseconds), atrial contraction may occur before early diastolic filling is completed. If the PR interval is too short (60–100 milliseconds), the atrium may contract at the same time as the ventricle. Therefore, by optimizing the PR interval with the guidance of Doppler echocardiography, mitral inflow may increase cardiac output and the patient’s symptoms may improve (19). Atrioventricular optimization is also important after a biventricular pacing
device has been placed in patients who have mechanical dyssynchrony. The optimal atrioventricular delay can be achieved by identifying an interval that produces nonfused mitral E and A velocities without truncating the duration of A velocity. This can be done empirically by reviewing mitral inflow velocities on various atrioventricular delays or by the method described by Ritter and colleagues (20) (see Chapter 5 and Fig. 5-11):
device has been placed in patients who have mechanical dyssynchrony. The optimal atrioventricular delay can be achieved by identifying an interval that produces nonfused mitral E and A velocities without truncating the duration of A velocity. This can be done empirically by reviewing mitral inflow velocities on various atrioventricular delays or by the method described by Ritter and colleagues (20) (see Chapter 5 and Fig. 5-11):
SAV optimal = SAVshort + d
where d = (SAVlong + QAlong) – (SAVshort + QAshort) and SAV is sensed atrioventricular (AV) delay.
Left bundle branch block is common in patients with dilated cardiomyopathy. Intraventricular dyssynchrony created by a left bundle branch block results in ineffective cardiac contraction and heart failure (21,22). Tissue Doppler imaging and other modified techniques (tissue tracking, tissue synchronization imaging, or strain imaging) are able to quantify the degree of intraventricular dyssynchrony. The initial data suggest that patients with a greater dyssynchrony receive more benefit from cardiac resynchronization therapy than those with less dyssynchrony (23,24). Numerous variables can be quantified with echocardiography as a measure of mechanical dyssynchrony (Fig. 15-6). Initial clinical experience suggests that intraventricular mechanical dyssynchrony (defined as more than a 60-millisecond difference in time to peak tissue Doppler systolic velocity) between lateral and septal basal segments (25), more than a 100-millisecond maximal difference in time to peak systolic velocities from 12 segments, or more than 33 milliseconds of standard deviation derived from 12 basal and mid-level segments of LV best predicts who will have a good response to biventricular pacing (26). However, it is often difficult to identify the timing of peak systolic velocity, and there may be multiple systolic velocities when recorded by tissue Doppler echocardiography. It appears from our prospective data that dyssynchrony measured by strain imaging does provide better predictive value for identifying responders than dyssynchrony by tissue velocity (27). Which echocardiographic variables are most helpful in predicting a positive response
to cardiac resynchronization requires additional clinical investigation.
to cardiac resynchronization requires additional clinical investigation.
The use of a passive constraint device has been reported to minimize LV remodeling or even to result in reverse remodeling (28). Echocardiography is essential for identifying patients with dilated cardiomyopathy who are appropriate for these innovative therapies and for monitoring their responses. A randomized clinical trial (ACORN) was performed to assess a cardiac support device in patients with dilated cardiomyopathy. As the echocardiography core laboratory, the Mayo Clinic Echocardiography Laboratory measured all echocardiographic data. These data showed that the decrease in LV volume and improvement in the sphericity index of the patients with the ACORN device were significantly more than those of patients without the device.
Mitral regurgitation frequently accompanies dilated cardiomyopathy, and its severity contributes to a worse clinical outcome. Reverse remodeling by medical or device therapy (or both) improves not only LV function but also mitral regurgitation. Some new devices are aimed at correcting mitral regurgitation without addressing progressive LV dilatation. Whether this approach directed toward mitral regurgitation alone improves the hemodynamics and survival of patients needs to be assessed in large clinical trials. According to a retrospective review of 419 patients with dilated or ischemic cardiomyopathy, mitral valve annuloplasty for moderate to severe mitral regurgitation had no clearly demonstrable mortality benefit (29). A prospective randomized controlled trial is warranted for this population. By reliably measuring structural, functional, and hemodynamic variables, echocardiography is uniquely suited to monitoring the progression of dilated cardiomyopathy.
Reverse remodeling has been attempted by surgical anterior ventricular endocardial restoration (SAVER), introduced in 1984 (30). It results in a characteristic
echocardiographic appearance (Fig. 15-7). This procedure is most suitable for patients who have a large LV (LV end-systolic volume ≥60 mL/m2), scarred akinetic apex, and normally contracting inferior lateral walls. Whether SAVER improves a patient’s outcome or achieves reverse remodeling, including prevention of worsening mitral regurgitation, is being tested in the STICH trial.
echocardiographic appearance (Fig. 15-7). This procedure is most suitable for patients who have a large LV (LV end-systolic volume ≥60 mL/m2), scarred akinetic apex, and normally contracting inferior lateral walls. Whether SAVER improves a patient’s outcome or achieves reverse remodeling, including prevention of worsening mitral regurgitation, is being tested in the STICH trial.
![]() Figure 15-5 A: Mitral flow velocity curve and simultaneous left atrial (LA) and left ventricular (LV) pressure curves in a 76-year-old man with a long PR interval and severe LV dysfunction (ejection fraction, 25%) due to severe coronary artery disease. He has severe New York Heart Association functional class IV symptoms. Left, Atrial pacing with anterograde native conduction and a long atrioventricular delay. There is an increase in LV pressure above LA pressure during atrial relaxation in mid-diastole (arrowhead), culminating in a shortening of diastolic filling time and onset of diastolic regurgitation. The baseline cardiac output (CO) is 3.0 L/min. Center, Atrioventricular (AV) pacing at a short AV interval of 60 milliseconds. Diastolic filling occurs through all of diastole. Atrial contraction now occurs simultaneously with LV contraction, resulting in a lower CO than on the left. Note that mean LA pressure increased from 31 mm Hg (left) to 42 mm Hg (center). Right, AV pacing at the optimal AV interval of 180 milliseconds. The relation of atrial contraction to the onset of ventricular contraction is now optimal (see text), resulting in diastolic filling throughout the entire diastolic filling period. An appropriate relation exists between mechanical LA and LV contraction so that mean LA pressure is maintained at a low level (34 mm Hg), with LA contraction occurring just before LV contraction. This causes an increase in LV end-diastolic pressure to 43 mm Hg. CO has increased to 5.2 L/min. B: Continuous wave Doppler image of mitral regurgitation signal and simultaneous LA and LV pressure curves in a patient with a long PR interval. Left, Normal sinus rhythm (NSR) with a long intrinsic PR interval. Diastolic mitral regurgitation (arrowheads) is due to an increase in LV pressure above LA pressure before ventricular contraction. Center, P-synchronous pacing with a short AV interval of 60 milliseconds. Diastolic mitral regurgitation is no longer present, but there is a decrease in CO from that on the left from an atrial contraction that is ineffective because it occurs during ventricular contraction. Right, P-synchronous pacing at the optimal AV interval (100 milliseconds). Diastolic mitral regurgitation is no longer present. LV diastolic pressure increases appropriately at the onset of ventricular contraction. LV pressure has increased from 30 mm Hg (left) to 43 mm Hg (right). (From Nishimura et al [19]. Used with permission.) |
Hypertrophic Cardiomyopathy
Two-Dimensional and M-Mode Echocardiography
Hypertrophic cardiomyopathy is a genetic disorder caused by a missense mutation in 1 of at least 10 genes that encode the proteins of the cardiac sarcomere (5). Mutations
associated with hypertrophic cardiomyopathy are scattered throughout sarcomeric genes, with mutations in the gene encoding the β-myosin heavy chain representing one of the most common genetic causes of hypertrophic cardiomyopathy (31). The phenotypic expression of hypertrophic cardiomyopathy, which occurs in 1 of every 500 adults, includes massive hypertrophy involving primarily the ventricular septum.
associated with hypertrophic cardiomyopathy are scattered throughout sarcomeric genes, with mutations in the gene encoding the β-myosin heavy chain representing one of the most common genetic causes of hypertrophic cardiomyopathy (31). The phenotypic expression of hypertrophic cardiomyopathy, which occurs in 1 of every 500 adults, includes massive hypertrophy involving primarily the ventricular septum.
Although asymmetric septal hypertrophy is the most common type of morphologic pattern, hypertrophic cardiomyopathy can present with concentric, apical, or free wall LV hypertrophy (5,32,33,34) (Fig. 15-8). When the basal septum is hypertrophied and bulging, the LVOT becomes narrowed, providing a substrate for dynamic obstruction. The velocity of blood flow across the narrowed LVOT increases and produces the Venturi effect. Consequently, the mitral leaflets and support apparatus are drawn toward the septum (i.e., systolic anterior motion), obstructing the LVOT (Fig. 15-9). This obstruction is dynamic and depends on the loading conditions and LV size and contractility. When aortic flow is interrupted by obstruction of the LVOT, the aortic valve develops premature midsystolic closure. Systolic anterior motion of the mitral valve distorts the configuration of the valve, resulting in mitral regurgitation. Therefore, varying degrees of mitral regurgitation almost invariably accompany the obstructive form of hypertrophic cardiomyopathy.
M-mode echocardiography is useful in documenting asymmetric septal hypertrophy, systolic anterior motion of the mitral valve (see Fig. 2-19 B), and midsystolic aortic valve closure. Asymmetric septal hypertrophy is also seen in RV hypertrophy, hypertension, and inferior wall myocardial infarction with preceding LV hypertrophy. Systolic anterior motion of the mitral valve can be seen also in other hyperdynamic cardiac conditions. Two-dimensional (2D) echocardiography is the method of choice for establishing the diagnosis of hypertrophic cardiomyopathy. Furthermore, detailed morphologic characterization is provided by 2D echocardiographic imaging. The most frequent morphologic variety of hypertrophic cardiomyopathy consists of diffuse hypertrophy of the ventricular septum and anterolateral free wall (70%–75% of cases), followed by basal septal hypertrophy (10%–15% of cases), concentric hypertrophy (5% of cases), apical hypertrophy (<5% of cases), and hypertrophy of the lateral wall (1%–2% of cases). The magnitude of hypertrophy is related directly to the risk of sudden death. The cumulative risk for sudden death is near 0% for a wall thickness of 19 mm or less and 40% for a thickness of 30 mm or more (35).
Apical hypertrophic cardiomyopathy may be missed on 2D echocardiography unless an apical examination is performed carefully. A parasternal examination may not provide the diagnosis because the hypertrophy usually is confined to the apex (Fig. 15-10 A and C
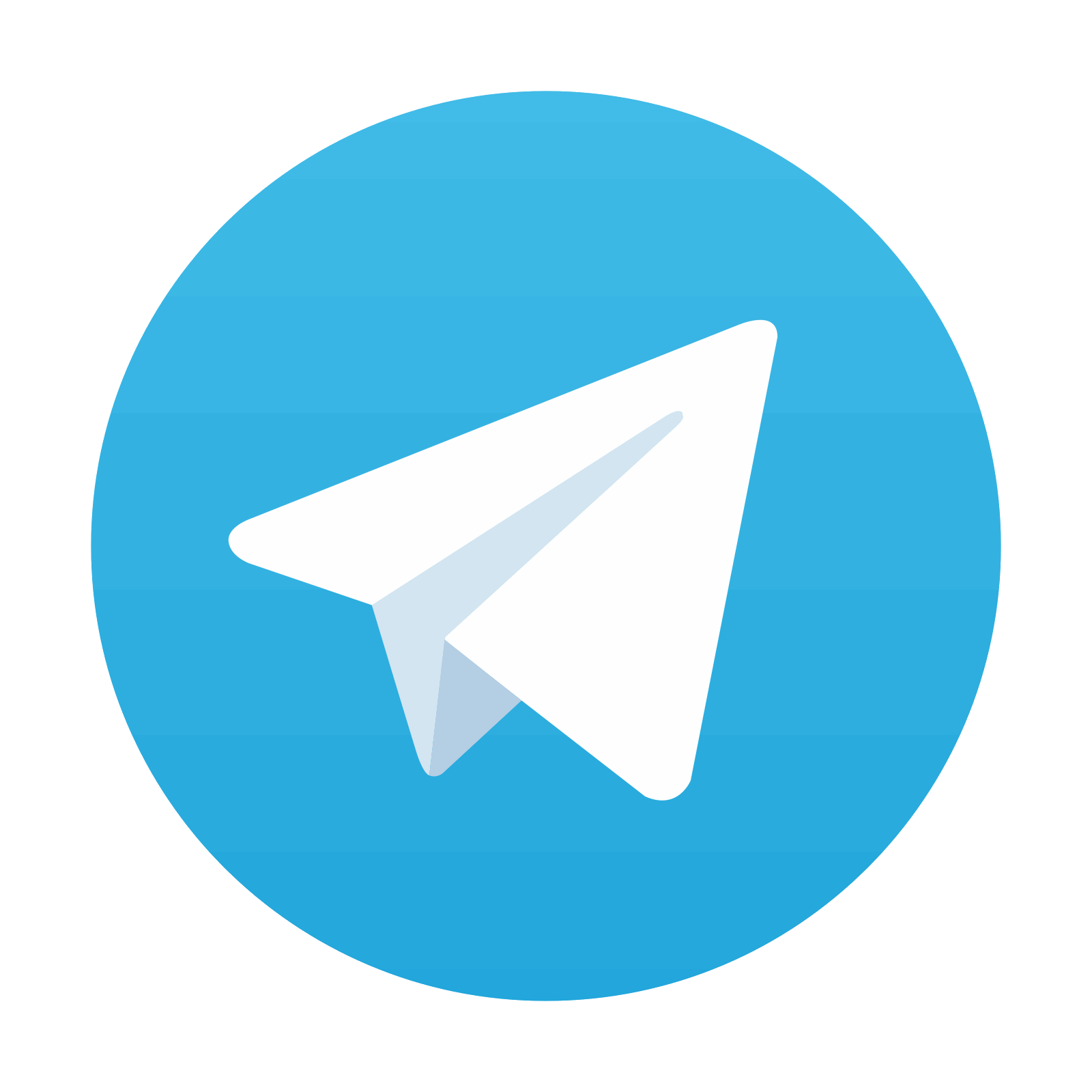
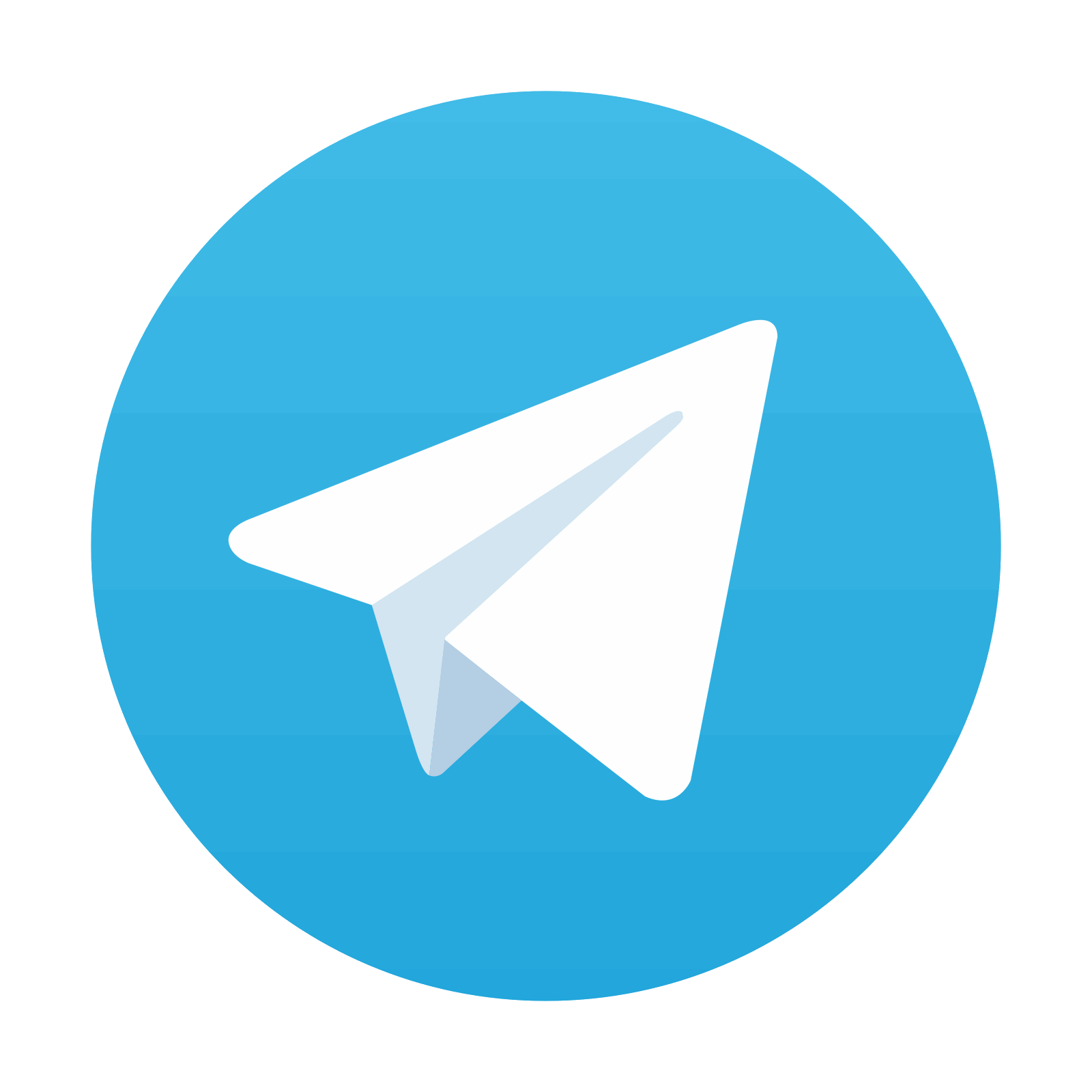
Stay updated, free articles. Join our Telegram channel

Full access? Get Clinical Tree
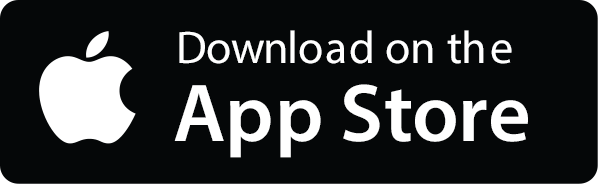
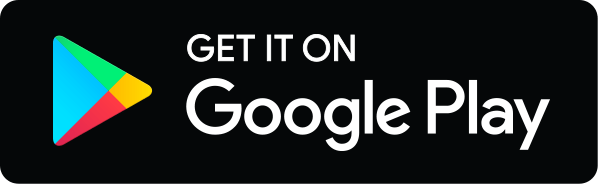
