Prolonged endurance-type exercise is associated with elevated cardiac troponin (cTn) levels in asymptomatic recreational athletes. It is unclear whether exercise-induced cTn release mirrors a physiological or pathological underlying process. The aim of this study was to provide a direct comparison of the release kinetics of high-sensitivity cTnI (hs-cTnI) and T (hs-cTnT) after endurance-type exercise. In addition, the effect of remote ischemic preconditioning (RIPC), a cardioprotective strategy that limits ischemia-reperfusion injury, was investigated in a randomized controlled crossover manner. Twenty-five healthy volunteers completed an outdoor 30-km running trial preceded by RIPC (4 × 5 min 220 mm Hg unilateral occlusion) or control intervention. hs-cTnT, hs-cTnI, and sensitive cTnI (s-cTnI) concentrations were examined before, immediately after, 2 and 5 hours after the trial. The completion of a 30-km run resulted in a significant increase in circulating cTn (time: all p <0.001), with maximum hs-cTnT, hs-cTnI, and s-cTnI levels of 47 ± 27, 69 ± 62, and 82 ± 64 ng/L (mean ± SD), respectively. Maximum hs-cTnT concentrations were measured in 60% of the participants at 2 hours after exercise, compared with maximum hs-cTnI and s-cTnI concentrations at 5 hours in 84% and 80% of the participants. Application of an RIPC stimulus did not reduce exercise-induced cTn release (time × trial: all p >0.5). In conclusion, in contrast to acute myocardial infarction, maximum hs-cTnT levels after exercise precede maximum hs-cTnI levels. Distinct release kinetics of hs-cTnT and hs-cTnI and the absence of an effect of RIPC favors the concept that exercise-induced cTn release may be mechanistically distinct from cTn release in acute myocardial infarction.
Insight into the (patho)physiology of exercise-induced cardiac troponin (cTn) release is an active and relevant topic of discussion. The recent advent of both a high-sensitivity (hs-) assay for cTnI (cTnI) and T (cTnT) with similar analytical characteristics enables to directly compare the kinetics of both diagnostic equivalent molecules. For example in acute myocardial infarction (AMI), where cTn release results from the breakdown of the contractile apparatus after ischemic myocardial injury, peak levels of hs-cTnI and hs-cTnT are reached at similar levels after admission. A direct comparison of exercise-induced hs-cTnI and hs-cTnT release could provide more insight into the underlying (patho)physiology. In addition, remote ischemic preconditioning (RIPC), a powerful noninvasive cardioprotective strategy, can be applied to study the contribution of an imbalance between oxygen supply and demand in exercise-induced cTn release. RIPC describes the application of brief episodes of nonlethal ischemia and reperfusion to a tissue or an organ, resulting in protection of the same or another visceral organ against an injurious ischemic insult in the future. In line with the cardioprotective effect of RIPC in various settings of tissue ischemia, we hypothesize that if an oxygen demand-supply imbalance contributes to exercise-induced cTn release, application of an RIPC stimulus would result in reduced postexercise cTn levels. The aim of this study was a head-to-head comparison of the release kinetics of hs-cTnT and hs-cTnI after prolonged endurance-type exercise. In addition, we examined the effect of RIPC on exercise-induced cTn release using a randomized controlled single-blind crossover design.
Methods
Twenty-nine healthy runners (age range 18 to 65 years) were recruited with posters at local running clubs. Before testing, all participants were informed about the study procedures and method but remained naïve to the study rationale. This study was carried out according to the principles of the Declaration of Helsinki and approved by the local Institutional Review Board and Ethics Committee of Maastricht University Medical Center. All subjects provided written informed consent before participation. This study was conducted from March 2013 to June 2013 and was registered at clinicaltrials.gov as NCT01774461 .
In a randomized controlled single-blind crossover design, participants completed 2 identical outdoor 30-km running trials, either preceded by an RIPC or control intervention. Both experimental test days started in the morning and were separated by at least 2 weeks. Before each trial, participants were instructed to refrain from any strenuous physical labor and sports activities for 24 hours and to standardize their breakfast on both experimental days. The running trials were organized in a research setting on paved (bicycle) lanes with a minimal number of cross sections with the start and finish ≈ 1 km from Maastricht University Medical Center. While running, participants were individually followed by a researcher on a bicycle and allowed to drink water and Isostar sports drink ad libitum. Before the first running trial, a comprehensive 2-dimensional and 3-dimensional transthoratic echocardiogram at rest was recorded from all participants. No abnormalities were observed.
Temporal unilateral upper arm ischemia was achieved by inflating a blood pressure cuff to 220 mm Hg. Circulatory occlusion lasted 5 minutes and was followed by 5 minutes of reperfusion by deflation of the cuff. This sequence was repeated for a total of 4 cycles and resulted in a total procedure time of 40 minutes (4 × [5 minutes of circulatory occlusion + 5 minutes of nonocclusion]). The control intervention followed an identical protocol, except for the blood pressure cuff being inflated to only 20 mm Hg, allowing uninterrupted perfusion. The order of testing (RIPC vs control) was randomized and counterbalanced between participants.
Blood samples were collected in serum and ethylenediaminetetraacetic acid-containing tubes at baseline (preceding the RIPC intervention), immediately after exercise, 2 and 5 hours after exercise. Within 1 hour of collection, hematology parameters were analyzed on a Sysmex XE-5000 (Kobe, Japan) analyzer. In addition, serum tubes were centrifuged, and aliquots were stored at −80°C until analysis. Serum cTnT was measured using the high-sensitivity assay of Roche Diagnostics (Basel, Switzerland); the clinical reference limit (99th percentile of a healthy reference population) is 14 ng/L, with a 10% analytical variation at 13 ng/L. Serum cTnI was measured with the following 2 assays: the STAT high-sensitive troponin I assay (Abbott Diagnostics, Abbott Park, IL) and the Access AccuTnI+3 assay (Beckman Coulter, Brea, CA) According to the package insert, the 99th percentile limit of the Abbott hs-cTnI assay is 26.2 ng/L with a corresponding coefficient of variation of 4%. The Beckman Coulter assay has a 99th percentile limit of 40 ng/L and a corresponding 10% imprecision, as specified by the manufacturer. Complementary cardiovascular or skeletal biomarkers; N-terminal pro–B-type natriuretic peptide (NTproBNP), creatine kinase (CK), CK muscle and brain fraction (CK-MB), and lactate dehydrogenase were measured using assays of Roche Diagnostics. In addition, creatinine (Roche Diagnostics) and cystatin C (Gentian, Moss, Norway) were measured to calculate the estimated glomerular filtration rate according to the Chronic Kidney Disease Epidemiology Collaboration (CKD-EPI) formula.
Sample size estimation was based on hs-cTnT as the primary outcome variable. We aimed to include 29 runners to detect a 30% reduction in exercise-induced hs-cTnT release due to RIPC (from 40 ± 32 ng/L to 28 ± 32 ng/L, mean ± SD) with a statistical power of 0.8 at an alpha error probability of 0.05. An estimated postexercise hs-cTnT concentration of 40 ng/L was based on our previous studies of marathon-induced hs-cTnT release. The expected effect size of 30% was derived from the reports, where a 40% reduction of cTn release by RIPC preceding cardiac and vascular surgery has been observed. Assuming a similar effect in endurance-type exercise, we considered that a <30% reduction would exclude a relevant contribution of ischemia-reperfusion in the setting of exercise-induced cTn release.
Data are presented as mean ± SD. A 2-factor (time × trial) repeated-measures general model was used to assess difference in biochemical parameters. In addition, trial order (control or RIPC intervention) was entered into the statistical model as a between-subject variable, partitioning out this source of variability (analyses not shown). In the case of a nonnormal distribution, data were transformed by natural logarithm. All statistical tests were 2-tailed, and a p value of <0.05 was considered statistically significant. Analyses were performed using IBM SPSS Statistics for Windows 22.0 (IBM Corp, Armonk, NY).
Results
Twenty-nine participants (23 men and 6 women) were included in the study. Three male participants left the study prematurely due to a sports-related injury (n = 2) or medical reason unrelated to the study protocol (n = 1) and were therefore not included in the analysis. One male participant was excluded after study completion because of highly elevated hs-cTnT (Roche), hs-cTnI (Abbott), and sensitive cTnI (s-cTnI, Beckman) concentrations (72, 316, and 332 ng/L, respectively) before his second running trial. In the absence of clinical symptoms, these values were highly suggestive for exercise-induced troponin release in the past 24 hours and hence indicative for noncompliance to a critical aspect of the study protocol (refrainment of exercise in the 24 hours preceding the run). Therefore, 25 participants (19 men and 6 women) with an age of 40 ± 13 years (mean ± SD) were included in the present analysis ( Table 1 ). Subjects were moderately to highly trained, with an average of 52 running kilometers per week (range: 20 to 120). Fourteen runners (56%) completed at least one marathon or ultradistance trial. None of the subjects had a history of cardiovascular disease, assessed by medical questionnaire.
Variable | Subjects’ characteristics |
---|---|
Men | 19 (76%) |
Age (years) | 40 ± 13 |
Body mass index (kg/m 2 ) | 22 (18-25) |
Physical activity (h/week) | 7 (2-17) |
Running activity (km/week) | 52 (20-120) |
Running experience (years) | 10 (2-30) |
Marathon or ultra-distance experience | 14 (56%) |
The exercise trials were separated by a median of 2 weeks (range: 2 to 7). All participants completed both trials within a time span of 1:57:13 to 3:13:36 (hours:minutes:seconds), without symptoms of myocardial ischemia. There was no difference in the completion time between the control trial and the trial preceded by ischemic preconditioning: mean control trial 2:26:56 and mean RIPC trial 2:26:54. The overall average timeframe between the intervention and the 30-km run was 24 minutes (range: 15 to 41) and similar in the setting with and without RIPC. The overall average delay from finish to the first postexercise blood sample collection was 23 minutes (range: 14 to 33) with no differences in the setting with and without RIPC.
At baseline of the control trial, almost all athletes displayed hs-cTnT, hs-cTnI, and s-cTnI concentrations below the clinical decision limit of myocardial infarction (24, 23, and 24 participants, respectively; Figure 1 ). The completion of a 30-km run resulted in an expected significant increase of circulating hs-cTnT, hs-cTnI, and s-cTnI (time: all p <0.001; Figure 1 , Table 2 , Supplementary Figure 1 ). Maximum concentrations of hs-cTnT, hs-cTnI, and s-cTnI were 47 ± 27 ng/L, 69 ± 62 ng/L, and 82 ± 64 ng/L (mean ± SD, control trial) and exceeded the clinical reference value of hs-cTnT, hs-cTnI, and s-cTnI in 100% (n = 24), 60% (n = 15), and 68% (n = 17) of the participants. The kinetics of hs-cTnT was different from hs-cTnI and s-cTnI, as 60% of the participants (n = 15) displayed hs-cTnT maximum concentrations at 2 hours after exercise, whereas maximum concentrations of hs-cTnI and s-cTnI were measured at 5 hours after exercise in 84% and 80% participants (n = 21 and n = 20), respectively. Elevations of hs-cTnT, hs-cTnI, and s-cTnI measured immediately after exercise in the control trial were significantly correlated with each other (Pearson: all R >0.8, p <0.001). In addition, NTproBNP concentrations increased significantly after the 30-km run with maximum concentrations of 14 ± 8 pmol/L (mean ± SD, control trial, time: p <0.001; Figure 1 , Table 2 , Supplementary Figure 2 ). Elevations of NTproBNP were not significantly associated with hs-cTnT, hs-cTnI, or s-cTnI (Pearson: all R <0.2, p >0.3).
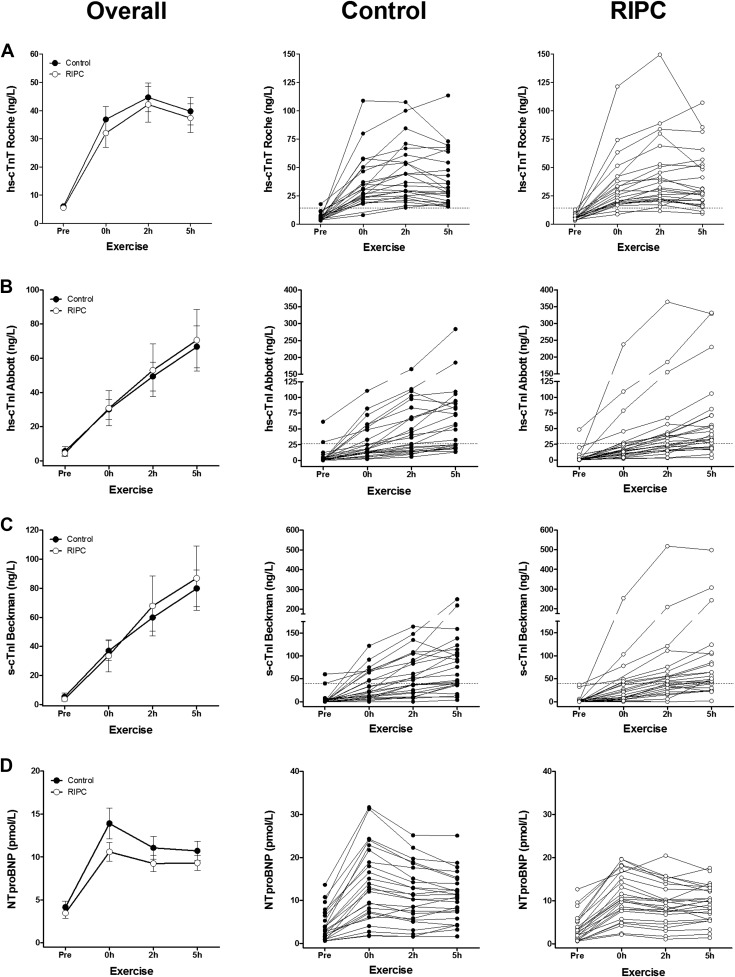
Parameter | Pre-exercise | 0h post-exercise | 2h post-exercise | 5h post-exercise | P-value |
---|---|---|---|---|---|
hs-cTnT Roche (ng/L) | Time: p <0.001 | ||||
Control | 6 ± 4 | 37 ± 22 | 45 ± 26 | 40 ± 24 | Trial: p=0.2 |
RIPC | 6 ± 3 | 32 ± 25 | 42 ± 31 | 37 ± 26 | Time x Trial: p=0.5 |
hs-cTnI Abbott (ng/L) | Time: p<0.001 | ||||
Control | 6 ± 13 | 30 ± 28 | 49 ± 42 | 67 ± 61 | Trial: p=0.4 |
RIPC | 4 ± 10 | 31 ± 51 | 53 ± 78 | 71 ± 90 | Time x Trial: p=0.5 |
s-cTnI Beckman (ng/L) | Time: p<0.001 | ||||
Control | 5 ± 14 | 37 ± 33 | 60 ± 47 | 80 ± 63 | Trial: p=0.2 |
RIPC | 4 ± 10 | 33 ± 53 | 68 ± 104 | 87 ± 110 | Time x Trial: p=0.7 |
NTproBNP (pmol/L) | Time: p<0.001 | ||||
Control | 4 ± 4 | 14 ± 9 | 11 ± 7 | 11 ± 6 | Trial: p=0.1 |
RIPC | 3 ± 3 | 11 ± 5 | 9 ± 5 | 9 ± 4 | Time x Trial: p=0.1 |
CK (U/L) | Time: p<0.001 | ||||
Control | 144 ± 88 | 267 ± 125 | 314 ± 127 | 423 ± 185 | Trial: p=0.9 |
RIPC | 129 ± 81 | 264 ± 132 | 323 ± 167 | 505 ± 300 | Time x Trial: p=0.1 |
CK-MB (μg/L) | Time: p<0.001 | ||||
Control | 4 ± 3 | 6 ± 3 | 7 ± 3 | 10 ± 5 | Trial: p=0.9 |
RIPC | 4 ± 2 | 6 ± 3 | 8 ± 4 | 12 ± 7 | Time x Trial: p=0.2 |
LD (U/L) | Time: p<0.001 | ||||
Control | 173 ± 26 | 274 ± 60 | 259 ± 51 | 252 ± 45 | Trial: p=0.5 |
RIPC | 167 ± 21 | 276 ± 63 | 262 ± 58 | 249 ± 48 | Time x Trial: p =0.2 |
eGFR (mL/min/1.73 m 2 ) | Time: p<0.001 | ||||
Control | 110 ± 12 | 79 ± 16 | 94 ± 15 | 103 ± 13 | Trial: p =0.4 |
RIPC | 108 ± 12 | 79 ± 20 | 93 ± 20 | 100 ± 18 | Time x Trial: p=0.6 |
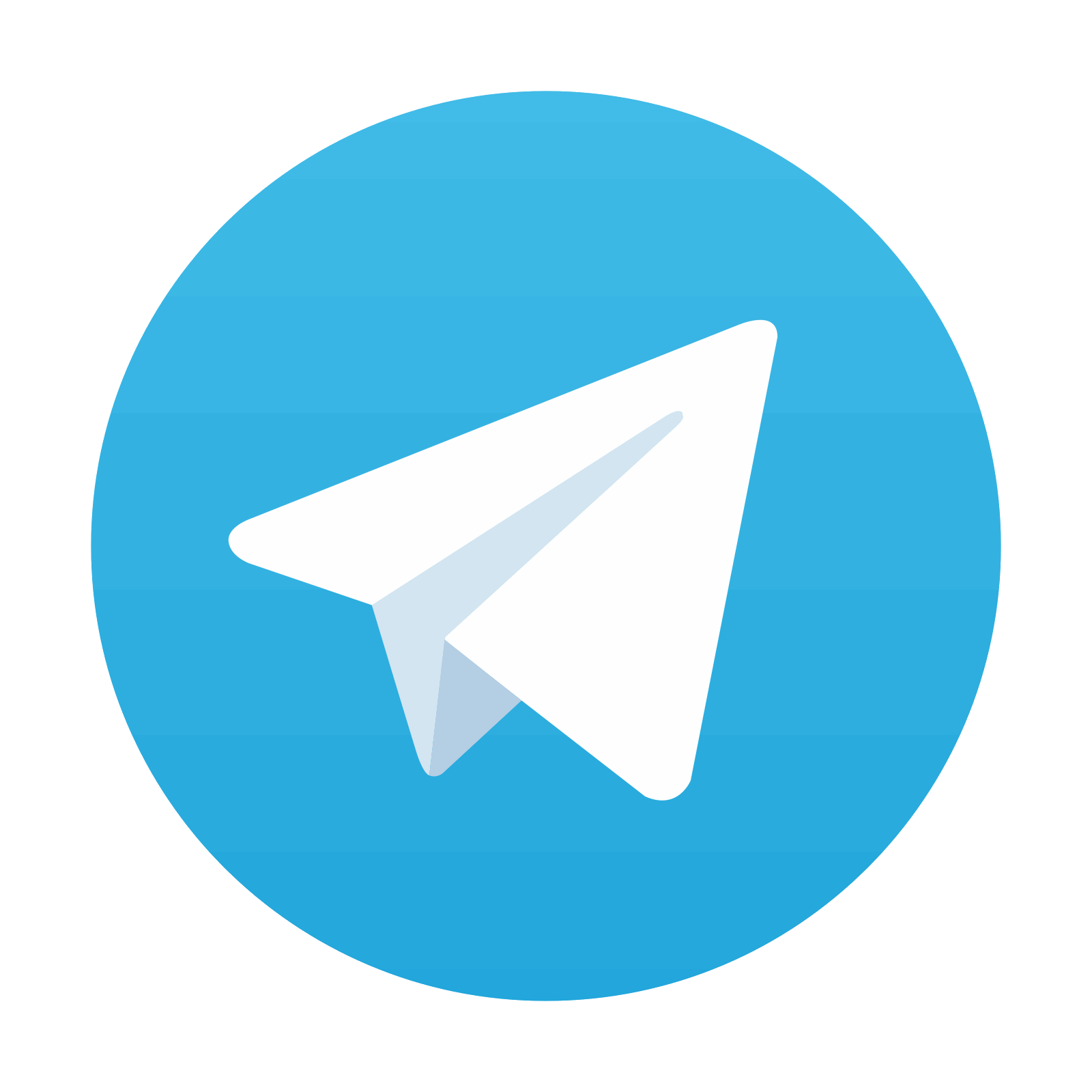
Stay updated, free articles. Join our Telegram channel

Full access? Get Clinical Tree
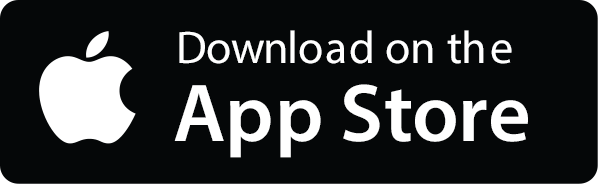
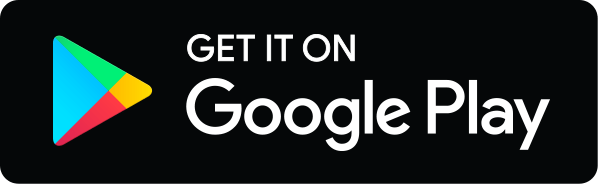
