Cardiac Rehabilitation and Secondary Prevention
Philip A. Ades
Rainer Hambrecht
Background
Cardiac rehabilitation is a multifactorial process that includes exercise training, education and behavioral counseling regarding risk reduction and lifestyle changes. These services should be integrated into the comprehensive care of cardiac patients. (1)
Cardiac rehabilitation–lifestyle therapy for coronary heart disease (CHD) is fundamentally different from other well-established pharmacologic, interventional, or surgical therapies: It does not rely on a single pathogenic mechanism for intervention, it is not a “single-bullet” treatment, and requires the ongoing cooperation and participation of the patient. These characteristics are not signs of an ill-defined polypragmatic approach without a sound scientific basis, but rather are deeply rooted in the chronic multifactorial disease process that drives the progression of atherosclerosis. The risk factor concept of atherogenesis derived from population-based studies such as The Framingham Study (2) has greatly advanced our understanding of the determining environmental and behavioral factors. Many of the mechanisms that mediate the adverse cardiovascular effects of physical inactivity are still unknown. Nonetheless, the epidemiologic link between lack of physical activity and/or diminished fitness and increased risk for CHD is clear and well proven (3).
Cardiac rehabilitation is a therapeutic approach based on epidemiologic and pathophysiologic mechanisms, which is aimed at modifying the atherogenic disease process on the individual level. Although some approach the area of cardiac rehabilitation with skepticism and consider it inferior to “high-tech” interventions, the ultimate goal of medical care continues to be to prolong and improve quality of life. Using prognostic impact as a measure of therapeutic efficacy, cardiac rehabilitation is by no means inferior to conventional pharmacologic or interventional care. For example, it was recently demonstrated that cardiac rehabilitation with a 12-month outpatient exercise training program is superior to percutaneous coronary interventions regarding the prevention of subsequent cardiovascular events and improvement of exercise tolerance (4). The mortality reduction in stable CHD achieved by exercise-based rehabilitation is 27%, which is comparable to the effects of our most potent drugs such as β-blocking agents, lipid-lowering drugs, and angiotensin converting enzyme inhibitors (5). Furthermore, an invasive interventional approach in the nonacute setting is severely handicapped by the fact that you only treat the most advanced coronary lesions but are unable to modify overall disease progression of what is, in essence, a diffuse metabolic disease. Exercise-based rehabilitation, on the other hand, embraces the concept that it is most often not the highest grade stenoses that initiate an acute coronary event but rather a ruptured plaque in a less severe obstruction that is the culprit. Comprehensive cardiac rehabilitation aims at influencing all determinants of the atherosclerotic disease process.
Comprehensive cardiac rehabilitation services include medical evaluation, prescribed exercise, cardiac risk factor modification, and lifestyle counseling. The clinical implementation of cardiac rehabilitation is frequently linked to a structured “secondary prevention center,” with on-site and home-based exercise programs, lipid clinics, weight loss programs, and other risk factor modification components aimed at preventing second coronary events, cardiac rehospitalizations, and cardiac disability in patients with established CHD (6,7). Services are tied to a series of short-term and longer term outcomes, which include return to work and measures of physical functioning, cardiac symptoms, psychological well-being, risk factors, progression of coronary atherosclerosis, recurrence of cardiac events, and number of rehospitalizations. Cardiac rehabilitation is prescribed for patients after myocardial infarction (MI), coronary bypass surgery, or after percutaneous coronary interventions, in addition to patients with chronic angina pectoris, chronic heart failure, or heart transplantation and finally for selected patients after valvular heart surgery or exertional arrhythmias (1,8). Debate is ongoing as to whether early outpatient exercise training requires direct supervision
or whether it could effectively be more widely applied, for low- and moderate-risk patients, in the home setting (9,10,11,12). The clinical application of training therapy in coronary artery disease (CAD) and chronic heart failure patients is detailed in Table 13.1.
or whether it could effectively be more widely applied, for low- and moderate-risk patients, in the home setting (9,10,11,12). The clinical application of training therapy in coronary artery disease (CAD) and chronic heart failure patients is detailed in Table 13.1.
Cardiac rehabilitation is highly cost effective and is associated with a decrease in cardiac hospitalizations (13,14,15,16). The cardiac rehabilitation facility should be viewed as the clinical site at which systematic secondary prevention services are delivered in an outpatient setting in collaboration with the primary physician. Maintaining physical functioning, preventing coronary disability, and preventing recurrent coronary events are the primary outcome goals.
The Evolution of Modern Cardiac Rehabilitation and Secondary Prevention
Historical Background: Evolution From Symptomatic to Prognostic Intervention
Cardiac rehabilitation was conceived in an era when post-MI activity prescriptions were evolving from 6 weeks of bed rest in the 1930s, to “chair” therapy in the 1940s, to 3 to 5 minutes of walking per day at 4 weeks in the 1950s (17,18,19). By the early 1960s, clinicians recognized that early ambulation helped patients to avoid many of the complications of bed rest, such as pulmonary embolism and deconditioning, and in-hospital ambulation gradually displaced long-term bed rest as the standard of care (20,21,22). As the ambulation process extended beyond hospital discharge, concerns about the safety of unsupervised exercise resulted in the development of highly structured, physician-supervised, electrocardiographically monitored exercise programs in the 1970s. The focus was almost exclusively on exercise and on the physical recovery process. By the 1990s, hospitalizations for acute MI shortened and are now as brief as 3 to 4 days (23), such that deconditioning is minimal. However, also minimized is the ability to counsel patients about risk factor modification. Smoking relapse prevention programs, initiated in hospital, have proven effective (24), but therapy and education for other modifiable risk factors are largely left for the postdischarge period.
With the development of a body of literature supporting the benefits of risk factor modification in coronary patients (called secondary prevention), the cardiac rehabilitation center has evolved to become a clinical site for the systematic delivery of secondary prevention services. Recently demonstrated benefits of cardiac rehabilitation and secondary prevention in coronary patients are broad and compelling. A recent meta-analysis of patients participating in exercise-based rehabilitation programs following an acute MI documented a significant 27% reduction of total mortality among training patients and a 31% reduction in cardiac mortality, confirming earlier work (5,25,26). A recent report from Olmstead county in Minnesota provides encouraging and interesting observations in a “real world” setting (27). These investigators identified 1821 patients with MI discharged from the hospital alive between 1982 and 1998 and 55% of patients participated in cardiac rehabilitation, defined conservatively as attendance at a single visit. Three-year survival was 95% in participants and 64% in nonparticipants. This survival benefit was maintained after adjusting for propensity to participate in cardiac rehabilitation with an overall 56% reduction in mortality, which was similar across age and gender subgroups. Participation in cardiac rehabilitation was further associated with a 28% reduction
in recurrent MI. Interestingly, the benefits of cardiac rehabilitation were more pronounced in recent years, coincident with greater participation of elderly, women, and patients with more medical comorbidities.
in recurrent MI. Interestingly, the benefits of cardiac rehabilitation were more pronounced in recent years, coincident with greater participation of elderly, women, and patients with more medical comorbidities.
TABLE 13.1 Indications, Exercise Prescription, and Expected Outcomes for Exercise-Based Cardiac Rehabilitation in Selected Patient Populations | |
---|---|
|
![]() FIGURE 13.1. Kaplan-Meier survival curve of patients with stable coronary artery disease randomized to either interventional treatment (PCI) or aerobic endurance exercise training (Training) over 12 months. Training was associated with a significantly lower rate of major cardiovascular events (death, MI, stroke, hospitalization for worsening angina, need for revascularization) as compared to conventional PCI. Reprinted with permission (4). |
The therapeutic benefit of regular physical exercise has also been confirmed in direct comparison with an interventional strategy: 12 months of exercise therapy in stable CAD patients was associated with a higher event-free survival and an increased exercise capacity than a percutaneous coronary intervention (Fig. 13.1) (4). Furthermore, the combination of exercise and low-fat diets has been documented to slow the atherogenic process (28,29), and studies that incorporated multiple risk factor interventions (9), pharmacologic lipid lowering, or both, demonstrated a decrease in coronary events and a retardation of atherosclerosis (30).
![]() FIGURE 13.2. Survival rates of patients with stable chronic heart failure randomized to optimal medical therapy with or without additional exercise training. In this meta-analysis a total of 801 patients from nine independent prospective randomized clinical trials were included. Over a follow-up period of 705 days training was associated with a 35% reduction of all-cause mortality. Reprinted with permission (31). |
Over the past two decades, rehabilitation programs have been extended into new diagnostic categories beyond standard post-MI treatment. In stable chronic heart failure, physical activity was traditionally discouraged, with negative physiologic consequences for the patients. Exercise intolerance worsened and the progression of disease-related muscular atrophy accelerated. However, carefully designed exercise programs with an intensity of 50% to 70% of maximal oxygen uptake or 70% to 85% of maximal heart rate, have proven to be effective in increasing exercise capacity by approximately 20%. As confirmed by a recent meta-analysis, exercise therapy reduces the relative risk of chronic heart failure mortality by 35% and chronic heart failure–related hospitalizations by 28% (Fig. 13.2) (31).
The general focus of cardiac rehabilitation today is no longer just to limit the devastating physical and mental consequences of a serious cardiovascular event, but to prevent future cardiac events by combining individually tailored exercise programs with chronic disease management skills as a long-term intervention. In the context of this process, exercise training is offered to most patients with chronic stable cardiovascular diseases including CHD, chronic heart failure, valvular heart disease, congenital heart disease, peripheral vascular occlusive disease, and after cardiac transplantation. Despite well-described benefits, fewer than 15% of patients who might benefit currently participate in formal cardiac rehabilitation, due in part to a lack of geographically available programs (32,33).
Scientific Foundation of Cardiac Rehabilitation and Secondary Prevention
As the primary goal of cardiac rehabilitation interventions has changed from regaining muscle strength to modification of atherosclerotic progression and improving prognosis, studies of exercise or comprehensive interventions have focused on mechanistic as well as symptomatic outcomes. This development shifted the scientific basis of rehabilitation interventions in many patient groups, most notably in CHD and chronic
heart failure patients, from empirical knowledge to pathophysiologic evidence. The molecular concepts of how exercise interventions interfere with the underlying disease process are now providing a new basis for developing more effective training programs.
heart failure patients, from empirical knowledge to pathophysiologic evidence. The molecular concepts of how exercise interventions interfere with the underlying disease process are now providing a new basis for developing more effective training programs.
Pathophysiology of Exercise Intolerance in Coronary Artery Disease
The Exercise Limitation in Coronary Artery Disease
Traditionally, coronary stenoses were regarded as static flow obstructions leading to exercise-induced ischemia whenever myocardial oxygen demand exceeded the regional blood supply. Although this mechanism is still valid to a degree, the pathogenesis of myocardial ischemia is frequently more complex. From the first description of a paradoxical vasoconstriction of atherosclerotic segments during acetylcholine infusion into coronary arteries (34), we know that changes in coronary endothelial function can critically diminish coronary blood flow even in patients with moderate epicardial coronary artery stenoses (50% to 70%). It has been shown that a paradoxical response to acetylcholine is indicative of a greater vasoconstrictive effect to both endogenous and exogenous catecholamines (35). Sympathetic activation and consecutive release of catecholamines occurs during physical activity, exposure to cold, or mental stress. In all these contexts, paradoxical epicardial coronary vasoconstriction has been described (36,37,38), which makes endothelial dysfunction a likely pathomechanism to explain stress- or exercise-induced angina pectoris in stable CAD (39).
Cardiovascular Effects of Exercise in Coronary Artery Disease
The first era of mechanistic rehabilitation research started in the late 1980s and assessed the effects of aggressive lifestyle interventions including exercise on the progression of coronary atherosclerosis. Studies evaluating the regression hypothesis used quantitative coronary angiography to quantify changes in target lesion diameter.
Three major studies—the Lifestyle Heart Trial (29), the Stanford Coronary Risk Intervention Project (SCRIP) (9), and the Heidelberg Regression Study (28)—confirmed that comprehensive lifestyle changes including a low-fat diet and/or lipid-lowering medication and exercise training were effective in slowing or slightly reversing the progression of coronary atherosclerosis and in reducing the cardiac adverse event rate. However, only a minority of trained patients actually had a significant regression in minimal stenosis diameter, those who exercised more than 5 to 6 hours of per week (>2500 kcal/week) (40). In contrast, myocardial perfusion was improved and coronary event rate was reduced out of proportion to the minimal angiographic regression noted (41).
With the observation of the importance of endothelial function came an awareness that the luminal diameter of epicardial vessels is highly dynamic in response to mechanical (flow-related) or agonist-mediated (endogenous or pharmacologic) stimuli. Hence, a new era of research assessed the effects of endurance training on coronary endothelial function. Hambrecht and colleagues (42) documented a significant improvement in coronary endothelial function in patients with stable CAD after only 4 weeks of high-intensity exercise training (60 minutes at 70% of VO2 max/d) (Fig. 13.3). In two consecutive studies of moderate exercise training in patients awaiting coronary bypass grafting surgery, where intraoperative harvesting of left internal mammary artery samples was possible, two important mechanisms of training-inducedchanges were described: The balance between nitric oxide production and nitric oxide degradation was favorably changed by training as a result of increased vascular extracellular nitric oxide synthase expression and activity and reduced generation of reactive oxygen species
produced by vascular NAD(P)H oxidases (43,44). In addition, animal experiments indicate improved vascular antioxidative protection by elevated extracellular superoxide dismutase activity after training (45). The significance of these data is underscored by long-term follow-up studies, which confirmed the predictive value of endothelial dysfunction for future cardiovascular events (46,47).
produced by vascular NAD(P)H oxidases (43,44). In addition, animal experiments indicate improved vascular antioxidative protection by elevated extracellular superoxide dismutase activity after training (45). The significance of these data is underscored by long-term follow-up studies, which confirmed the predictive value of endothelial dysfunction for future cardiovascular events (46,47).
![]() FIGURE 13.3. In patients with stable CAD a four-week high-intensity training program was effective in significantly attenuating paradoxical coronary vasoconstriction in response to intracoronary infusion of acetylcholine at a rate of 7.2 μg/min (A). In addition, average coronary peak flow velocity (APV) during acetylcholine infusion almost doubled (B) and coronary flow reserve increased by 29% (C) (42). **P < 0.01 versus control group. #P < 0.05 vs. control group for comparison of percent change (begin vs. 4 weeks) between groups. |
Recently, yet another potential mechanism of training-induced improvement of myocardial perfusion has been identified: Contrary to previous concepts, postnatal vasculogenesis has been confirmed in humans, which is initiated by pluripotent bone marrow–derived circulating endothelial progenitor cells (EPCs) (48). Training increases the number of circulating EPCs and may thereby improve endothelial regeneration and collateral formation into ischemic tissue areas (49,50).
Pathophysiology of Exercise Intolerance in Chronic Heart Failure
Exercise Intolerance in Chronic Heart Failure
It was originally hypothesized that exercise intolerance in chronic heart failure occurred as a direct consequence of insufficient adaptation of cardiac output to the increased demands during physical exertion and that any extra workload placed on the diseased myocardium would inevitably lead to further deterioration of left ventricular function. However, exercise studies in chronic heart failure patients documented a complete lack of correlation between the left ventricular ejection fraction and exercise capacity (51).
If central hemodynamics are not the principal determining factor of exercise capacity, what other factors should be considered? The first focus of interest was on systemic neurohumeral alterations—a concept of chronic heart failure evolving as a clinical process that was initiated by myocardial dysfunction but which then affected virtually any organ system as a consequence of neurohormonal activation (52,53). This pathophysiologic model of chronic heart failure is still valid today and has been extended by demonstration of significant immune activation and inflammation in chronic heart failure (54,55).
Building on this model, peripheral changes associated with neurohumoral and inflammatory activation have been systematically analyzed in the last decade. Characteristic changes of endothelial function, respiration, and skeletal muscle function have been found. Today it is well-established that chronic heart failure causes a peripheral hypoperfusion owing to impaired endothelium-dependent vasodilation (56) leading to cytokine activation (57), a reduction in the strength of respiratory muscles (58), and profound morphologic (59,60), metabolic (61,62), and functional alterations in skeletal muscle (63). In the course of these scientific advances, peripheral changes have become a new therapeutic target (Fig. 13.4).
Effects of Exercise
With regard to symptomatic benefit after exercise training in chronic heart failure, a recent meta-analysis of randomized controlled trials by the European Heart Failure Training Group revealed an improvement of peak Vo2 by up to 2 mL/kg·min-1 with a range of +14% to +31% increase versus control patients (64). Although modest in absolute terms this increase of about 20% translates into a considerably better quality of life for most patients. Cardiac function is not worsened by exercise training; rather, there was a small but significant improvement of ejection fraction and a reduction in cardiomegaly (65). Exercise training is a nonspecific intervention that affects several functional systems in chronic heart failure patients, namely, vascular endothelial function, central hemodynamics, neurohumoral activation, the respiratory system, and skeletal muscle metabolism and function.
Endothelium-dependent vasodilation, especially during exercise, is significantly improved by endurance training in chronic heart failure patients (66,67), which contributes to a reduced cardiac afterload and enhanced skeletal muscle perfusion. Cardiac function is enhanced, most likely as a consequence of afterload reduction after a 6-month training program (Fig. 13.5) (65). Apart from systolic function, clinical studies indicate an improved left ventricular early diastolic filling following exercise interventions in chronic heart failure subgroups with relaxation abnormalities (68).
Neurohumoral activation as indicated by circulating levels of angiotensin II, aldosterone, and atrial natriuretic peptide was reduced by 25% to 32% after long-term exercise training (69). The activity and strength of inspiratory muscles is decreased in chronic heart failure patients contributing to a reduction of the key clinical symptom of exercise-induced dyspnea. Both systemic exercise training and selective respiratory muscle training improve ventilation dynamics and exercise performance (58).
Skeletal muscle morphology, metabolism, and function are significantly altered in chronic heart failure. These changes are not just a consequence of deconditioning but represent intrinsic changes induced by systemic neurohumoral and inflammatory responses in chronic heart failure. All aspects of skeletal muscle characteristics can be positively influenced by training: On the ultrastructural level, the volume density of cytochrome C–positive mitochondria is increased permitting an enhanced oxidative phosphorylation. In addition to metabolic improvements, recent studies indicate that training has the potential to reverse the inflammatory activation with increased expression of cytokines like tumor necrosis factor-α (TNF-α), interleukin (IL)-1β, and IL-6 in the skeletal muscle (57). It is hoped that these changes might also attenuate the proapoptotic environment with reduced IGF-I in the skeletal muscle (70,71).
Clinical Application of Exercise Training
In light of the multiple beneficial effects of exercise in chronic heart failure, training programs are recommended by current guidelines on chronic heart failure treatment. Both the American Heart Association and the European Society of Cardiology agree that exercise programs should be encouraged in stable NYHA class II and III patients (72,73,74). Clinical stability is defined by stable symptoms, absence of resting symptoms and postural hypotension, stable fluid balance (need of an increase in diuretic dosage no more than once a week), freedom from evidence of congestion, stable renal function (creatinine level), and normal or near-normal electrolyte values (75). With respect to the 15% to 25% functional improvement of exercise tolerance, the reduction of symptoms, and the improved quality of life, exercise training is therefore recommended in stable chronic heart failure (73).
Exercise training is usually performed as a steady-state or interval program of 50% to 80% of the maximal heart rate or at an exercise intensity (in Watts (W)) equivalent to 50% to 70% of the maximal exercise capacity reached during exercise testing. Exercise sessions should start at a low level (e.g., 50% of maximal heart rate for 10 minutes) and individual titration of exercise training should be performed in the following order: duration (up to 20 minutes per unit), then frequency (up to three times a day and/or three to five times per week), and then intensity (up to 70% to 80% of peak heart rate) (73).
In chronic heart failure patients, aerobic training should be combined with resistance exercise to antagonize the muscle
catabolism seen in more advanced stages of heart failure, although this has been a topic of some controversy. The reluctance to include resistance exercise in training programs for chronic heart failure patients is based on older studies that found a rise in afterload along with an acute reduction in cardiac output and an increase in the severity of mitral regurgitation with prolonged isometric exercise (74,75). More recent studies with a maximum of 2 × 10 repetition exercises at 70% of maximal capacity, however, show no significant decrease in ejection fraction or an increase in systolic BP (78,79,80).
catabolism seen in more advanced stages of heart failure, although this has been a topic of some controversy. The reluctance to include resistance exercise in training programs for chronic heart failure patients is based on older studies that found a rise in afterload along with an acute reduction in cardiac output and an increase in the severity of mitral regurgitation with prolonged isometric exercise (74,75). More recent studies with a maximum of 2 × 10 repetition exercises at 70% of maximal capacity, however, show no significant decrease in ejection fraction or an increase in systolic BP (78,79,80).
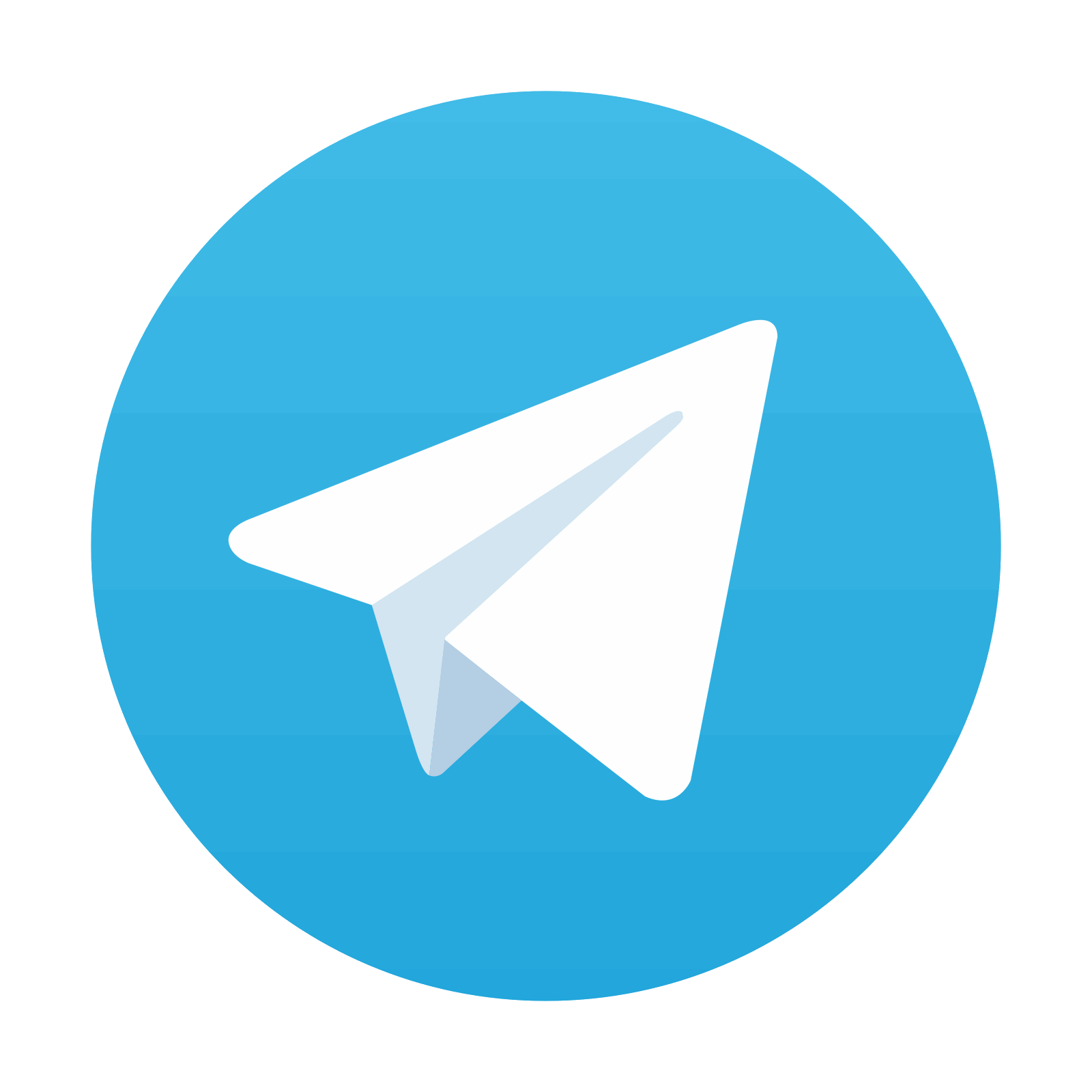
Stay updated, free articles. Join our Telegram channel

Full access? Get Clinical Tree
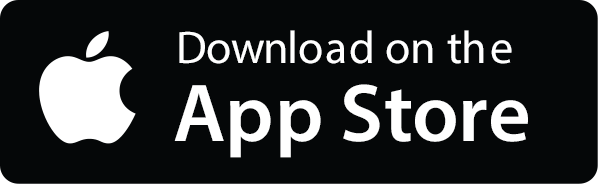
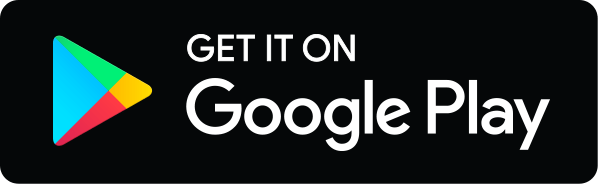
