However, the right atrium/right ventricle and left atrium/left ventricle are not directly aligned with the right and left sides of the body as viewed in the transverse plane magnetic resonance image of the heart within the thorax (Fig. 1.2A). The schematic drawing shows how the right-sided chambers of the heart are located anterior to the left-sided chambers, with the result that the interatrial and interventricular septa form a diagonal in this transverse plane view (see Fig. 1.2B).1,2
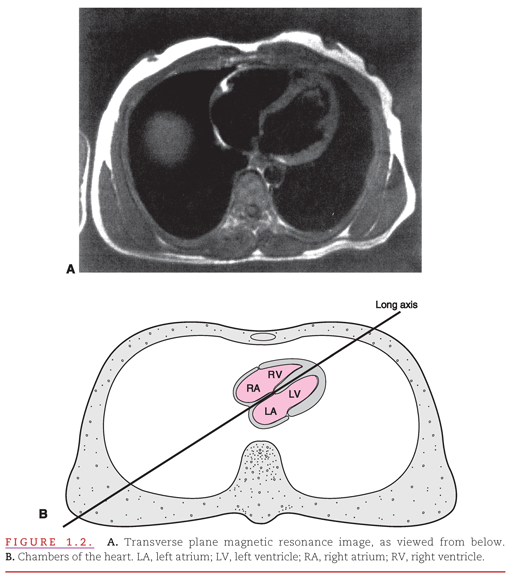
The mechanical pumping action of the heart is produced by cardiac muscle (“myocardial”) cells that contain contractile proteins. The timing and synchronization of contraction of these myocardial cells are controlled by noncontractile cells of the pacemaking and conduction system. Impulses generated within these specialized cells create a rhythmic repetition of events called cardiac cycles. Each cycle includes electrical and mechanical activation (systole) and recovery (diastole). The terms commonly applied to these components of the cardiac cycle are listed in Table 1.1. Because the electrical events initiate the mechanical events, there is a brief delay between the onsets of electrical and mechanical systole and of electrical and mechanical diastole.
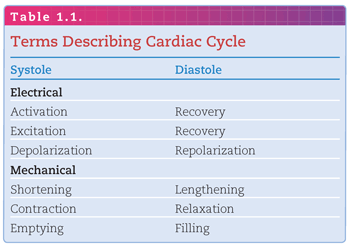
The electrical recording from inside a single myocardial cell as it progresses through a cardiac cycle is illustrated in Figure 1.3. During electrical diastole, the cell has a baseline negative electrical potential and is also in mechanical diastole, with separation of the contractile proteins. At top, a single cardiac cell is shown at three points in time, during which it is relaxed, contracted, and relaxed again. An electrical impulse arriving at the cell allows positively charged ions to cross the cell membrane, causing its depolarization. This movement of ions initiates “electrical systole,” which is characterized by an action potential. This electrical event then initiates mechanical systole, in which the contractile proteins within the myocardial cell slide over each other, thereby shortening the cell. Electrical systole continues until the positively charged ions are pumped out of the cell, causing its repolarization. Below the cell is a representation of an internal electrical recording that returns to its negative resting level. The repolarization process begins with an initial brief component that is followed by a “plateau” that varies among myocardial cells. Repolarization is completed by a rapid component. This return of “electrical diastole” causes the contractile proteins within the cell to separate. The cell is then capable of being reactivated when another electrical impulse arrives at its membrane.
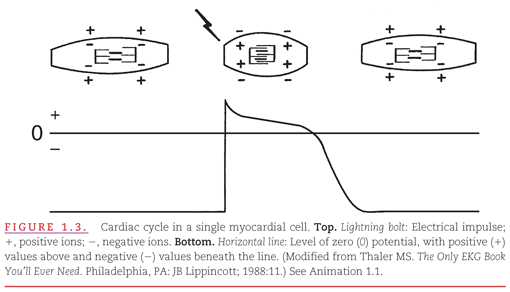

The electrical and mechanical changes in a series of myocardial cells (aligned end to end) as they progress through a cardiac cycle are illustrated in Figure 1.4. In Figure 1.4A, the four representative cells are in their resting or repolarized state. Electrically, the cells have negative charges; mechanically, their contractile proteins are separated. An electrical stimulus arrives at the second myocardial cell in Figure 1.4B, causing electrical and then mechanical systole. The wave of depolarization in Figure 1.4C spreads throughout all the myocardial cells. In Figure 1.4D, the recovery or repolarization process begins in the second cell, which was the first to depolarize. Finally, in Figure 1.4E, the wave of repolarization spreads throughout all of the myocardial cells, and they await the coming of another electrical stimulus.3–6
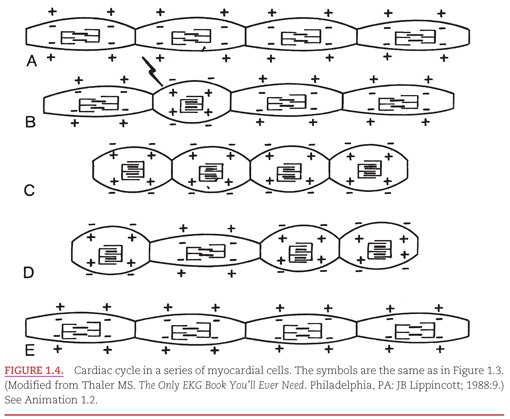

In Figure 1.5, the relationship between the intracellular electrical recording from a single myocardial cell presented in Figure 1.3 is combined with an ECG recording on a “lead” that has its positive and negative electrodes on the body surface. The ECG recording is the summation of electrical signals from all of the myocardial cells. There is a flat baseline in two very different situations: (a) when the cells are in their resting state electrically and (b) when the summation of cardiac electrical activity is directed perpendicular to a line between the positive and negative electrodes. The depolarization of the cells produces a high-frequency ECG waveform. Then, between the initial transient and final complete phases of repolarization, the ECG returns to the baseline. Completion of repolarization of the myocardial cells is represented on the ECG by a lower frequency waveform in the opposite direction from that representing depolarization.
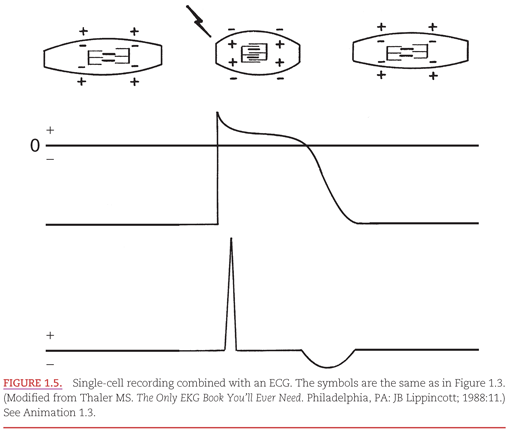

In Figure 1.6, a lead with its positive and negative electrodes has been placed on the body surface and connected to a single-channel ECG recorder. The process of production of the ECG recording by waves of depolarization and repolarization spreading from the negative toward the positive electrode is illustrated. In Figure 1.6A, the first of the four cells shown is electrically activated, and the activation then spreads into the second cell. This spread of depolarization toward the positive electrode produces a positive (upward) deflection on the ECG. In Figure 1.6B, all of the cells are in their depolarized state, and the ECG recording returns to its baseline level. In Figure 1.6C, repolarization begins in the same cell in which depolarization was initiated, and the wave of repolarization spreads into the adjoining cell. This produces the oppositely directed negative (downward) waveform on the ECG recording.
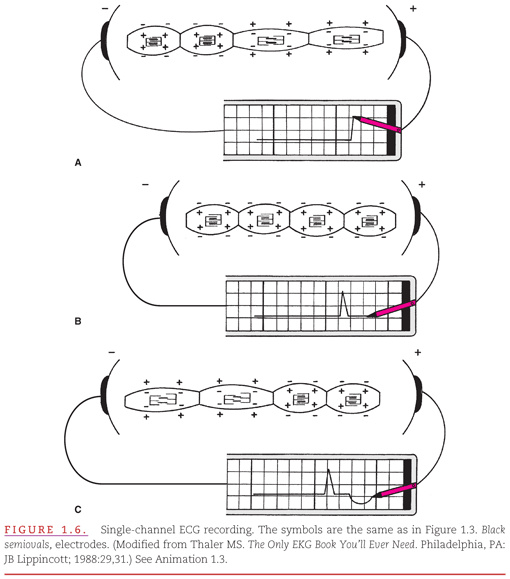

CARDIAC IMPULSE FORMATION AND CONDUCTION
The electrical activation of a single cardiac cell or even of a small group of cells does not produce enough voltage to be recorded on the body surface. Clinical electrocardiography is made possible by the activation of large groups of atrial and ventricular myocardial cells, whose numbers are of sufficient magnitude for their electrical activity to be recorded on the body surface.
Myocardial cells normally lack the ability for either spontaneous formation or rapid conduction of an electrical impulse. They depend on special cells of the cardiac pacemaking and conduction system that are located strategically through the heart for these functions (Fig. 1.7). These cells are arranged in nodes, bundles, bundle branches, and branching networks of fascicles. The cells that form these structures lack contractile capability, but they can generate spontaneous electrical impulses (act as pacemakers) and alter the speed of electrical conduction throughout the heart. The intrinsic pacemaking rate is most rapid in the specialized cells in the atria and slowest in those in the ventricles. This intrinsic pacemaking rate is altered by the balance between the sympathetic and parasympathetic components of the autonomic nervous system.7–10
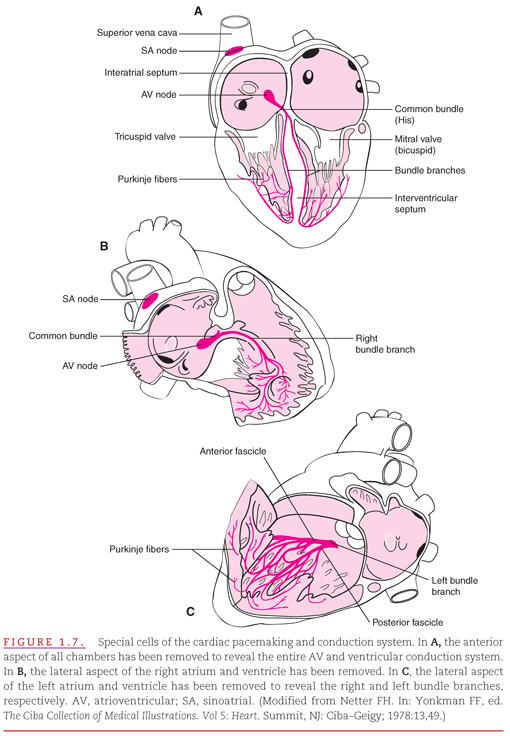
Figure 1.7 illustrates three different anatomic relationships between the cardiac pumping chambers and the specialized pacemaking and conduction system: Anterior precordium with less tilt (see Fig. 1.7A), right anterior precordium looking onto the interatrial and interventricular septa through the right atrium and ventricle (see Fig. 1.7B), and left posterior thorax looking onto the septa through the left atrium and ventricle (see Fig. 1.7C). The sinoatrial (SA) or sinus node is located high in the right atrium, near its junction with the superior vena cava. The SA node is the predominant cardiac pacemaker, and its highly developed capacity for autonomic regulation controls the heart’s pumping rate to meet the changing needs of the body. The atrioventricular (AV) node is located low in the right atrium, adjacent to the interatrial septum. Its primary function is to slow electrical conduction sufficiently to asynchronize the atrial contribution to ventricular pumping. Normally, the AV node is the only structure capable of conducting impulses from the atria to the ventricles because these chambers are otherwise completely separated by nonconducting fibrous and fatty tissue.11–13
In the atria, the electrical impulse generated by the SA node spreads through the myocardium without needing to be carried by any specialized conduction bundles. Electrical impulses reach the AV node where the impulse is delayed before continuing to the intraventricular conduction pathways.
The intraventricular conduction pathways include a common bundle (bundle of His) that leads from the AV node to the summit of the interventricular septum as well as the right and left bundle branches of the bundle of His, which proceed along the septal surfaces of their respective ventricles. The left bundle branch fans out into fascicles that proceed along the left septal endocardial surface and toward the two papillary muscles of the mitral valve. The right bundle branch remains compact until it reaches the right distal septal surface, where it branches into the interventricular septum and toward the free wall of the right ventricle. These intraventricular conduction pathways are composed of fibers of Purkinje cells, which have specialized capabilities for both pacemaking and rapid conduction of electrical impulses. Fascicles composed of Purkinje fibers form networks that extend just beneath the surface of the right and left ventricular endocardium
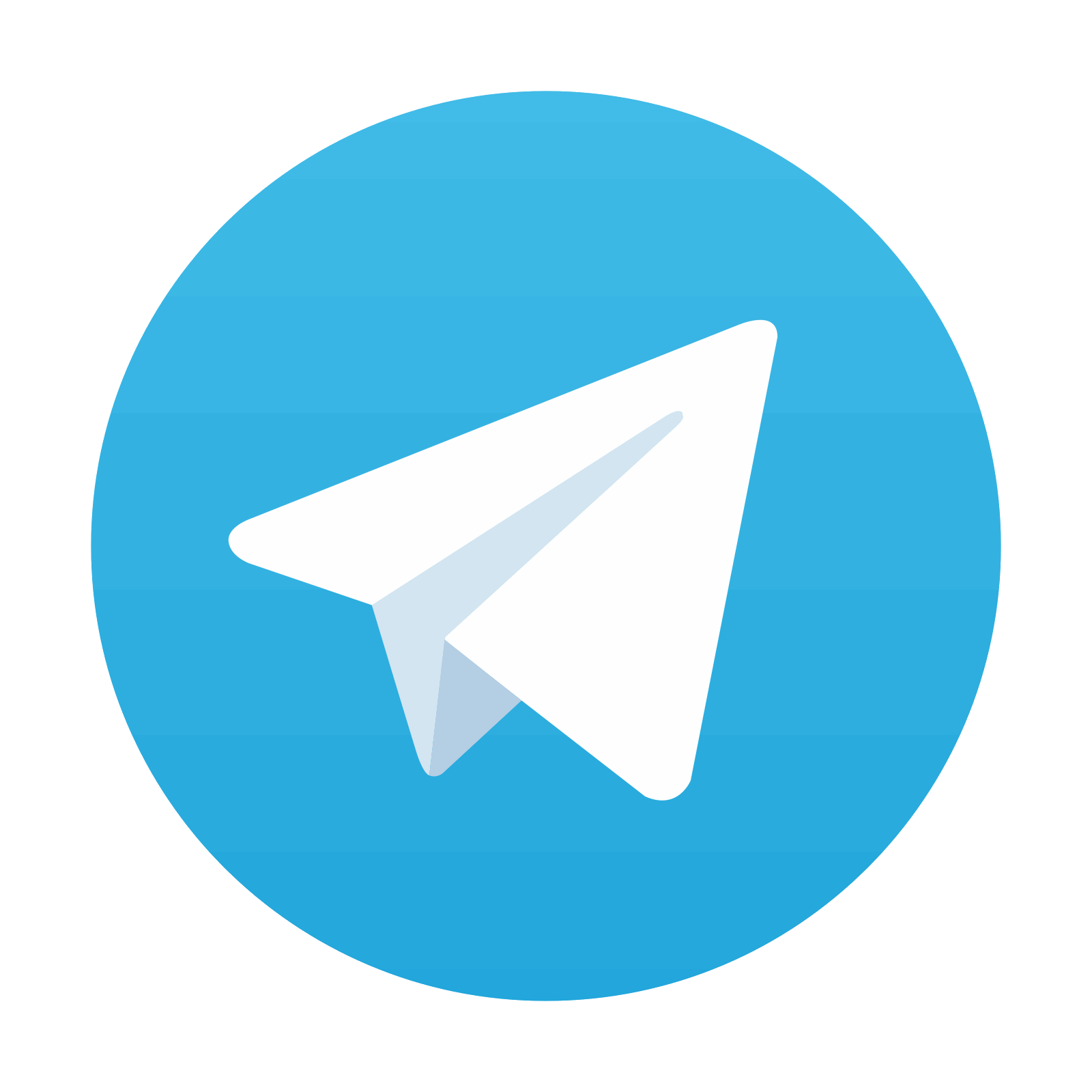
Stay updated, free articles. Join our Telegram channel

Full access? Get Clinical Tree
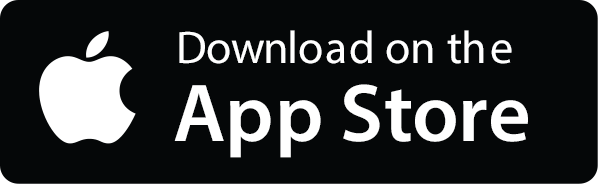
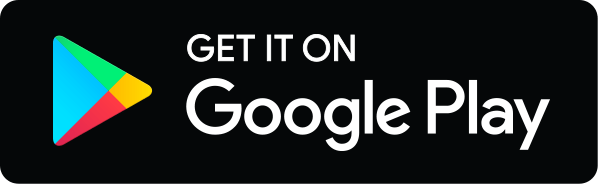