Recall that effective, efficient ventricular pumping action depends on proper cardiac function in five basic aspects. This chapter focuses on the abnormalities in three of these aspects: (1) abnormal cardiac excitation and rhythmicity, (2) valvular stenosis (inadequate valve opening), and (3) valvular insufficiency (incomplete valve closure). Discussion of abnormalities in myocardial force production and cardiac filling is presented in Chapter 11. The material presented here is an introduction to the more common cardiac electrical and cardiac valve dysfunctions, with an emphasis on the primary physiological consequences of these abnormal situations.
ELECTRICAL ABNORMALITIES AND ARRHYTHMIAS
Many cardiac excitation problems can be diagnosed from the information in a single lead of an electrocardiogram. The lead II electrocardiogram traces at the top of Figures 5–1 and 5–2 are identified as normal sinus rhythms based on the following characteristics: (1) the frequency of QRS complexes is approximately 1/s, indicating a normal beating rate; (2) the shape of the QRS complex is normal for lead II and its duration is less than 120 ms, indicating rapid depolarization of the ventricles via normal conduction pathways; (3) each QRS complex is preceded by a P wave of proper configuration, indicating sinoatrial (SA) nodal origin of the excitation; (4) the PR interval is less than 200 ms, indicating proper conduction delay of the impulse propagation through the atrioventricular (AV) node; (5) the QT interval is less than half of the R-to-R interval, indicating normal ventricular repolarization; and (6) there are no extra P waves, indicating that no AV nodal conduction block is present. The subsequent electrocardiographic tracings in Figures 5–1 and 5–2 represent irregularities commonly found in clinical practice. Examination of each of these traces with the above characteristics in mind will aid in the differential diagnosis.
Figure 5–1. Supraventricular arrhythmias.
Figure 5–2. Ventricular arrhythmias.
The physiological consequences of abnormal excitation and conduction in the heart depend on whether the electrical abnormality evokes a tachycardia, which will limit the time for cardiac filling between beats; evokes a bradycardia, which is inadequate to support sufficient cardiac output; or decreases the coordination of myocyte contraction, which will reduce stroke volume.
Supraventricular Abnormalities
Traces 2 through 6 below the normal trace in Figure 5–1 represent typical supraventricular arrhythmias (ie, originating in the atria or AV node). Supraventricular tachycardia (shown in trace 2 in Figure 5–1 and sometimes called paroxysmal atrial tachycardia) occurs when the atria are abnormally excited and drive the ventricles at a very rapid rate. These paroxysms often begin abruptly, last for a few minutes to a few hours, and then, just as abruptly, disappear and the heart rate reverts to normal. QRS complexes appear normal (albeit frequent) with simple paroxysmal atrial tachycardia because the ventricular conduction pathways operate normally. The P and T waves may be superimposed because of the high heart rate. Low blood pressure and dizziness may accompany bouts of this arrhythmia because the extremely high heart rate does not allow sufficient diastolic time for ventricular filling.
There are two mechanisms that may account for supraventricular tachycardia. First, an atrial region, often outside the SA node, may become irritable (perhaps because of local interruption in blood flow) and begin to fire rapidly to take over the pacemaker function. Such an abnormal pacemaker region is called an ectopic focus. Alternatively, atrial conduction may become altered so that a single wave of excitation does not die out but continually travels around some abnormal atrial conduction loop. In this case, the continual activity in the conduction loop may drive the atria and AV node at a very high frequency. This self-sustaining process is called a reentry phenomenon and is illustrated in Figure 5–3. This situation may develop as a result of abnormal repolarization and altered refractory periods in local areas of the myocardium. Atrial flutter is a special form of tachycardia of atrial origin in which a large reentrant pathway drives the atria at very fast rates (250-300 beats/min) and normal refractory periods of AV nodal tissue are overwhelmed. Thus, ventricular rate is often some fixed ratio of the atrial rate (2:1, 4:1) with frequencies often 150 to 220 beats/min. The electrocardiogram often shows a saw-tooth pattern of merged P waves with intermittent normal QRS complexes.
Figure 5–3. Normal and reentrant (circus) cardiac excitation pathways.
Conduction blocks occur at the AV node and generally represent impaired conduction through this tissue. In a first-degree heart block (trace 3 in Figure 5–1), the only electrical abnormality is unusually slow conduction through the AV node. This condition is detected by an abnormally long PR interval (>0.2 s). Otherwise, the electrocardiogram may be completely normal. At normal heart rates, the physiological effects of the first-degree block are inconsequential. The danger, however, is that the slow conduction may deteriorate to an actual interruption of conduction.
A second-degree heart block (trace 4 in Figure 5–1) is said to exist when some, but not all, atrial impulses are transmitted through the AV node to the ventricle. Impulses are blocked in the AV node if the cells of the region are still in a refractory period from a previous excitation. In this situation, the primary problem is a slower-than-normal conduction through the AV nodal region and thus the second-degree block is aggravated by high atrial rates. In the second-degree block, some, but not all, P waves are accompanied by corresponding QRS complexes and T waves. Atrial rate is often faster than ventricular rate by a certain ratio (eg, 2:1, 3:1, and 4:1). This condition may not represent a serious clinical problem as long as the ventricular rate is adequate to meet the pumping needs.
In a third-degree heart block (trace 5 in Figure 5–1), no impulses are transmitted through the AV node. In this event, some area in the ventricles—often in the common bundle or bundle branches near the exit of the AV node—assumes the pacemaker role for the ventricular tissue. Atrial rate and ventricular rate are completely independent, and P waves and QRS complexes are totally dissociated in the electrocardiogram. Ventricular rate is very likely to be slower than normal (bradycardia) and is often slow enough to impair cardiac output.
Atrial fibrillation (trace 6 in Figure 5–1) is characterized by a complete loss of the normally close synchrony of the excitation and resting phases between individual atrial cells. Cells in different areas of the atria depolarize, repolarize, and are excited again randomly. Consequently, no P waves appear in the electrocardiogram, although there may be rapid, irregular, small waves apparent throughout diastole. The ventricular rate is often very irregular in atrial fibrillation because impulses enter the AV node from the atria at unpredictable times. Fibrillation is a self-sustaining process. The mechanisms behind it are not well understood, but impulses are thought to progress repeatedly around irregular conduction pathways (sometimes called circus pathways, which imply a reentry phenomenon as described earlier and in Figure 5–3). However, because atrial contraction usually plays a negligible role in ventricular filling, atrial fibrillation may be well tolerated by most patients as long as ventricular rate is sufficient to maintain the cardiac output.1
Ventricular Abnormalities
Traces 2 through 6 below the normal trace in Figure 5–2 show typical ventricular electrical abnormalities. Conduction blocks called bundle branch blocks or hemiblocks (trace 2 in Figure 5–2) can occur in either of the branches of the Purkinje system of the intraventricular septum, often as a result of a myocardial infarction. Ventricular depolarization is less synchronous than normal in the half of the heart with the nonfunctional Purkinje system. This results in a widening of the QRS complex (>0.12 s) because a longer time is required for ventricular depolarization to be completed. The direct physiological effects of bundle branch blocks are usually inconsequential.
Premature ventricular contractions (PVCs) (trace 3 in Figure 5–2) are caused by action potentials initiated by and propagated away from an ectopic focus in the ventricle. As a result, the ventricle depolarizes and contracts before it normally would. A PVC is often followed by a missed beat (called a compensatory pause) because the ventricular cells are still refractory when the next normal impulse emerges from the SA node. The highly abnormal ventricular depolarization pattern of a PVC produces the large-amplitude, long-duration deflections on the electrocardiogram. The shapes of the electrocardiographic records of these extra beats are highly variable and depend on the ectopic site of their origin and the depolarization pathways involved. The volume of blood ejected by the premature beat itself is smaller than normal, whereas the stroke volume of the beat following the compensatory pause is larger than normal. This is partly due to the differences in filling times and partly to an inherent phenomenon of the cardiac muscle called postextrasystolic potentiation. Single PVCs occur occasionally in most individuals and, although sometimes alarming to the individual experiencing them, are not dangerous. Frequent occurrence of PVCs, however, may be a signal of possible myocardial damage or perfusion problems.
Ventricular tachycardia (trace 4 in Figure 5–2) occurs when the ventricles are driven at high rates, usually by impulses originating from a ventricular ectopic focus. Ventricular tachycardia is a very serious condition. Not only is diastolic filling time limited by the rapid rate, but also the abnormal excitation pathways make ventricular contraction less synchronous and therefore less effective than normal. In addition, ventricular tachycardia often precedes ventricular fibrillation.
Prolonged QT intervals (the left side of trace 5 in Figure 5–2) are a result of delayed ventricular myocyte repolarization, which may be due to inappropriate opening of sodium channels or prolonged closure of potassium channels during the action potential plateau phase. Although the normal QT interval varies with heart rate, it is normally less than 40% of the cardiac cycle length (except at very high heart rates). Long QT syndrome is identified when the QT interval is greater than 50% of the cycle duration. It may be genetic in origin (mutations influencing various ion channels involved with cardiac excitability), may be acquired from several electrolyte disturbances (low blood levels of Ca2+, Mg2+, or K+), or may be induced by several pharmacological agents (including some antiarrhythmic drugs). The prolongation of the myocyte refractory period, which accompanies the long QT syndrome, extends the vulnerable period during which extra stimuli can evoke tachycardia or fibrillation. Patients with long QT syndrome are predisposed to a particularly dangerous type of ventricular tachycardia called torsades de pointes (“twisting of points,” as shown on the right side of trace 5 in Figure 5–2). This differs from the ordinary ventricular tachycardia in that the ventricular electrical complexes cyclically vary in amplitude around the baseline and can deteriorate rapidly into ventricular fibrillation.
In ventricular fibrillation (trace 6 in Figure 5–2), various areas of the ventricle are excited and contract asynchronously. The mechanisms are similar to those in atrial fibrillation. The ventricle is especially susceptible to fibrillation whenever a premature excitation occurs at the end of the T wave of the previous excitation, that is, when most ventricular cells are in the “hyperexcitable” or “vulnerable” period of their electrical cycle. In addition, because some cells are repolarized and some are still refractory, circus pathways can be triggered easily at this time. Because no pumping action occurs with ventricular fibrillation, the situation is fatal unless quickly corrected by cardiac conversion. During conversion, the artificial application of large currents to the entire heart (via paddle electrodes applied across the chest) may be effective in depolarizing all heart cells simultaneously, thus allowing a normal excitation pathway to be reestablished.
CARDIAC VALVE ABNORMALITIES
Pumping action of the heart is impaired when the valves do not function properly. Abnormal heart sounds, which often accompany cardiac valvular defects, are called murmurs. These sounds are caused by abnormal pressure gradients and turbulent blood flow patterns that occur during the cardiac cycle. A number of techniques, ranging from simple auscultation (listening to the heart sounds) to echocardiography or cardiac catheterization, are used to obtain information about the nature and extent of these valvular malfunctions.
In general, when a valve does not open fully (ie, is stenotic), the chamber upstream of the valve has to develop more pressure during its systolic phase to achieve a given flow through the valve. This increase in “pressure” work will induce hypertrophy of cardiac muscle cells and thickening of the walls of that chamber. When a valve does not close completely (ie, is insufficient), the regurgitant blood flow represents an additional volume that must be ejected in order to get sufficient forward flow out of the ventricle into the tissues. This increase in “volume” work often leads to chamber dilation but not to an increase in wall thickness.2
A second generality about valve abnormalities is that whenever there is an elevation in the atrial pressure as a result of AV valve stenosis or regurgitation, this will result in higher pressures in the upstream capillary beds. If capillary hydrostatic pressures are elevated, tissue edema will ensue with consequences on the function of those upstream organs.
A brief overview of four of the common valve defects influencing left ventricular function is given in Figure 5–4. Note that similar stenotic and regurgitant abnormalities can occur in right ventricular valves with similar consequences on right ventricular function.
Figure 5–4. Characteristics of left ventricular valve abnormalities: (A) aortic stenosis, (B) mitral stenosis, (C) aortic regurgitation (insufficiency), and (D) mitral insufficiency.
Aortic Stenosis
Some characteristics of aortic stenosis are shown in Figure 5–4A. Normally, the aortic valve opens widely and offers a pathway of very low resistance through which blood leaves the left ventricle. If this opening is narrowed (stenotic), resistance to flow through the valve increases. A significant pressure difference between the left ventricle and the aorta may be required to eject blood through a stenotic aortic valve. As shown in Figure 5–4A, intraventricular pressures may rise to very high levels during systole, while aortic pressure rises more slowly than normal to a systolic value that is subnormal. Pulse pressure is usually low with aortic stenosis. High intraventricular pressure development is a strong stimulus for cardiac muscle cell hypertrophy, and an increase in left ventricular muscle mass invariably accompanies aortic stenosis. This tends to produce a leftward deviation of the electrical axis. (The mean electrical axis will fall in the upper right-hand quadrant in Figure 4–6.) Blood being ejected through the narrowed orifice may reach very high velocities, and turbulent flow may occur as blood enters the aorta. This abnormal turbulent flow can be heard as a systolic (or ejection) murmur with a properly placed stethoscope. The primary physiological consequence of aortic stenosis is a high ventricular afterload that is caused by restriction of the outflow tract. This imposes an increased pressure workload on the left ventricle.
Mitral Stenosis
Some characteristics of mitral stenosis are shown in Figure 5–4B. A pressure difference of more than a few millimeters of mercury across the mitral valve during diastole is distinctly abnormal and indicates that this valve is stenotic. The high resistance mandates an elevated pressure difference to achieve normal flow across the valve (
= ΔP/R). Consequently, as shown in Figure 5–4B, left atrial pressure is elevated with mitral stenosis. The high left atrial workload may induce hypertrophy of the left atrial muscle. Elevated left atrial pressure is reflected back into the pulmonary bed and, if high enough, causes pulmonary congestion and “shortness of breath.” A diastolic murmur associated with turbulent flow through the stenotic mitral valve can often be heard. The primary physiological consequences of mitral stenosis are elevations in left atrial pressure and pulmonary capillary pressure. The latter can cause pulmonary edema and interference with normal gas exchange in the lungs (leading to shortness of breath).
Aortic Insufficiency
Typical characteristics of aortic regurgitation (also known as insufficiency or incompetence) are shown in Figure 5–4C. When the leaflets of the aortic valve do not provide an adequate seal, blood regurgitates from the aorta back into the left ventricle during the diastolic period. Aortic pressure falls faster and further than normal during diastole, which causes a low diastolic pressure and a large pulse pressure. In addition, ventricular end-diastolic volume and pressure are higher than normal because of the extra blood that reenters the chamber through the incompetent aortic valve during diastole. Turbulent flow of the blood reentering the left ventricle during early diastole produces a characteristic diastolic murmur. Often the aortic valve is altered so that it is both stenotic and insufficient. In these instances, both a systolic and a diastolic murmur are present. The primary physiological consequences of aortic insufficiency are reduction in forward flow out to the tissues (if the insufficiency is severe) and increase in the volume workload of the left ventricle.
Mitral Regurgitation
Typical characteristics of mitral regurgitation (insufficiency, incompetence) are shown in Figure 5–4D. When the mitral valve is insufficient, some blood regurgitates from the left ventricle into the left atrium during systole. A systolic murmur may accompany this abnormal flow pattern. Left atrial pressure is raised to abnormally high levels, and left ventricular end-diastolic volume and pressure increase. Mitral valve prolapse is the most common form of mitral insufficiency in which the valve leaflets evert into the left atrium during systole. The primary physiological consequences of mitral regurgitation are somewhat similar to aortic insufficiency in that forward flow out of the left ventricle into the aorta may be compromised (if the insufficiency is severe) and there is an increase in the volume workload of the left ventricle. In addition, the elevated left atrial pressure can also lead to pulmonary effects with shortness of breath.
PERSPECTIVES
It might be useful for the student to be reminded that the heart is “simply” a pulsatile pressure-generating pump producing one-way flow through a closed hydraulic system. There are many ways that this pump can fail, and the information in this chapter just touches on the critical role of the excitation process (which sets the “speed” of the pump) and the valves (that assure forward flow). Many of the physiological consequences of abnormalities in these processes (ie, inadequate output and lowered pressure) are easily predicted from a basic consideration of fluid dynamics.
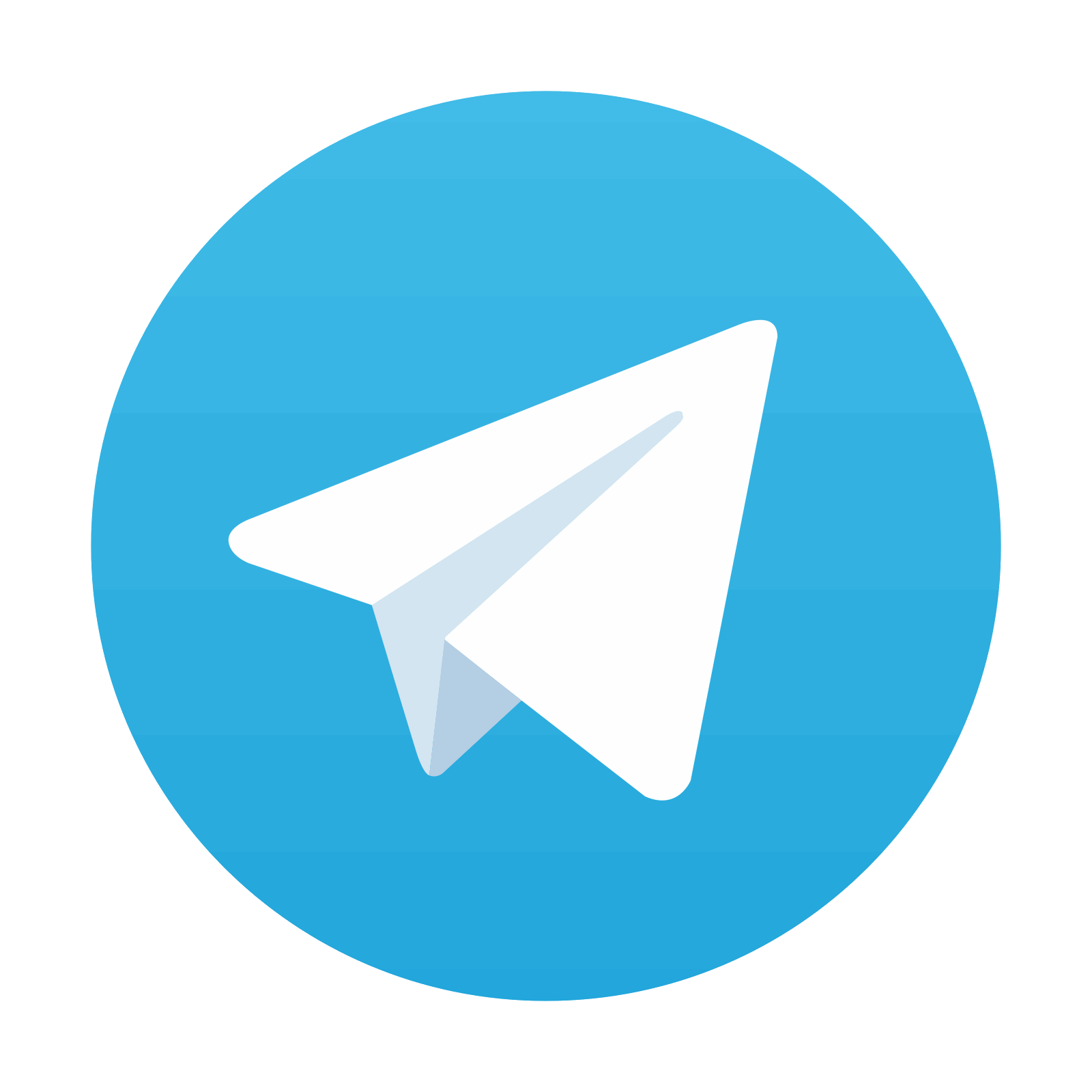
Stay updated, free articles. Join our Telegram channel

Full access? Get Clinical Tree
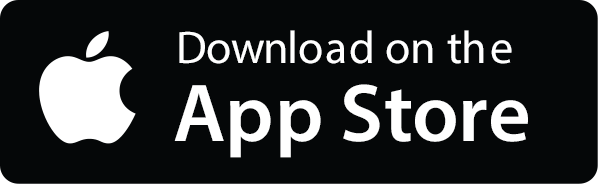
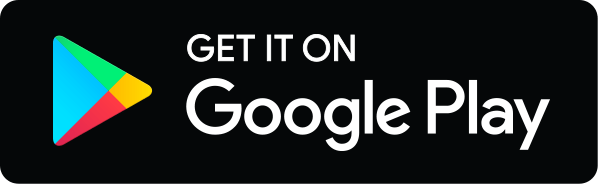