Airway obstruction
Patients found to have impending or active airway obstruction are often noted to have alteration of mental status or to exhibit stridorous breathing, dysphonia, tachypnea, indrawing, or accessory muscle use. Urgent placement of a definitive airway is indicated for those patients who are found to be apneic, hypoxemic, or obtunded (GCS < 8) or who are noted to have complex facial injuries, thermal inhalation injuries, penetrating injuries to the neck, or expanding or pulsatile cervical hematomas
Tension pneumothorax
Blunt or penetrating injuries to the thorax may result in airway, lung parenchymal or pleural disruption, and subsequent escape of air to the pleural space. If the injury creates a “one-way valve” with air entering the negative pressure environment of the pleural space, but prevented from returning to the airway, air trapping will occur. Positive pleural pressure creates an extremely dangerous situation of respiratory and hemodynamic compromise due to alterations in respiratory mechanics and venous return. Positive pressure ventilation can hasten this process or convert apparently simple pneumothoraces to tension pneumothoraces (TPs). Patients with diminished air entry and evidence of hemodynamic instability should undergo immediate pleural decompression. TPs require immediate placement of chest tubes on the side of diminished air entry
Failure of the lung to re-expand with well-placed chest tubes on 20 cm H2O suction, persistent air leaks, or the observation of hemoptysis or blood in the endotracheal tube should raise the concern that a more proximal injury to the tracheobronchial tree has occurred. In such instances, a second chest tube may be required, and evaluation for airway injuries by flexible bronchoscopy should be undertaken. Bronchoscopy-guided interventions in the operating room such as selective bronchial intubation or placement of dual-lumen tubes or bronchial blockers may allow protection and selective ventilation of the uninjured lung until definitive measures can be undertaken
Massive hemothorax
Penetrating and sometimes blunt injuries to the chest can cause the accumulation of greater than 1,500 mL of blood in the pleural space. Blood loss of this magnitude in the chest can present with symptoms and signs of respiratory compromise and profound shock. When a chest tube, placed in the setting of hemodynamic instability, evacuates 1,500 mL of blood immediately or continues to drain 200–300 mL/h, ongoing bleeding is likely and preparations for exploratory thoracotomy should be made. Such preparations include reassessment of the patient’s airway, breathing, and circulation; thorough evaluation for associated injuries, which may contribute to ongoing hemorrhage; and meticulous efforts to warm the patient and treat transfusion-associated coagulopathy
Note: Rapid exsanguinations can occur into the chest without significant chest tube output if the tube is clogged or kinked
Physicians attempting to rule out ongoing sources of hemorrhage should not be reassured by low volume chest tube output, and patients must be frequently reexamined and re-X-rayed as necessary
Open pneumothorax
High-velocity weapons or high-speed blunt injury mechanisms can cause full thickness injury to the chest wall. Gaps in the chest wall may permit air entry into the pleural space, especially during spontaneous inspiration when intrapleural pressures are negative. When a chest wall defect reaches roughly two-thirds the diameter of the trachea, pleural ventilation may become the path of least resistance during inspiration, and normal bronchial air flow may become extremely compromised. Patients with this injury present with respiratory distress and a “sucking chest wound”
Early therapeutic priorities for these patients include airway management, coverage of the wound, and pleural drainage
Cardiac tamponade
Depending on the distensibility of the pericardium as little as 60–100 mL of blood in the pericardial sac can increase pressures around the heart sufficient to limit venous return to the right atrium. Obstructive shock from loss of cardiac preload can initially be compensated by sympathetic measures directed at maintaining cardiac output (tachycardia, vasoconstriction of the venous bed). However, unless mounting pressure is relieved, dramatic decreases in cardiac output and coronary perfusion can ensue. This condition is known as cardiac tamponade
Flail chest
Multiple rib fractures may result in segmental instability of the chest wall. When blunt forces disrupt the continuity of ribs, costal cartilage, or sternum at more than one site, the intervening chest wall can remain stationary or even be drawn inward at inspiration. This phenomenon is known as flail chest (FC). The high-energy transfers required to create a flail segment often result in significant associated injuries such as pulmonary contusion (50 %) and hemopneumothorax (70 %), as well as head, abdominal, pelvic, and extremity injuries. Acute hypoxemia and respiratory failure is seen in those FC patients with associated pulmonary contusions, and flail segments may, in fact, become more evident as more respiratory effort is required with blossoming contusions. The acute and chronic severity of FC is primarily dependent on the magnitude of the underlying pulmonary contusion rather than on abnormalities of the chest wall
The diagnosis of FC is clinical—observation of segmental paradoxical chest wall movement with respiration requires adequate exposure and careful examination during all phases of spontaneous breathing. The cornerstones of therapy for FC are close observation and reexamination for signs of deterioration of respiratory function, use of humidified supplemental oxygen and mechanical ventilation as necessary for support of gas exchange, optimization of analgesia, and prompt treatment of associated injuries
The damage control resuscitation concept evolved in response to the recognition of hemorrhage and coagulopathy as a common and potent complication of severe trauma. The strategy encompasses three resuscitation priorities: (1) setting modest blood pressure and perfusion targets until hemostasis is achieved in order to avoid the adverse effects of excess fluid administration, (2) minimization of crystalloid use and early transition of resuscitation to blood component therapy to restore oxygen-carrying capacity and normalize coagulation, and (3) damage control surgery. Surgery emphasizes three key operative priorities: minimization of operative time with early transfer to ICU (for shock resuscitation, correction of coagulopathy, and reversal of hypothermia), rapid control of hemorrhage, and temporizing control of contamination [1–4].
Surgeons caring for patients with severe chest wall trauma will be entering this complex milieu and may be required to adjust their approach based on associated injuries and prevailing priorities. The timing of reconstructive surgery in multiple injured patients will depend on the establishment of effective oxygenation, the reversal of shock, the correction of coagulopathy, and will require coordination with critical care and other surgical services completing other operative interventions. In general, the lateral decubitus positioning required for many chest wall reconstructive procedures does not provide adequate access for damage control procedures and may not be tolerated in patients with pulmonary injuries. Chest wall reconstruction is therefore left until all acute interventions have been completed and homeostasis has been achieved.
Diagnostic Approaches and Advances
Chest Radiograph
The portable chest radiograph (CXR) is one of the first adjuncts to the primary survey advocated by ATLS. In most trauma bays, it is convenient and fast and reveals major early threats to life. There are, however, limitations to this imaging modality. In a blunt trauma patient with a significant mechanism of injury, full spine precautions have to be maintained. The CXR is thus performed supine and anteroposterior, which decreases its accuracy. As a result, the trauma room CXR may miss up to half of pneumothoraces [5, 6]. Up to 65 % of patients with significant blunt chest trauma will have significant chest trauma missed by CXR alone [7]. Despite these limitations, a CXR can diagnose large hemopneumothoraces and lung contusions and should still be part of the initial investigation.
Ultrasound
Ultrasound (US) is increasingly used in the trauma and critical care setting. It has multiple advantages including portability, versatility, steep learning curve, and good accuracy. Smaller probes with greater image quality have made this diagnostic tool an essential in the trauma bay. The addition of the thoracic component to the Focused Assessment with Sonography for Trauma (FAST) has been called the extended FAST (E-FAST). Using a high-frequency probe (10–15 MHz), multiple sonographic signs have been described to detect pneumothoraces: absence of pleural sliding, comet-tail artifact, B lines, and the presence of A lines. The most specific sign is the “lung point,” which defines the border of the pneumothorax (Fig. 10.1). The same findings can be obtained with the standard low-frequency probe (2.5–5 MHz) used for FAST. Multiple studies have shown that US has better sensitivity and specificity than CXR for detection of pneumothorax [8, 9]. A recent systematic review reports a sensitivity ranging from 86 % to 98.2 % and a specificity ranging from 97.2 % to 100 % [10]. Thoracic ultrasound can also detect a hemothorax. This diagnostic modality remains accurate even in the setting of severe chest trauma with multiple rib fractures, as long as there is an adequate window to visualize the pleura.
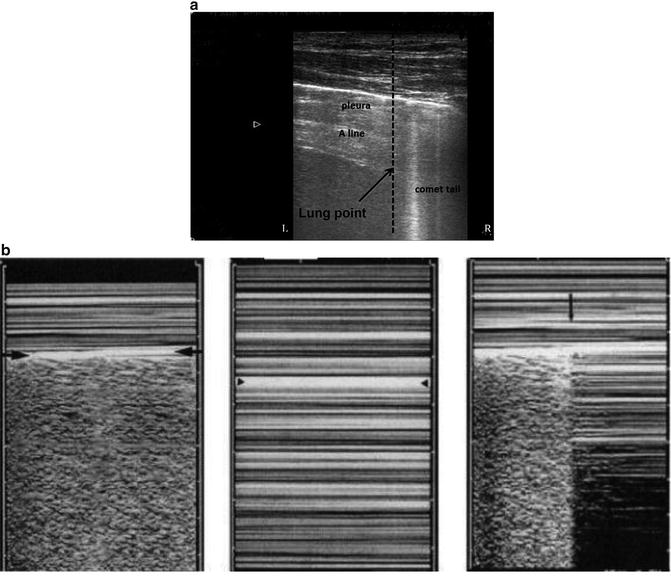
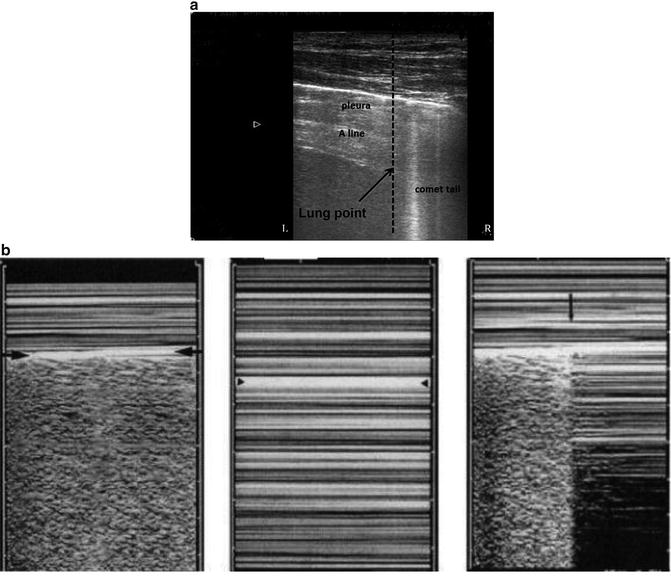
Fig. 10.1
Thoracic ultrasound. (a) A 10 MHz probe is used to image the pleural surface in a patient with shortness of breath after blunt chest trauma. The bright horizontal line represents the pleura. The horizontal reverberations are referred to as “A” lines, which are a sign of pneumothorax. Similarly, absence of rib shadowing, also called “comet-tail artifact,” is typical of pneumothorax. This image thus depicts the lung point: on the left side is a pneumothorax, on the right side normal lung. (b) The lung point, depicted by the arrow in the third image (right-hand side), is 100 % specific for pneumothorax. In M-mode, normal lung has a “seashore” appearance (image 1). In a pneumothorax, there is no pleural sliding; thus, the granular pattern created by the pleural movement is absent
Another use of the US is the limited transthoracic echocardiography (LTTE), which is gaining popularity in the trauma environment. It allows for a quick assessment of the patient’s volume status, cardiac function, and presence of pericardial effusion. Three views are obtained: the parasternal long and short axis, the apical window, and the subxiphoid window. A recent study by Ferrada et al. reported a 100 % accuracy for pericardial effusion and global heart function [11]. Most patients in this study sustained blunt trauma, and several had a chest tube, previous sternotomy or thoracotomy. All exams were performed in less than 5 min by non-ultrasound trained trauma attendings.
We suggest that US should always be performed in the blunt trauma patient with severe chest injuries, as it is a noninvasive and quick method to diagnose hemopneumothorax and pericardial effusion. An added benefit of this diagnostic test is the assessment of cardiac contractility and volume status.
Computed Tomography
Computed tomography (CT) for chest trauma is the gold standard against which all other imaging modalities are compared. A large retrospective study of more than 1,047 trauma patients showed that a positive CXR was the most significant predictor of finding associated thoracic injuries on CT (OR 15.6; p < 0.001) [12]. All patients with multiple rib fractures and/or flail chest should have a CT of the chest with intravenous contrast. In patients with significant mechanisms (fall greater than 5 m, pedestrian struck thrown more than 3 m, high-speed motor vehicle crash), a CT angiography of the chest is recommended to rule out blunt thoracic aortic injury. At the Vancouver General Hospital, we perform a rapid imaging protocol in trauma (RIPIT): a whole-body dual-source CT with fast reconstruction time at 40 images per second [13]. After a non-contrast CT of the head, a contiguous whole-body arterial phase from the vertex to the pelvis is performed, which gives good quality images of the thoracic aorta and adjacent structures. With the presence of the CT scanner adjacent to the trauma bay and new advances in technology, the whole-body CT can be completed in 5 min.
Injuries Commonly Associated with Chest Wall Trauma
Mediastinum
Blunt Cerebrovascular Injury
Blunt cerebrovascular injuries (BCVIs) to the extracranial carotid and vertebral arteries, which are thought to result from neck hyperflexion, hyperextension, or rotational mechanisms, occur in about 1 % of hospitalized trauma patients and are associated with increased risk of potentially devastating ischemic strokes, both on admission and within days of injury. Classic studies have shown that certain injury patterns, including traumatic brain injury, complex skull fractures, facial or mandibular fractures, cervical spine fractures, neck soft-tissue injuries, and thoracic or thoracic vascular injuries, are associated with BCVIs and can be used as criteria for screening with CT angiography and/or digital subtraction angiography [14–16]. However, it is estimated that 30 % of patients with BCVIs do not meet any of these screening criteria, and some investigators advocate for more liberal screening, even based on suspicious mechanism alone [17]. Surgeons caring for patients with major thoracic trauma should ensure that their patients have been screened for BCVI, and started on prophylactic antiplatelet or anticoagulant therapy as soon as the risks of stroke (thought to be 21 % in untreated patients) [18] begin to outweigh the risks of anticoagulation-associated hemorrhage.
Blunt Aortic Injury
Blunt thoracic aortic injury (BTAI) is most often caused by rapid deceleration, from motor vehicle or motorcycle crashes. Other common mechanisms include fall from height more than 5 m, crush between two objects, and ejection from a vehicle. Only 20 % of patients with BTAI will survive to the hospital. The injury usually occurs on the descending thoracic aorta, just distal to the takeoff of the left subclavian artery. The diagnosis can be suspected on CXR, with suggestive signs like widened mediastinum, apical cap, loss of aortic knob, or a depressed left main stem bronchus (Fig. 10.2). However, these signs may not be obvious on a supine anteroposterior CXR and are not specific to BTAI. As discussed above, the trauma surgeon should have a low threshold for performing a CT scan in patients with serious mechanism and/or suspicious findings on CXR. Helical CT angiography is the gold standard for diagnosing these injuries. If equivocal, a formal angiogram can be performed. The treatment of BTAI has drastically evolved over the course of the past decades. Two large prospective studies from the American Association for the Surgery of Trauma have redefined the optimal management of this injury [19, 20]. They have shown, first, that delayed repair improves outcomes and, second, that the endovascular stent graft technique has less morbidity and mortality than conventional open repair. A recent study reports 0 % 30-day mortality, no conversion to open repair, and a re-intervention rate of 5.8 % at 3 years with the endovascular approach [21]. In patients with multiple rib fractures and BTAI, priority should be given to patient resuscitation, blood pressure control, and semi-urgent endovascular repair. Rib plating should only be done once the aortic injury has been repaired.
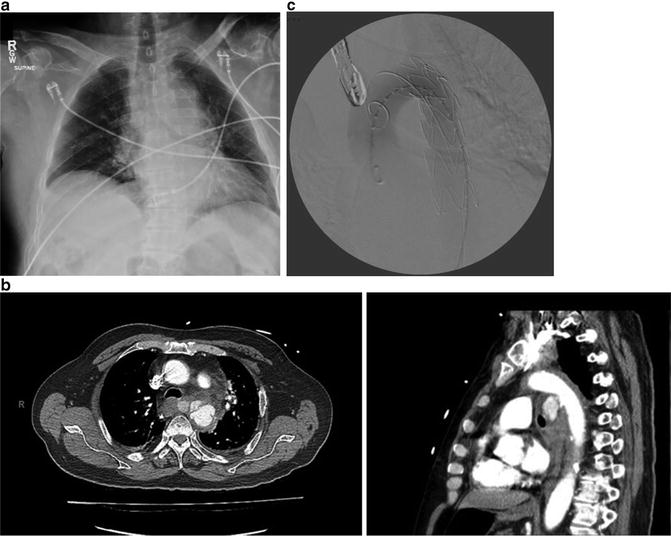
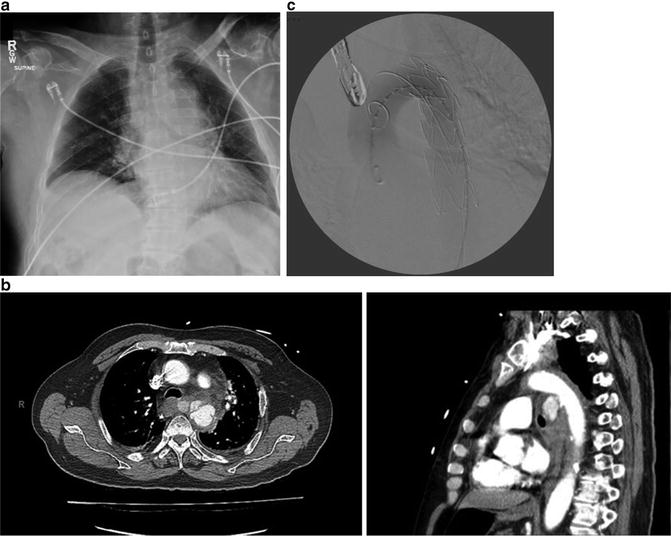
Fig. 10.2
Blunt thoracic aortic injury. An 83-year-old patient was the passenger of a car which crashed into an electric pole, at 70 km/h. He was hemodynamically stable but complained of mild chest pain. The CXR is shown in (a). He subsequently underwent a CT scan, shown in (b). The patient had a hypoxic arrest shortly after the CT and required emergent intubation and thoracic endovascular repair (c)
Blunt Cardiac Injury
Patients sustaining motor vehicle crashes, motorcycle crashes, and high-level falls (≥20 feet), as well as pedestrians that are hit by cars, and certainly all patients with significant chest wall trauma, are at risk of blunt cardiac injury (BCI). The clinical manifestations of BCI can be structural, from trauma to the structural elements of the heart, or electrical, from injuries to the heart’s electrical conduction system. From a structural standpoint, injuries include transmural rupture (which is usually fatal at the scene), valvular rupture causing acute heart failure and necessitating valve repair or replacement, coronary artery dissection necessitating immediate revascularization procedures, and intramural hematomas, which often resolve with simple expectant management. Electrical disturbances seen in BCI include commotio cordis, a trauma-induced conduction abnormality that is rapidly fatal in the vast majority of cases, as well as atrial and ventricular dysrhythmias and bundle branch block, which often require pharmacological control, cardioversion, or pacing, depending on their severity and hemodynamic effects [22].
BCIs are uncommon, but are of extreme clinical significance. Surgeons caring for patients with significant chest wall trauma should ensure that the possibility of BCI has been considered and ruled out or adequately addressed. A 12-lead ECG (an essential part of the diagnostic workup of chest trauma patients), when normal, has a 95 % negative predictive value for BCI [23], which increases to 100 % when combined with a normal cardiac troponin I [24]. Patients with new ECG abnormalities or troponin elevations require admission to monitored settings and may need additional investigation with echocardiography or cardiac catheterization, depending on the nature of the abnormalities and their hemodynamic status (Fig. 10.3). Such patients can still undergo urgent procedures, provided that their cardiac function can be monitored and optimized.
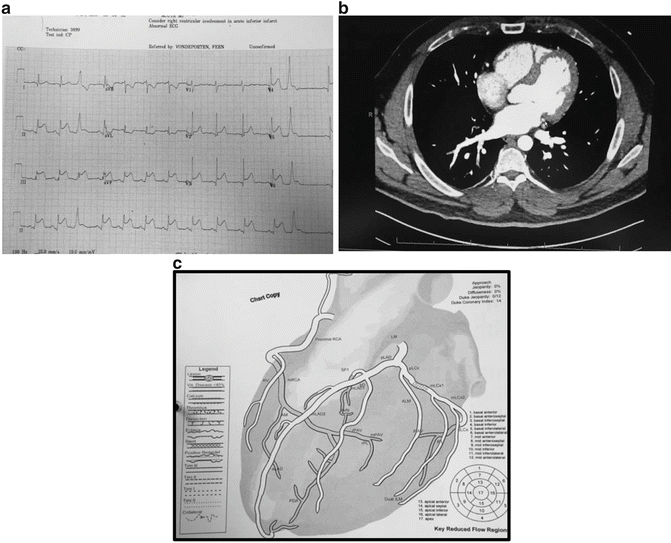
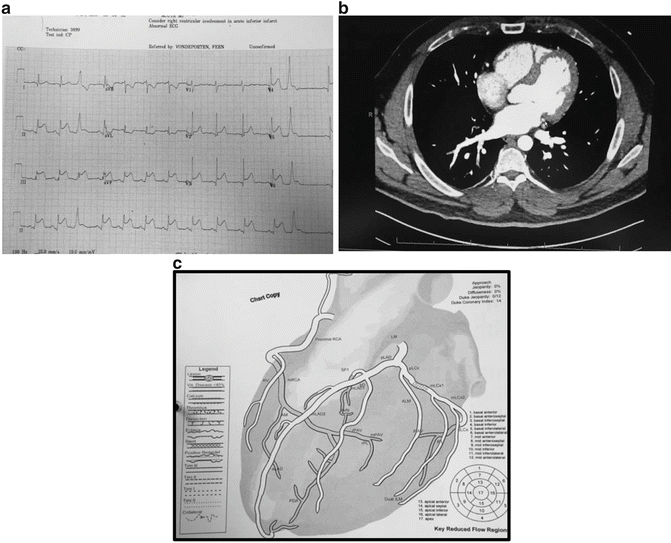
Fig. 10.3
Blunt cardiac injury. A healthy 34-year-old cyclist fell at high speed, striking his chest and sustaining numerous rib fractures. He completed his ride, but soon afterward, felt heavy retrosternal chest pain. (a) 12-lead ECG demonstrates inferior ST segment elevation. (b) Cardiac CT reveals occlusion of the left circumflex artery, likely as a result of plaque rupture and dissection resulting from impact. (c) This segment was revascularized using a stent
Esophageal Injury
Blunt esophageal injury is very rare, estimated to be present in 0.1 % of trauma admissions [25], possibly because the esophagus is protected between the aorta and vertebral column in the posterior mediastinum. Blunt esophageal injuries are caused by an increased pressure in the lumen against a closed upper or lower esophageal sphincter, usually secondary to a blow to the epigastrium [26]. A less common cause of esophageal injury in blunt mechanisms is impalement by an adjacent thoracic vertebral osteophyte fracture. Although rare, esophageal injuries cause significant morbidity, especially if diagnosed late. A combination of clinical evaluation (odynophagia, chest pain, hematemesis, sepsis) and multiple imaging modalities is recommended for diagnosis: CXR, CT, water-soluble and barium esophagogram, and esophagoscopy [27]. Pneumomediastinum on CT should prompt consideration of investigation for esophageal trauma, if the clinical circumstances are suggestive. A thoracic esophageal injury should be approached with a posterolateral right or left thoracotomy, depending on the site of perforation. These cases are usually heavily contaminated and the repair is at high risk of dehiscence. If associated rib fractures are present, rib plating should be discouraged because of the high risk of hardware infection.
Lung and Tracheobronchial Tree
Pulmonary Contusion, Acute Respiratory Distress Syndrome, and Other Causes of Respiratory Failure
Respiratory failure in blunt chest trauma results from a combination of injury to the lung parenchyma (pulmonary contusion (PC)) and disruption of the chest wall (flail chest (FC)). Therapeutic efforts are therefore directed at the consequences of both types of injury.
PC is characterized by parenchymal hemorrhage, inflammation, and occlusion of alveoli with blood and pulmonary edema (Fig. 10.4) [28, 29]. Blood or fluid-filled alveoli are unable to participate in gas exchange, and blood shunting past these alveoli will remain deoxygenated. Large lung contusions create large shunt fractions and worse hypoxemia. The extent of pulmonary contusion correlates closely with the severity of hypoxemic respiratory failure, the risk of pneumonia, and the duration of mechanical ventilation. In the long term, patients with PC can develop fibrotic changes in the lung that correlate with worse pulmonary function.
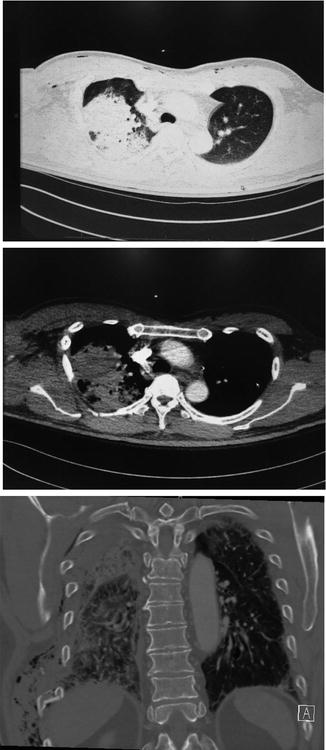
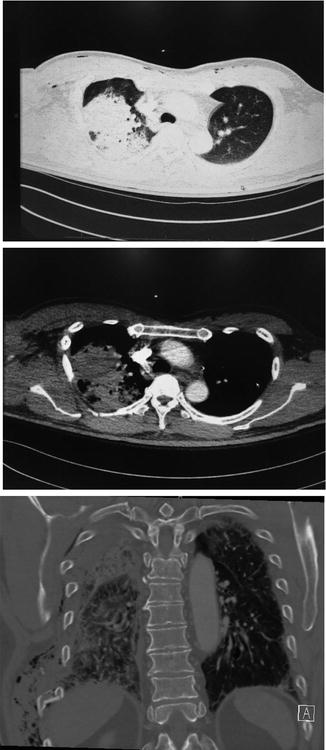
Fig. 10.4
Pulmonary contusion. A 43-year-old male was involved in a head on motor vehicle crash. In addition to a mild traumatic brain injury, he sustained rib fractures and a right upper lobe pulmonary contusion. He was intubated for airway protection and placed on positive pressure ventilation to maintain cerebral oxygenation in the context of hypoxemic respiratory failure from his pulmonary contusion. Chest wall reconstruction was deferred until the severity and course of the pulmonary contusion are determined
Supportive care with supplemental oxygen, adequate analgesia (including thoracic epidural analgesia) [30], chest physiotherapy, and the judicious use of intravenous fluids helps the hypoxemic effects of PC resolve in most cases. Positive pressure ventilation with positive end-expiratory pressure (PEEP) reduces shunt fraction and improves rib fracture apposition. However, invasive mechanical ventilation is associated with significant complications and high cost. In recent years, the practice of intubating all patients with significant pulmonary contusion has given way to the more selective use of intubation and mechanical ventilation as a safety net for PC patients who develop respiratory failure despite aggressive supportive care. In recent years, well-designed studies have shown that the liberal use of noninvasive modes of positive pressure ventilation, such as continuous positive airway pressure (CPAP), is effective in reducing atelectasis, pneumonia, respiratory failure, and the need for invasive mechanical ventilation [29]. A multidisciplinary approach to PC, which includes the use of noninvasive positive pressure ventilation as needed, along with bronchial hygiene, chest physiotherapy, early mobilization, and conscientious pain control has been shown to drastically reduce the need for invasive mechanical ventilation [31]. When a flail chest is present, rib fracture fixation may be an important adjunct in a multidisciplinary strategy, although the indications and timing of the intervention should be tailored to the specific injury patterns and clinical circumstances.
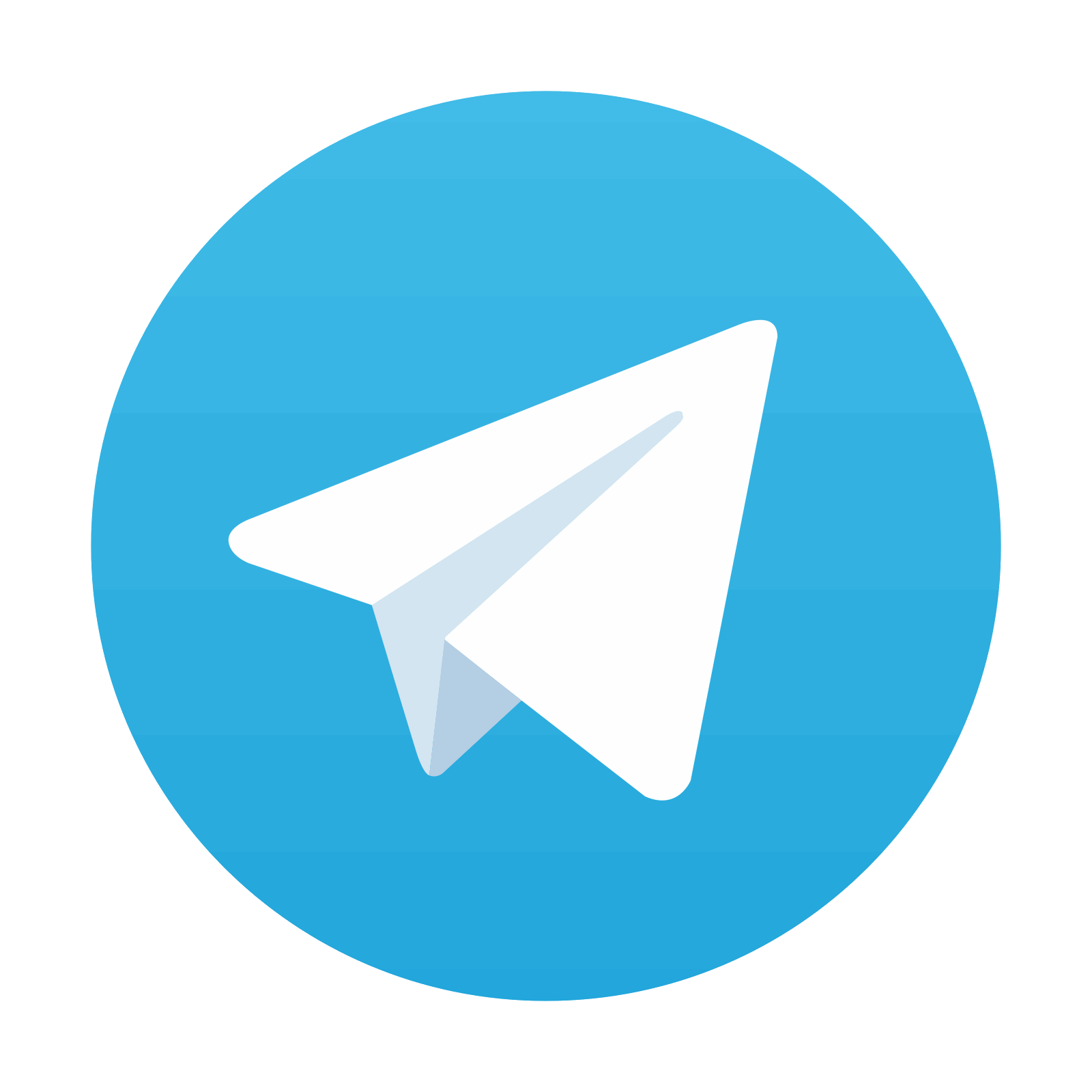
Stay updated, free articles. Join our Telegram channel

Full access? Get Clinical Tree
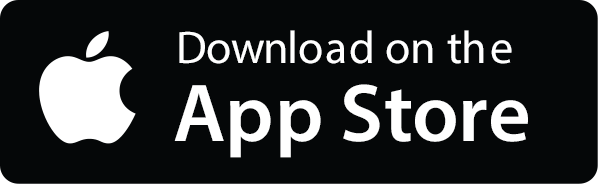
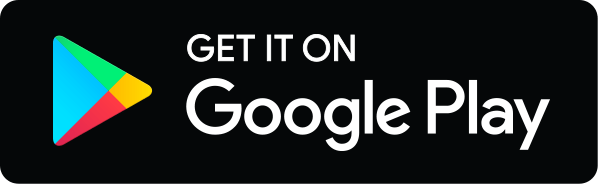