Chapter 61 Arrhythmias and Electrolyte Disorders
Cardiac arrhythmias are an expression of the same fundamental electrophysiological principles that underlie the normal electrical behavior of the heart. The electrical activity of the heart depends on transmembrane ionic gradients and the time-dependent and voltage-dependent alterations of their conductance. The resting membrane potential (Vm) is calculated by the Goldman constant field equation1:
which incorporates the permeability (P) and activity (a) of all ionic species that contribute to it.
Potassium
Potassium is the most abundant intracellular cation and the most important determinant of the resting membrane potential (RMP). The electrophysiological effects of potassium depend not only on its extracellular concentration, but also on the direction (hypokalemia versus hyperkalemia) and rate of change. Hoffman and Suckling have noted that the effect of potassium on the RMP is modulated by the simultaneous calcium concentration.2 The interrelationship is such that an elevated calcium level decreases the depolarizing effect of an elevated potassium level, and low calcium levels diminish the depolarization produced by hypokalemia. When extracellular potassium levels are higher than normal, the cell membrane behaves as a potassium electrode, as described by the Nernst equation:
At levels of less than ∼3 mmol/L, the transmembrane potential (Vm) is less than that predicted by the Nernst equation, because hypokalemia reduces membrane permeability to potassium (Pk).3
Indeed, potassium currents are modulated by the potassium gradient itself and other electrolytes as well (Table 61-1). The conductance of the inward rectifier current (iK1) is proportional to the square root of the extracellular potassium concentration [K+]o.4,5 The dependence of the activation of the delayed rectifier current (IKr) on the extracellular potassium concentration [K+]o helps explain why the action potential duration (APD) is shorter at higher [K+]o and longer at low [K+]o concentrations (see Table 61-1).6 As important as the time factor may be on the electrophysiological impact of different potassium levels, it is equally important to note that rapid fluctuations in extracellular potassium levels do occur, especially through transcellular shifts (Table 61-2). Insulin, β-adrenergic agonists, aldosterone, and changes in blood pH may independently affect serum potassium levels.6
Table 61-1 Modulation of Potassium Currents by Electrolyte Concentration
IK1, Inward rectifier | Its conductance is proportional to the square root of [K+]o. The instantaneous inward rectification on depolarization is caused by the Mg2+ block at physiological [Mg2+]i.* |
IKr, Delayed rectifier | Low [K+]o decreases the delayed rectifier current (IKr). |
Ito, Transient outward | One type is voltage activated and modulated by neurotransmitters. The other type is activated by intracellular calcium. |
IK(Ca) | Opens in the presence of high levels of intracellular calcium. |
IK(Na) | Opens in the presence of high levels of intracellular sodium. |
* From Ishihara K, Mitsuiye T, Noma A, Takano M: The Mg2+ block and intrinsic gating underlying inward rectification of the K+ current in guinea-pig cardiac myocytes, J Physiol (Lond) 419:297–320, 1989.
Table 61-2 Factors That Affect the Transcellular Shift of Potassium
FROM INSIDE TO OUTSIDE | FROM OUTSIDE TO INSIDE |
---|---|
Acidosis | Alkalosis |
β-Adrenergic receptor stimulation | β2-Adrenergic receptor stimulation |
Digitalis | Insulin |
Solvent drag |
Hypokalemia
Hypokalemia is the most common electrolyte abnormality encountered in clinical practice. Potassium values of <3.6 mmol/L are seen in over 20% of hospitalized patients.8 As many as 10% to 40% of patients on thiazide diuretics and almost 50% of patients resuscitated from out-of-hospital ventricular fibrillation have low potassium levels. Hypokalemia results from decreased potassium intake, transcellular shift, and, most commonly, increased renal or extra-renal losses (Table 61-3).9,10
Table 61-3 Causes of Hypokalemia
Decreased intake | |
Potassium shift into the cell (see Table 61-2) | |
RENAL POTASSIUM LOSS | |
Increased mineralocorticoid effects | Increased flow to distal nephron |
Primary or secondary aldosteronism | Diuretics |
Ectopic adrenocorticotropic hormone–producing tumor or Cushing syndrome | Salt-losing nephropathy |
Hypomagnesemia | |
Bartter syndrome | Nonresorbable anion |
Licorice | Carbenicillin, penicillin |
Renovascular or malignant hypertension | Renal tubular acidosis (type I or II) |
Congenital abnormality of steroid metabolism | Congenital defect of distal nephron |
Renin-producing tumor | Liddle syndrome |
EXTRARENAL POTASSIUM LOSS | |
Vomiting, diarrhea, laxative abuse, villous adenoma, Zollinger-Ellison syndrome |
Electrophysiological Effects of Hypokalemia
Hypokalemia leads to a higher (more negative) RMP and, at least during electrical diastole, a decrease in membrane excitability as a result of widening of the RMP and the threshold potential (TP) difference. Low extracellular potassium decreases the delayed rectifier current (IKr), resulting in an increase in the APD and a delay in repolarization. It has been suggested that extracellular K+ ions are required to open the delayed rectifier channel.7
Most importantly, hypokalemia alters the configuration of the action potential (AP), with the duration of phase 2 first increasing and subsequently decreasing, whereas the slope of phase 3 decelerates. The latter effect leads to an AP with a long “tail,” resulting in an increase in the relative refractory period (RRP) and a decrease in the difference of the RMP from the TP during the terminal phase of the AP. Thus, cardiac tissue demonstrates increased excitability with associated ectopy for a considerable portion of the AP. Conduction slows because depolarization begins in incompletely repolarized fibers. Furthermore, hypokalemia prolongs the plateau in the Purkinje fibers but shortens it in the ventricular fibers.11 The AP tail of the conducting system prolongs more than that of the ventricles, increasing the dispersion of repolarization. Hypokalemia increases diastolic depolarization in Purkinje fibers, thereby increasing automaticity.
In summary, the electrophysiological effects of hypokalemia are (1) a decrease in conduction velocity; (2) shortening of the effective refractory period (ERP); (3) prolongation of the RRP; (4) increased automaticity; and (5) early after-depolarizations (EADs) (Box 61-1).
Electrocardiographic Manifestations of Hypokalemia
The electrocardiographic manifestations of hypokalemia can be conceptualized as those caused by its effects on repolarization and those emanating from its effects on conduction (Box 61-2).12 The electrocardiogram (ECG) changes resulting from its effects on repolarization include (1) decreased amplitude and broadening of the T waves; (2) prominent U waves; (3) ST-segment depression; and (4) T and U wave fusions, all of which is seen in severe hypokalemia (Figure 61-1). When the U wave exceeds the T wave in amplitude, the serum potassium is less than 3 mmol/L. Electrocardiographic changes caused by conduction abnormalities are seen in the more advanced stages of hypokalemia and include (1) increase in QRS duration without a concomitant change in the QRS configuration; (2) atrioventricular (AV) block; (3) cardiac arrest; (4) increase in P-wave amplitude and duration; and (5) slight prolongation of the P-R interval.
Arrhythmogenic Potential and Clinical Implications of Hypokalemia
Hypokalemia-induced hyperexcitability is clinically manifested by an increase in supraventricular and ventricular ectopy. In the Framingham Offspring Study, potassium and magnesium levels were inversely related to the occurrence of complex or frequent ventricular premature complexes (VPCs) after adjustment for covariates.13
Hypokalemia facilitates re-entry by slowing conduction during the prolonged RRP and by causing an increase in the dispersion of refractoriness. Its suppressant effect on the sodium-potassium (Na-K) pump leads to intracellular Ca2+ overload and this facilitates the development of delayed after-depolarizations (DADs) via a transient inward current (Iti). Hypokalemia enhances the propensity for ventricular fibrillation in the normal as well as the ischemic canine heart.14 An association between hypokalemia and ventricular fibrillation in patients with acute myocardial infarction has been well established.15–17
Dofetilide and quinidine were found to exert increased block of Ikr in the setting of low [K+]o providing a mechanism that explains the link between hypokalemia and torsades de pointes (Figures 61-2 and 61-3).
Hyperkalemia
Although less common than hypokalemia, hyperkalemia may affect approximately 8% of hospitalized patients in the United States. Hyperkalemia is seen mainly in the setting of compromised renal function, particularly in association with the administration of a variety of nephrotoxic medications. Hyperkalemia may result from either decreased excretion or a shift of potassium from within the cell (Box 61-3).
Electrophysiological Effects of Hyperkalemia
The disproportional effects of varying levels of hyperkalemia on the RMP and the TP explain the initial increase in excitability and conduction velocity and then their decrease as the potassium level increases further (Table 61-4). Mild-to-moderate levels of hyperkalemia decrease the RMP (less negative) more than the TP, thereby diminishing the difference between the two and increasing excitability. The decrease in the slope of the upstroke of the AP (dV/dT), one of the major determinants of conduction velocity, is counterbalanced by a decrease in the difference between the RMP and the TP, resulting in an ultimate increase in conduction velocity. Severe hyperkalemia is associated with an increase in the difference between the RMP and the TP, leading to a decrease in excitability. Further decrement in the AP upstroke overwhelms the positive effect of the TP decrease on conduction velocity, resulting in a definitive decrease of the latter.
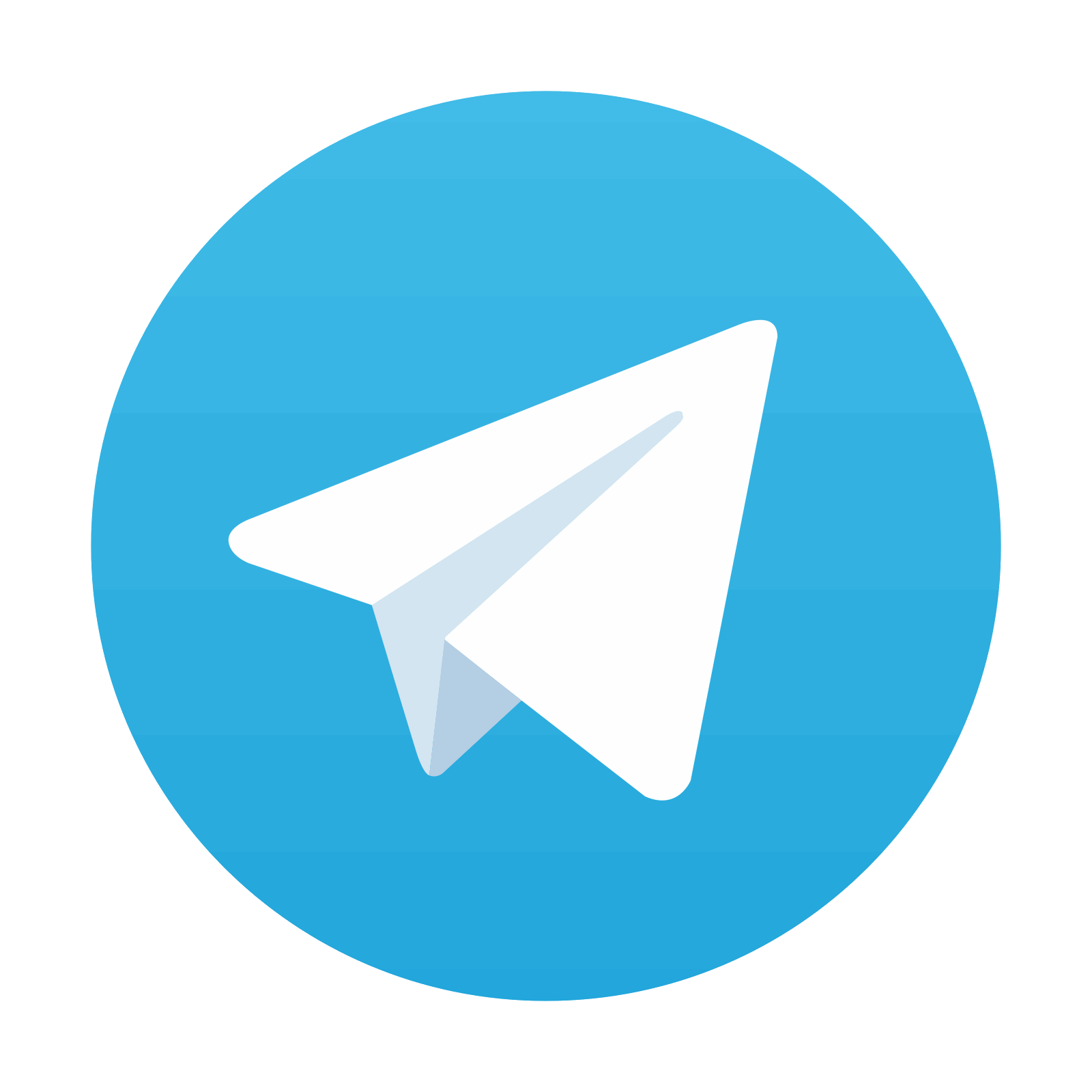
Stay updated, free articles. Join our Telegram channel

Full access? Get Clinical Tree
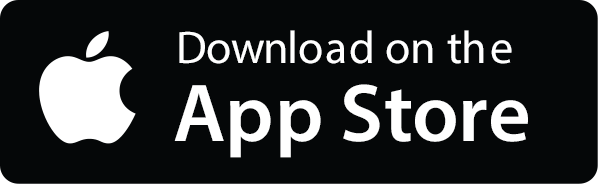
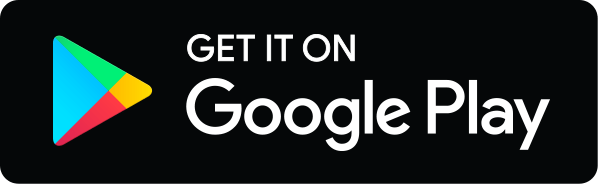