Chapter 79 Arrhythmias and Adult Congenital Heart Disease
Congenital heart defects complicate approximately 0.5% to 1% of all live births. More than 1 million adults in the United States are estimated to have repaired or unrepaired congenital heart disease.1 Approximately 50% of this group has a congenital heart defect categorized as moderate or severe by the American Heart Association/American College of Cardiology (AHA/ACC) task force on adult congenital heart disease (ACHD); this group of patients is at the greatest risk for cardiac arrhythmias.2 This number can only be expected to increase in the future.3 Advances in surgical technique now allow many patients with previously fatal congenital defects to survive into adulthood. However, the electrophysiological and physiological/hemodynamic consequences of these lesions often manifest as arrhythmias in young adults. In many patients, arrhythmias are a leading cause of functional decline as well as sudden cardiac arrest.4,5 A recent survey showed that hospital admissions for ACHD doubled between 1998 and 2005; the most common reason for hospital admission was a cardiac arrhythmia.6 Knowledge of the anatomy and physiology of congenital heart defects is critical to managing cardiac arrhythmias in this unique ACHD patient population.
Percutaneous catheter mapping and ablation of atrial and ventricular arrhythmias in the ACHD population have undergone significant advances in the past decade. In particular, the advent of electroanatomic three-dimensional mapping and its integration with cardiac imaging such as computed tomography (CT) or magnetic resonance imaging (MRI) have helped the electrophysiologist define the precise electroanatomic substrate for the arrhythmias that develop in this complex population.7 Percutaneous access to the cardiac chambers often presents unique challenges in this patient population, requiring detailed knowledge of the lesion and anatomic repair as well as familiarity with procedures such as trans-septal and trans-baffle puncture. In addition, ACHD patients present significant hemodynamic challenges because they have lower cardiac reserve and greater physiological instability in response to anesthesia or with the occurrence of rapid heart rates.
General Principles
It cannot be overemphasized that a thorough understanding of the individual anatomy and physiology of the patient with congenital heart disease is essential before planning and performing any invasive electrophysiological procedure. Prior operative and catheterization reports should be reviewed in detail. Noninvasive imaging such as echocardiography, MRA, or CTA should be reviewed with a physician experienced in interpreting studies of patients with ACHD.8 Patients must be viewed within the historical context of the type of cardiac repair they have received; different variations of surgical repairs for the same congenital heart defect may yield vastly different electrophysiological outcomes. Venous access may be altered by the surgical procedure or by prior catheterizations, and standard approaches to the chamber of interest may not be possible.9
Antiarrhythmic Drug Therapy
FDA class 1C agents (flecainide, propafenone) have been shown to be proarrhythmic in adults with ischemic heart disease. Nevertheless, they are often efficacious in the treatment for supraventricular and ventricular arrhythmias and are sometimes used in the pediatric patient with congenital heart disease because these agents are well tolerated and pose no risk of organ toxicity.10 These agents should be used with caution in the patient with ACHD; they may have a role in the presence of a backup implantable cardioverter-defibrillator (ICD).
A more recent addition to the antiarrhythmic armamentarium is dofetilide. Although studies have demonstrated safety in the adult population with prior myocardial infarction (MI), studies on its efficacy or toxicity in the ACHD patient population have not been done.11 Dofetilide is a potent potassium channel blocker and must be initiated in the inpatient setting, where monitoring of the QTc interval can occur. Unlike sotalol, dofetilide has no β-blocking properties and therefore is less likely to cause significant bradycardia or AV conduction block. However, concomitant β-blocking agents typically are required to slow the ventricular rate in response to atrial arrhythmias. Verapamil is contraindicated in patients taking dofetilide because of its interaction with hepatic metabolism; diltiazem, diuretics, and digoxin may also increase the risk of proarrhythmia and should be used with caution.
In patients with significant systemic ventricular dysfunction, amiodarone is the agent of choice. Amiodarone therapy often slows conduction velocity in an already diseased atrial substrate and may therefore facilitate 1 : 1 A : V conduction during atrial flutter or intra-atrial re-entrant tachycardia (IART) leading to hemodynamic compromise or silent ventricular dysfunction. This is uncommon because of the associated effect of slowing AV nodal conduction. The known risk of hepatic dysfunction with amiodarone may be worsened in the ACHD population because chronic hepatic venous congestion is common. In addition, patients should be monitored for pulmonary, thyroid, and ocular toxicities. Because of its long-term toxicities, amiodarone is typically reserved for patients with recurrent, poorly tolerated arrhythmias not amenable to catheter ablative therapy or for those with significant coexistent ventricular dysfunction. Typically, the lowest dose of amiodarone effective in suppressing arrhythmias—often 100 mg daily—is sufficient. Amiodarone can be safely initiated in the outpatient setting, typically at 400 mg daily and then titrated downward; however, in patients at risk for significant bradycardia, inpatient monitoring is advised. Dronedarone—a new antiarrhythmic agent with similar structure to amiodarone, but without amiodarone’s iodine moiety or long-term organ toxicity—has recently been approved for use in atrial arrhythmias and may provide another option for the patient with ACHD.12
Invasive Electrophysiology Study
The advent of three-dimensional electroanatomic mapping techniques allows realistic depiction of the patient’s anatomy combined with three-dimensional visualization of the tachycardia circuit. Electroanatomic mapping systems use magnetic fields or impedance measurements to locate a catheter in space. By moving the catheter around the chamber of interest, a shell of the chamber can be created. At each point on the shell, both the local recorded bipolar voltage and the activation time during a tachycardia can be annotated and color coded. The voltage map, in combination with pace mapping, can be quite useful for denoting areas of scar (low voltage) or prosthetic baffle material (no voltage) when developing an ablation strategy.13 Activation mapping denotes the local activation time relative to another fixed reference catheter. In the general adult patient with atrial arrhythmias, the coronary sinus (CS) catheter is used as the reference. In the ACHD population, the CS may not be accessible and any stable catheter position such as the atrial appendage can be used. The use of active fixation leads for this purpose in complex cases has been described.14 The combined voltage and activation maps, coupled with standard entrainment mapping, can be used to tailor an ablation strategy. These advanced technologies have improved the outcomes of catheter ablation but do not substitute a thorough understanding of the anatomy and electrophysiology associated with the arrhythmia occurring in these patients. Integration with CTA or MRA angiographic imaging can be performed to obtain a more realistic depiction of the complex anatomy of the patient with ACHD (Figure 79-1). In our experience, the main usefulness of CTA image integration is to confirm that the entirety of a particular cardiac chamber has been mapped.
As described above, re-entry around anatomic obstacles such as surgical incisions or patch material is the dominant mechanism of both atrial and ventricular arrhythmias in patients with ACHD. A typical example is seen in repaired tetralogy of Fallot (TOF), in which patch material is often used to close the ventricular septal defect (VSD) and may be needed to expand the right ventricular outflow tract (Figure 79-2). During ablation, linear lesions can be created between low-voltage regions or anatomic barriers to transect a critical isthmus of the tachycardia circuit and interrupt the tachycardia. Creation of long linear lesions in scarred atrial tissue often is difficult; irrigated catheters have improved the outcome and should be considered when performing an ablation in this patient population. Whenever possible, proof of block across a linear lesion should be obtained with pacing maneuvers to minimize the likelihood of slow conduction across the line leading to a recurrent tachycardia. Less frequently, atrial stretch can lead to “focal” atrial tachycardia. This tachycardia can be focally ablated at the site of earliest activation, often identified by low-amplitude fractionated electrograms.
The remainder of this chapter is dedicated to a discussion of the most common groups of ACHD lesions seen in patients with arrhythmias: atrial septal defects (ASDs), Fontan procedures, transposition of the great arteries after an intra-atrial repair, and TOF. These repairs represent the majority of patients seen in the ACHD arrhythmia clinic. Although less common, Ebstein’s anomaly also is discussed given its unique electrophysiological consequences. The common strategies applied to the arrhythmias encountered with these lesions can often be extrapolated to other patient groups not discussed here, including those with scar-related arrhythmias after VSD repair or AV canal repair. The reader is directed to the recently published guidelines for the management of ACHD as well as for device therapy.2,15
Atrial Septal Defects
ASDs are among the most common congenital heart lesions and often are associated with atrial arrhythmias, both preoperatively and after repair.16 The majority of ASDs are of the secundum type (75%). Secundum ASDs occur within the oval fossa, although they may extend outside the fossa when an associated deficiency of the atrial septum exists. The electrocardiogram (ECG) in patients with secundum ASDs typically shows evidence of a vertically oriented P wave, right-axis deviation of the QRS complex, and incomplete right bundle branch block (RBBB). Primum ASDs, which are a type of endocardial cushion defect, occur less commonly (15% to 20%) than secundum ASDs and often are associated with mitral or tricuspid valve regurgitation. The ECG in a patient with a primum ASD typically has left-axis deviation of the QRS complex. Primum ASDs can be associated with AV conduction disturbances and, occasionally, with complete heart block. Sinus venosus ASDs (5% to 10%) occur in either the superior or inferior paraseptal region, at the mouth of the IVC or the SVC. Superior sinus venosus ASDs may be associated with sinus node disease and typically have left-axis deviation of the P wave on the ECG. Least common is the coronary sinus septal defect, a defect between the wall of the coronary sinus and the left atrium. All the above ASDs lead to left atrial enlargement, fibrosis, and changes in atrial refractoriness.17 These changes in response to the chronic volume overload often lead to the development of both AF and atrial flutter.
Of the patients referred for the surgical closure of an ASD, 10% to 20% would have had at least one episode of AF or atrial flutter.15 Risk factors for atrial arrhythmia after surgical ASD closure include older age at the time of repair (>40 years), elevated pulmonary arterial pressure, preoperative atrial arrhythmia, and postoperative junctional rhythm. Given the continued risk of arrhythmia, combining the Maze procedure with septal defect repair should be considered in those patients with a history of AF or atrial flutter. Whether prophylactic Maze surgery has a role in the treatment of those patients at highest risk of AF undergoing ASD repair remains controversial. Incisional atrial flutters after ASD repair typically occur around the posterior right atriotomy incision rather than around the ASD patch itself. Catheter ablation can be extremely effective. The long-term occurrence of atrial arrhythmias after transvenous ASD closure is unknown, but one promise of this technology is a potential reduction in incisional re-entrant tachycardias.
Ebstein’s Anomaly of the Tricuspid Valve
A wide range of severity in hemodynamic abnormalities and arrhythmia occurrence exists in patients with Ebstein’s anomaly of the tricuspid valve. The structural abnormality involves the apical displacement of the septal leaflet of the tricuspid valve, often with displacement of the mural (posterior) leaflet and atrialization of the basal portion of the right ventricle. The valve itself is malformed and regurgitant, and an ASD or patent foramen ovale, often with right-to-left shunting, is present in one third of patients.19 This combination leads to severe right atrial dilation and a susceptibility to atrial arrhythmias. Ebstein’s anomaly can also be seen in congenitally corrected transposition of the great arteries (L-TGA).
Ebstein’s anomaly is commonly associated with Wolff-Parkinson-White (WPW) syndrome, with a reported prevalence of 10% to 40%.20–22 Accessory pathways in patients with Ebstein’s anomaly are typically right sided, and multiple accessory pathways are often present (30% to 50%). In addition to classic AV pathways, variants such as slowly conducting atriofascicular fibers are also more common in patients with Ebstein’s anomaly.23 In this population, the clinician should take care not to overlook subtle pre-excitation, which may manifest as absence of the expected RBBB.24 Because Ebstein’s anomaly may be clinically silent into adulthood, echocardiography should be performed in any adult with right-sided accessory pathways and evidence of right atrial enlargement on the ECG to exclude this abnormality.
As with many other congenital lesions associated with right atrial enlargement, atrial flutter and AF are commonly seen in adults with Ebstein’s anomaly.25 The atrial arrhythmia burden remains high even after surgical repair, with at least one third of patients having AF observed in long-term follow-up.26
Ablation of supraventricular tachycardia (SVT) related to accessory pathways has become standard therapy for these patients. If surgical repair is planned, an electrophysiology study (EPS) should be performed before the operation in any patient with known or suspected pre-excitation. The absence of a RBBB pattern in lead V1 has been shown to be predictive of an occult accessory pathway in this group.27 Catheter ablation should be attempted before surgery, with intraoperative mapping performed if catheter ablation cannot be performed or has been unsuccessful.
Catheter ablation of the accessory pathways can be highly successful, although it remains a challenging procedure. Overall acute success rates for catheter ablation of accessory pathways are lower (80%), and recurrence rates are higher than in patients who do not have Ebstein’s anomaly. This is most likely caused by the presence of multiple pathways and catheter instability along the tricuspid annulus (which is displaced from the valve leaflets themselves) and because of the presence of tricuspid regurgitation.28 Use of long sheaths and a multipolar halo catheter along the tricuspid annulus may be helpful to guide the ablation and improve catheter stability. In addition, the use of a microcatheter placed within the right coronary artery may help with pathway localization when traditional endocardial catheters such as the multipolar halo catheter are unhelpful.29 As with patients who do not have Ebstein’s anomaly, coronary artery occlusion remains a risk of ablation in this region.30
Because the right ventricle is the primary site of abnormality in Ebstein’s anomaly, it is not surprising that ventricular tachycardia (VT) has been described in Ebstein’s anomaly.31,32 VT can arise from within the functional right ventricle itself or from within the atrialized portion of the right ventricle, which retains ventricular electrophysiological properties. As with atrial arrhythmias, the occurrence of VT in a patient with unrepaired Ebstein’s anomaly who has significant tricuspid regurgitation should initiate consideration of surgical repair. Because repair of the tricuspid valve may not always be feasible, we recommend an EPS and attempted ablation of VT before surgery. VT is typically focal and located in the basal atrialized portion of the right ventricle (Figure 79-3). Catheter ablation can be helpful in these patients and, if unsuccessful, can guide surgical cryoablation at the time of valve repair or replacement. This is particularly important if mechanical tricuspid valve replacement is required because future percutaneous access to the right ventricle will be eliminated.
Fontan Operation
Originally performed for the palliation of tricuspid atresia, the Fontan operation is applied to numerous congenital heart lesions when single-ventricle physiology exists. The classic Fontan operation involved the creation of a direct connection from the right atrium to the pulmonary artery, completely bypassing the right ventricle, leading to passive filling of the pulmonary arterial tree and elevated right atrial filling pressures. The occurrence of atrial arrhythmias over time is the major complication of the subsequent right atrial volume overload. In addition to AF and typical atrial flutter, incisional or IART may be seen.18
The Fontan operation has undergone numerous modifications in an effort to reduce perioperative mortality rates and to improve hemodynamic and electrophysiological outcomes. The most recent modification is the extracardiac cavopulmonary Fontan operation, which creates a conduit between the IVC and the SVC, which is then anastomosed to the pulmonary artery, completely bypassing the right atrium and the right ventricle. The lateral tunnel Fontan operation and the extracardiac Fontan operation have largely replaced the atriopulmonary connection in patients with a single ventricle and have resulted in improvements in hemodynamic and arrhythmia outcomes.33
The onset of atrial arrhythmias in patients with atriopulmonary Fontan repairs often prompts evaluation for conversion to a cavopulmonary Fontan repair. The risks of a repeat operation need to be balanced against the benefit of Fontan conversion and individualized for each patient. In experienced centers, conversion to a total cavopulmonary Fontan repair in patients with preserved left ventricular function has been associated with reduced long-term morbidity and mortality rates. Concomitant arrhythmia surgery is recommended with a modified right-sided Maze procedure in patients with IART or atrial flutter.34 Some have recommended the Maze as a prophylactic procedure in those undergoing Fontan conversion regardless of prior arrhythmias. A standard lesion set involves cryoablation lesions applied at the time of Fontan conversion connecting the superior atrial septum to the right atrial appendage, connecting the posterior atrial septum to the incised posterolateral atrial wall transecting the crista terminalis, and a cavotricuspid isthmus ablation.35,36 Lesion sets may need to be modified depending on the underlying anatomic substrate. Despite these remarkable technical advancements, atrial arrhythmias remain a common problem in this population.
Nearly half the adult patients with congenital heart disease who have undergone a Fontan operation will be seen to have atrial arrhythmias in follow-up.37 These arrhythmias include AF, atrial flutter, IART, and SVT. The presence of AV valvular regurgitation is associated with atrial tachyarrhythmias and the development of Fontan obstruction; therefore a hemodynamic evaluation often is warranted in any patient with a new atrial arrhythmia after a Fontan repair. Given the lack of active transport within the right atrium and the presence of prosthetic material in these patients, atrial arrhythmias should be anticoagulated with warfarin.5 Once diagnosed, atrial arrhythmias tend to recur in these patients. Concomitant sinus node dysfunction as well as AV conduction disease can complicate medical therapy for tachyarrhythmias, making a percutaneous ablative approach very appealing in patients who have not undergone surgery.
Intra-atrial Re-entrant Tachycardia
Although typical isthmus-dependent right atrial flutter revolving around the tricuspid valve is commonly seen in patients who have had the Fontan operation, multiple re-entrant and non–re-entrant circuits are often present.38 IART seen in patients who have had the Fontan operation is often caused by a combination of the atrial barriers created at the time of surgery and progressive atrial scarring. Risk factors for IART include older age at the time of surgery, sinus node dysfunction, and abnormal right atrial and ventricular hemodynamics.37 The combination of atrial enlargement, atrial scarring with resultant slow conduction, and the use of antiarrhythmic drug therapy can often lead to slow IARTs with the potential for 1 : 1 AV conduction or increased heart rates that can cause significant hemodynamic deterioration, especially in poorly functioning single or systemic right ventricles.
Antiarrhythmic therapy for IART has been disappointing, even when potent antiarrhythmic agents such as amiodarone are used. Many centers now consider catheter ablation for IART as an early therapy depending on the underlying substrate. In patients who have had the Fontan operation, if percutaneous access to the right atrium can be obtained, catheter ablation for IART can be reasonably successful, with acute efficacy ranging from 60% to 80% but long-term recurrence rates of approximately 40%.39 Patients who have had the Fontan operation with a lateral tunnel may require a surgical approach; however, trans-baffle puncture into the native right atrium is an option for experienced surgeons. Catheter-based treatment of IART requires careful definition of the anatomic circuit. Locating scar material within the atrium usually allows the identification of a critical isthmus between the anatomic barriers and the creation of a linear ablation lesion between these barriers to interrupt the tachycardia circuit.40 The use of three-dimensional electroanatomic mapping along with entrainment is critical for defining the circuit and the anatomic barriers more completely.41–42
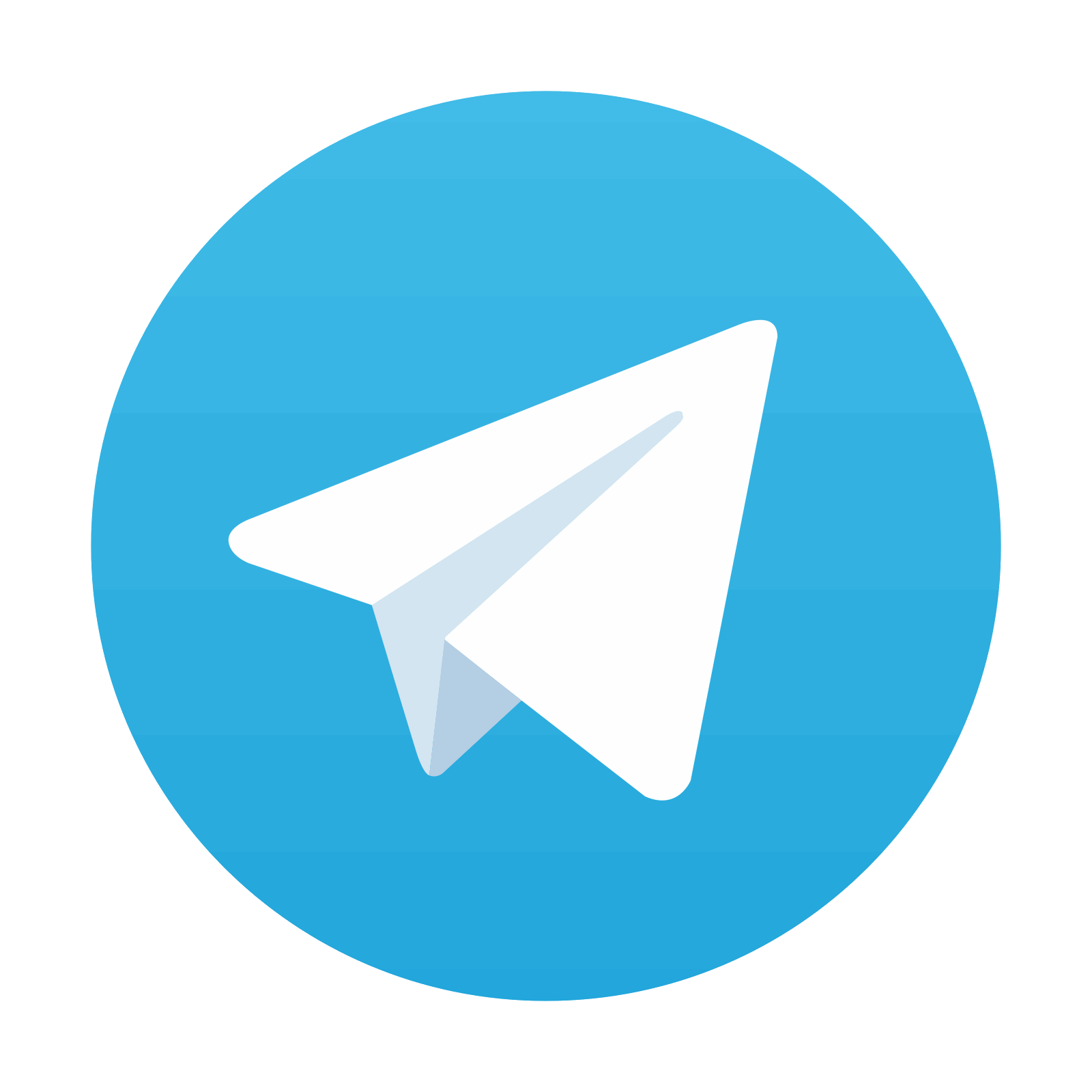
Stay updated, free articles. Join our Telegram channel

Full access? Get Clinical Tree
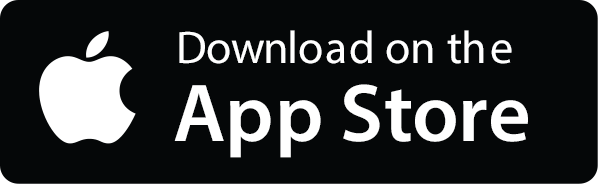
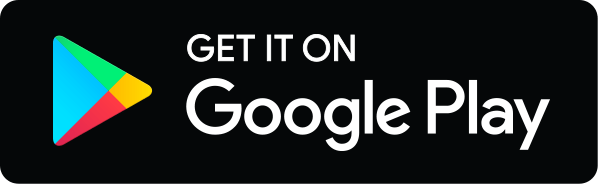