Key Points
- •
The complete axis of the RAAS along with intracellular RAAS components, alternative pathways, the kallikrein-kinin system, plasma renin and prorenin, and prorenin receptors play a significant role in cardiovascular and renovascular pathophysiology.
- •
The relationship between cardiac and renal disease is significant, with a strong but incomplete link between renoprotection and cardiovascular risk reduction.
- •
Proteinuria may be a potential cardiovascular risk factor and a target or indicator of therapeutic response.
- •
RAAS inhibitors are the primary classes of antihypertensive medications with both cardioprotective and renoprotective effects and are the cornerstone for prevention, particularly in high-risk patients.
- •
Combinations of different classes of RAAS inhibitors or RAAS inhibitors with calcium channel blockers provide novel ways for more complete RAAS suppression and improved end-organ protection.
Understanding of the role of the renin-angiotensin-aldosterone system (RAAS) in the genesis of cardiac and vascular disease and the close relationship between renal dysfunction and cardiovascular outcomes is increasingly important. Many classes of antihypertensive medications and their combinations have been shown to have beneficial effects, including cardioprotection and renoprotection, and are the cornerstone for primary and secondary prevention. This chapter details each class of antihypertensive medications and elaborates their roles in prevention ( Table 11-1 ).
Antihypertensive Drug Class | Examples | Mechanisms of Action | Physiologic Effects |
---|---|---|---|
Diuretic (thiazide type) | Chlorthalidone Hydrochlorothiazide | Inhibits sodium reabsorption via Na + /Cl − channels in the distal convoluted tubule of the kidney | ↓ Plasma volume ↓ Peripheral vascular resistance ↑ RAAS |
Nitrate | Nitroglycerin Isosorbide mononitrate Isosorbide dinitrate | Produces active metabolite nitric oxide | ↑ Vasodilation of coronary arteries, collateral vessels, veins ↓ Systemic arterial pressure ↓ Preload, myocardial wall stress, and MV o 2 ↑ Endothelial function |
Calcium channel blocker | Dihydropyridines: nifedipine, amlodipine, felodipine Nondihydropyridines: diltiazem, verapamil | Reduce excess Ca 2+ entry through voltage- and receptor-operated calcium channels in vascular smooth muscle and cardiac myocytes Improved nitric oxide production and release Increases t-PA activity Reduction in A II–mediated vasoconstriction and aldosterone production | ↑ Arteriolar vasodilation including coronary arteries ↓ Inotropy (nondihydropyridine) ↑ Endothelial function ↑ RAAS |
Beta blocker | Nonselective beta 1 and beta 2 antagonists: propranolol Selective beta 1 antagonists: atenolol, bisoprolol, metoprolol, nebivolol Alpha 1 , beta 1 , beta 2 antagonists: carvedilol, labetalol | Decrease in sympathetic activity through blockade of beta 1 , beta 2 , and alpha 1 receptors Lower plasma renin levels Reduction in endothelin and oxidative stress | ↓ In catecholamine levels ↓ Myocardial workload and oxygen demand ↓ Fatty acid metabolism ↓ Inotropy, transiently Altered sympathomimetic activity |
Angiotensin-converting enzyme (ACE) inhibitor | Benazepril, captopril, enalapril, fosinopril, lisinopril, perindopril, quinapril, ramipril, trandolapril | Inhibits ACE, reducing levels of A II and aldosterone Inhibits ACE-dependent metabolism of bradykinin and kallidin | Alters cardiac remodeling and vascular protective effects Modulation of adrenergic tone ↑ Bradykinin |
Angiotensin receptor blocker | Candesartan, irbesartan, losartan, olmesartan, telmisartan | Block the AT 1 receptor Compensatory rise in A II may activate AT 2 receptor | ↓ Vascular and cardiac hypertrophy ↓ Aldosterone secretion↓ Vasoconstriction |
Direct renin inhibitor | Aliskiren | Blocks renin, rate-limiting step of A II production Reduction in the formation of A I and A II Reduction in plasma renin activity | ↓ Atherosclerosis progression * ↓ aldosterone secretion ↓ Media degeneration * ↑ Nitric oxide availability * |
Mineralocorticoid receptor blocker | Eplerenone, spironolactone | Blocks aldosterone receptor | ↓ Plasma volume ↓ Cardiac hypertrophy |
Diuretics
Diuretics can be distinguished as loop or thiazide type. Loop-type diuretics have a greater ability to affect volume-overloaded states, and thiazide-type diuretics have a greater ability to reduce blood pressure. Their mechanisms of action differ. Loop diuretics inhibit the sodium-potassium-chloride (Na-K-Cl) transporter on the ascending limb of the loop of Henle. Thiazide diuretics primarily inhibit sodium reabsorption at the renal distal convoluted tubule, thereby reducing plasma volume and lowering blood pressure. Thiazide use also reduces peripheral vascular resistance and acutely stimulates the RAAS.
Because of the thiazide diuretic role in antihypertensive treatment and its associated outcomes data, thiazides are the main class of diuretics used for primary prevention. The most commonly used thiazide in clinical practice, hydrochlorothiazide, has a short half-life of 8 to 15 hours with long-term dosing. Another thiazide, chlorthalidone, has a longer half-life, 45 to 60 hours, and has been used in most clinical trials, establishing the utility of thiazides. Thiazides are particularly beneficial in controlling hypertension in certain populations of salt-sensitive patients, including African Americans, women, and the elderly.
Role of Diuretics
Early literature on diuretics did not show as great a reduction in cardiovascular events as had been predicted by epidemiologic data. Many believed that such a discrepancy was explained by the shorter duration of clinical trials compared with epidemiologic studies. Others postulated that the increased risk of cardiovascular effects was attributed to the many potential adverse, metabolic effects of diuretics, which include electrolyte abnormalities, hyperlipidemia, hyperglycemia, and impaired insulin sensitivity.
Diuretics can alter the lipid profile by increasing both serum low-density lipoprotein (LDL) and very-low-density lipoprotein (VLDL) cholesterol, without having a significant effect on high-density lipoprotein (HDL) cholesterol or the apoproteins. The mechanism of diuretic-induced dyslipidemia is unclear, and whether this occurs for all patients taking diuretics requires further investigation. Diuretics may also impair insulin sensitivity in both diabetic and nondiabetic patients, which alone or together with resulting compensatory hyperinsulinemia can promote dyslipidemia and atherogenesis. There may be an increased risk of new-onset diabetes or metabolic syndrome with the use of thiazides, especially at higher doses.
More recent clinical trials question the role that diuretics have in reducing insulin sensitivity. Systolic Hypertension in the Elderly Program (SHEP) and Hypertension in the Very Elderly Trial (HYVET), in which diuretics were used as initial therapy or in combination with other agents, showed a significant reduction in cardiovascular events with no significant change in lipid profiles and a minimal increase in blood glucose levels. A mean follow-up of 14.3 years of patients from the SHEP trial showed that diabetes that developed during the trial among subjects receiving placebo was associated with worsened cardiovascular outcomes. However, diabetes that developed among subjects during diuretic therapy did not significantly affect cardiovascular mortality.
The Antihypertensive and Lipid-Lowering Treatment to Prevent Heart Attack Trial (ALLHAT) randomized more than 33,000 high-risk hypertensive patients to a regimen of chlorthalidone, amlodipine, or lisinopril as first-line therapy. More than half of the patients enrolled had evidence of atherosclerotic heart disease, with one quarter having a history of myocardial infarction or stroke. No difference in cardiovascular outcomes or mortality was noted among the different agents. Although cholesterol levels and the prevalence of new-onset diabetes (11.6% versus 9.8% and 8.1%) were higher in the diuretic group up to a mean 4.9-year follow-up, these metabolic differences did not translate into increased cardiovascular events or mortality. Whether the follow-up period for ALLHAT was adequate to reveal the impact of dyslipidemia and new-onset diabetes on outcomes remains unclear.
The SHEP, HYVET, and ALLHAT trials were performed in older populations with an average age of 67 to 84 years. Data from these trials may not be applicable to younger patients with hypertension, particularly in association with comorbid conditions such as obesity, dyslipidemia, and abnormal glucose tolerance. In contrast to ALLHAT, the Second Australian National Blood Pressure (ANBP2) trial showed a significant improvement in cardiovascular outcomes in elderly hypertensive patients who were started with an angiotensin-converting enzyme (ACE) inhibitor compared with a thiazide diuretic. There were several differences between these trials, including differences in the antihypertensive agents used (enalapril instead of lisinopril and hydrochlorothiazide instead of chlorthalidone) and the population of patients studied (lower baseline risk factors). Nonetheless, thiazide diuretics appear highly effective in lowering blood pressure and reducing cardiovascular events ( Table 11-2 ).
Antihypertensive Drug Class | Renal Trials | Renal Effects |
---|---|---|
Diuretics (thiazide type) | ALLHAT (n = 33,357): chlorthalidone, amlodipine, or lisinopril versus doxazosin; diuretic with significantly increased GFR slope during 4 years compared with CCB (−0.018 versus −0.012 dL/mg per year) INSIGHT (n = 6321): nifedipine GITS versus hydrochlorothiazide + amiloride; significant decrease in GFR 2.3 mL/min SHEP (n = 4736): chlorthalidone ± atenolol; significant increase in creatinine 2.8 µmol/L | Unclear effect on proteinuria Potential worsening of renal function |
Nitrates | None | None |
Calcium channel blocker (CCB) | AASK (n = 1094): metoprolol, ramipril, or amlodipine; no significant difference in GFR slope; ACE inhibitor showed 38% improvement in composite GFR, ESRD, or death versus CCB ALLHAT (n = 33,357): chlorthalidone, amlodipine, or lisinopril versus doxazosin; CCB with significantly decreased GFR slope during 4 years compared with diuretic (−0.012 versus −0.018 dL/mg per year) IDNT (n = 1715): irbesartan or amlodipine versus placebo; no significant difference in renal outcomes between amlodipine and placebo | Unclear effect on proteinuria Potential preservation of renal function |
Beta blocker | AASK (n = 1094): metoprolol, ramipril, or amlodipine; no significant difference in GFR slope; ACE inhibitor showed 22% improvement in composite GFR, ESRD, or death versus beta blocker GEMINI (n = 1235): carvedilol versus metoprolol; 16.2% reduction in microalbuminuria and 41% reduction in progression to microalbuminuria | Reduction in proteinuria No evidence of preservation of renal function |
Angiotensin-converting enzyme (ACE) inhibitor | AASK (n = 1094): metoprolol, ramipril, or amlodipine; no significant difference in GFR slope; ACE inhibitor showed 38% improvement in composite GFR, ESRD, or death versus CCB ALLHAT (n = 33,357): chlorthalidone, amlodipine, or lisinopril versus doxazosin; ACE inhibitor showed no significant difference in the GFR slope during 4 years compared with diuretic (−0.019 versus −0.018 dL/mg per year) MICRO-HOPE (n = 3577): ramipril versus placebo; 24% reduction in overt nephropathy (>300 mg proteinuria), with and without baseline microalbuminuria REIN (n = 352): ramipril versus placebo; decline in GFR per month significantly lower with ACE inhibitor versus placebo (0.53 versus 0.88 mL/min), 55% reduction in risk of doubling of baseline creatinine or ESRD | Reduction in proteinuria Preservation of renal function |
Angiotensin receptor blocker (ARB) | IDNT (n = 1715): irbesartan or amlodipine versus placebo; 20% reduction in doubling of creatinine, ESRD, creatinine >6 mg/dL, mortality compared with placebo and 23% compared with CCB MARVAL (n = 332): valsartan versus amlodipine; urine albumin excretion was 56% of baseline with valsartan and 92% of baseline with amlodipine; 29.9% valsartan versus 14.5% amlodipine reverted to normoalbuminuria RENAAL (n = 1513): losartan versus placebo; reduction in time to doubling of creatinine, ESRD, death by 16%, incidence of doubling of serum creatinine by 25%, ESRD by 28%; no impact on mortality TRANSCEND (n = 5927): telmisartan versus placebo; no significant difference in composite dialysis or creatinine doubling or change in GFR | Reduction in proteinuria Preservation of renal function |
Combination | ||
ACE inhibitor/ARB | CALM (n = 199): candesartan, lisinopril, or combination; 50% reduction in urinary ACR with combination compared with candesartan 24% and lisinopril 39% ONTARGET (n = 8576): ramipril or telmisartan versus combination; increased ESRD, creatinine doubling, death in combination 14.5% compared with ACE inhibitor or ARB 13.5%; increase in urinary albumin excretion was 7% less in combination compared with ACE inhibitor VALERIA (n = 133): valsartan/lisinopril versus valsartan or lisinopril; 34% reduction in urine ACR in combination compared with lisinopril; 38% of combination group had normalization of microalbuminuria compared with 17% lisinopril | Reduction in proteinuria Unclear effect on renal function |
Direct renin inhibitor (DRI) + ARB | AVOID (n = 599): aliskiren/losartan versus losartan; 20% reduction in urinary ACR without significant reduction in blood pressure | Reduction in proteinuria Unclear effect on renal function |
ACE inhibitor or ARB/mineralocorticoid receptor blocker (MRB) | Epstein et al (n = 268): eplerenone/enalapril versus enalapril; reduction in urine albumin excretion by 48.4% versus 7.4% Mehdi et al (n = 81): spironolactone/lisinopril versus lisinopril/losartan; reduction in urine albumin excretion with ACE inhibitor/MRB of 34% compared with ACE inhibitor/ARB 16.8% | Reduction in proteinuria Unclear effect on renal function |
ACE inhibitor or ARB/CCB | ASCOT (n = 19,257): perindopril/amlodipine versus atenolol/bendroflumethiazide; 15% reduction in development of renal impairment Fogari et al (n = 453): fosinopril, amlodipine, or combination; 67% reduction of urine albumin excretion with combination versus fosinopril 46% or amlodipine 33% at 48 months | Reduction in proteinuria Possible effect on renal function |
Renal Effects
Aside from altered lipid profiles and glycemic control, the SHEP and International Nifedipine GITS (INSIGHT) studies showed a greater decline in renal function with diuretic therapy, assessed by a rise in serum creatinine. The proposed mechanisms of renal injury by diuretics are varied and include volume depletion leading to renal ischemia and stimulation of the RAAS, arteriolar vasoconstriction from endothelial dysfunction and increased oxidative stress, metabolic abnormalities leading to renal hypertrophy and fibrosis, and direct toxic effects on the distal tubular cells ( Table 11-3 ).
Antihypertensive Drug Class | PRC | PRA | Ang I | Ang II | Aldosterone |
---|---|---|---|---|---|
Diuretic (thiazide) | ↑ | ↑ | ↑ | ↑ | ↑ |
Beta blocker | ↓ ↓ | ↓ ↓ | ↓ ↓ | ↓ ↓ | ↓ ↓ |
Calcium channel blocker | ↑ | ↑ | ↑ | ↑ | ↑ |
Angiotensin-converting enzyme (ACE) inhibitor | ↑ ↑ ↑ | ↑ ↑ ↑ | ↑ ↑ ↑ | ↓ ↓ | ↓ |
Angiotensin receptor blocker (ARB) | ↑ ↑ ↑ | ↑ ↑ ↑ | ↑ ↑ ↑ | ↑ ↑ ↑ | ↓ ↓ |
ACE inhibitor/ARB | ↑ ↑ ↑ | ↑ ↑ ↑ | ↑ ↑ ↑ | = ↑ | ↓ |
Direct renin inhibitor (DRI) | ↓ ↓ | ↓ ↓ | ↓ ↓ | ↓ ↓ | ↓ |
DRI/ARB | ↓ | ↓ | ↓ | ↓ | ↓↓ |
Mineralocorticoid receptor blocker | ↑ ↑ | ↑ ↑ | ↑ ↑ | ↑ ↑ | ↑ ↑ |
Summary
Diuretics are an acceptable therapy for uncomplicated hypertension. The substitution of hydrochlorothiazide with chlorthalidone in clinical practice may be indicated because of its better tolerability (longer half-life). In younger hypertensive patients and in patients with comorbid conditions, especially diabetes or when the pathophysiology of hypertension may be driven by activation of the RAAS or sympathetic activation, agents other than diuretics or diuretic combinations may be preferred (see Table 11-1 ).
Nitrates
Nitrates are prodrugs; their active metabolite is nitric oxide, also known as endothelium-derived relaxing factor. Nitrates relax vascular smooth muscle and have vasodilatory effects in both the systemic arteries, including the coronary arteries and collateral vessels, and the veins. The predominant venodilatory effect of nitrates reduces ventricular preload, which in turn reduces myocardial wall stress and oxygen requirements. Higher doses of nitrates may also decrease systemic arterial pressure. Other beneficial effects include improved endothelial function and a reduction in platelet adhesion and aggregation.
Role of Nitrates
Rapid anginal improvement and the resolution of electrocardiographic signs of ischemia have promoted the use of nitrates for the treatment of angina. Trials comparing long-acting nitrates with both calcium channel blockers and beta blockers have shown no significant difference in antianginal efficacy. In the treatment of heart failure, the use of nitrates reduces secondary pulmonary hypertension and improves heart failure symptoms. There are few prognostic data on nitrate therapy in heart failure. The effect of vasodilator therapy on mortality in a chronic congestive heart failure (CHF) trial (V-HeFT I) showed that when oral nitrates are used with an arterial vasodilator, hydralazine, there is a 34% reduction in overall mortality compared with alpha blockade or placebo. The V-HeFT II trial compared the same combination of nitrate and hydralazine with enalapril in patients with symptomatic CHF. Enalapril showed a further 28% reduction in mortality over combination therapy.
For post–myocardial infarction patients in the fibrinolytic era, trials have failed to show a significant benefit of short-term, early nitrate therapy. In the Gruppo Italiano per lo Studio della Sopravvivenza nell’Infarto Miocardico trial (GISSI-3), administration of transdermal nitroglycerin for 6 weeks after acute myocardial infarction demonstrated a nonstatistically significant 6% risk reduction in overall mortality. In the Fourth International Study of Infarct Survival (ISIS-4) trial, administration of oral mononitrate for 1 month after acute myocardial infarction also did not demonstrate an impact on postinfarction mortality or combined endpoint of death and heart failure or extensive ventricular dysfunction. The duration of nitrate therapy and follow-up of these trials were relatively short, and the open-label use of nitrates was high in the placebo arms.
With contemporary percutaneous revascularization, one early trial evaluated the long-term nitrate treatment of post–myocardial infarction patients and suggested an increase in cardiac events. Nitrate rebound during nitrate-free intervals and tolerance have been suggested as mechanisms to blunt hemodynamic effects and to increase cardiovascular events. There is also the suggestion of reflex neurohormonal activation with an increase in plasma renin activity and circulating angiotensin II (A II) levels during nitrate therapy, which attenuates the vasodilator effect of nitroglycerin during prolonged treatment. Both the rebound phenomenon and tolerance may be suppressed by the use of ACE inhibitor or angiotensin receptor blocker (ARB) therapy. More recent retrospective studies using optimal medical therapy have not identified an increase in adverse events, but a more neutral effect, further questioning the utility of nitrates in secondary prevention (see Table 11-2 ).
Summary
Nitrates are effective antianginal agents used for the symptomatic therapy of all entities of myocardial ischemia. Nitrate therapy can be effective in patients with coronary vasospasm or heart failure caused by myocardial infarction. Their role in the treatment of hypertension is limited. In post–myocardial infarction patients without evidence of myocardial ischemia or heart failure, the role of nitrate therapy in secondary prevention is not well established (see Table 11-2 ).
Calcium Channel Blockers
Calcium channel blockers (CCBs) are classified into the dihydropyridines and the nondihydropyridines, which include phenylalkylamines and benzothiazepines. Examples of the dihydropyridines are nifedipine, felodipine, and amlodipine; examples of the nondihydropyridines are diltiazem and verapamil. Advanced generations of CCBs have modified release formulations, and specifically the newer generation dihydropyridines have higher vascular selectivity and less direct cardiac effects in comparison to short-acting nifedipine.
The CCBs act by counteracting excess calcium entry through the voltage- and receptor-operated calcium channels. CCBs cause potent arteriolar vasodilation by the reduction of calcium entry into the vascular smooth muscle. Within the cardiac myocytes, maintaining the cytosolic calcium balance and preserving ATP stores help maintain myocyte viability and delay ischemic damage. Other effects of CCBs include the protection of endothelial integrity and reduction of endothelial apoptosis, improved nitric oxide production and release, improved fibrinolytic function through an increase in tissue-type plasminogen activator (t-PA) activity, and importantly, reductions in A II–mediated vasoconstriction and decreased A II stimulatory effect on aldosterone production. Many of these effects have also been attributed to inhibitors of the RAAS.
Role of Calcium Channel Blockers
Chronic Stable Angina
CCBs dilate the coronary arteries and increase coronary blood flow. Coronary blood flow is one determinant among others, including heart rate, contractility, and arterial pressure, that affects myocardial oxygen consumption, and all can be variably affected by CCBs. For chronic stable angina, three trials have evaluated the long-term effects of CCBs. Angina Prognosis Study in Stockholm (APSIS) and Total Ischaemic Burden European Trial (TIBET) compared verapamil with metoprolol and nifedipine SR with atenolol, respectively, in patients with stable angina pectoris. Neither study found a difference in morbidity and mortality rates between CCBs and beta blockers. When long-acting nifedipine was added to optimal medical therapy including beta blockade, no difference was noted in mortality, with small reductions in the secondary endpoints of need for coronary revascularization and heart failure.
Post–Myocardial Infarction
The Secondary Prevention Reinfarction Israeli Nifedipine trials (SPRINT and SPRINT II) evaluated the effect of the dihydropyridine nifedipine on cardiovascular morbidity and mortality during weeks 2 to 3 for SPRINT and within the first week for SPRINT II after an acute myocardial infarction. No significant difference was noted in morbidity or mortality for late therapy; however, early institution of calcium channel blockade was associated with an increased early mortality (within the first 6 days).
The secondary Danish Verapamil Infarction Trial (DAVIT II) showed for the first time that a nondihydropyridine reduces the incidence of death and reinfarction by approximately 20% when it is started 1 week after acute myocardial infarction. A significant reduction in mortality (11.8% versus 7.7%) was noted only in patients without CHF. Interestingly, the order of magnitude of this benefit was similar to that achieved in numerous beta blocker trials. In the Multicenter Diltiazem Post-Infarction Trial (MDPIT), diltiazem nonsignificantly decreased cardiac mortality or nonfatal reinfarction by 11%. However, there was a significant increase in late CHF (21% versus 11%) and an increase in cardiac events (hazard ratio, 1.41) in patients with ejection fractions less than 40%. The diltiazem-associated rise in the frequency of CHF was progressively greater with worsening decrements of ejection fraction.
Congestive Heart Failure
As potent vasodilators, CCBs have been shown to improve hemodynamic responses in CHF patients with notable decreases in pulmonary capillary wedge pressure, systemic vascular resistance, and end-diastolic ventricular pressure. CCBs are also negative inotropic agents and have varying degrees of impairment in cardiac function. The differences in negative inotropy are due to differences in the binding affinity ratios of smooth to cardiac muscle, with dihydropyridines being more vascular selective. An early generation CCB, nifedipine, showed clinical effects not consistent with their hemodynamic benefit, with a significant increase in heart failure deterioration. This has also been noted with the use of diltiazem after myocardial infarction in patients with ventricular dysfunction (ejection fraction <40%). CHF patients who deteriorate after a CCB have been shown to have a worse prognosis, with a significant decrease in mortality at 1 year.
Later generations of CCBs, felodipine and amlodipine, were developed with predominant peripheral vasodilating effects, having minimal effect on the myocardium and with longer half-lives thought to decrease reflex neurohormonal activation in patients with heart failure. The Prospective Randomized Amlodipine Survival Evaluation trials (PRAISE-1 and PRAISE-2) evaluated the long-term use of amlodipine in advanced heart failure patients. Patients had New York Heart Association (NYHA) Class III or Class IV heart failure and ejection fractions less than 30% despite treatment with ACE inhibitor, diuretic, and digoxin. PRAISE-1 evaluated 1153 patients regardless of the cause of heart failure, and PRAISE-2 evaluated 1652 nonischemic cardiomyopathy patients. In both trials, amlodipine showed a neutral effect on mortality in patients with severe chronic heart failure.
A trial similar to PRAISE was performed with felodipine, Vasodilator–Heart Failure Trial (V-HeFT III trial). This study evaluated 450 patients with NYHA Class II or Class III heart failure and ejection fractions less than 45%. Exercise capacity was not statistically significant, and no differences were noted in mortality or rates of hospitalization (see Table 11-1 ).
Renal Effects
CCBs have pleiotropic effects that might contribute to renal protection against hypertension-induced damage. These effects include a reduction in mesangial inflammation and proliferation and the promotion of nephrotoxic free radical removal. At the renovascular level, CCBs preferentially dilate the glomerular afferent arteriole, with only modest action on the efferent arteriole. This preferential effect, potentially noted more predominantly with dihydropyridine CCBs, may cause glomerular hypertension, increasing capillary intraglomerular pressure that could be associated with progression of renal disease.
Given their diversity of renal effects and the potential class differences between CCBs on intraglomerular pressures, trials evaluating the effects of CCBs on nephropathy are equally divergent. The African American Study of Kidney Disease and Hypertension (AASK) showed a lesser degree of renal function preservation in African Americans with hypertensive nephrosclerosis treated with amlodipine than with the ACE inhibitor ramipril. Furthermore, in this population of patients, amlodipine showed a significant increase in proteinuria compared with both metoprolol and ramipril. The ALLHAT study, on the other hand, showed that amlodipine maintained glomerular filtration rate (GFR) better than therapy with a diuretic or ACE inhibitor. Overall, the use of CCBs in renal disease is safe without any evidence of further deterioration of renal function. CCBs may be better than diuretics and beta blockers, but they are not as effective as RAAS inhibitors in reducing proteinuria and preventing the progression of renal disease (see Table 11-2 ).
Summary
In patients with chronic stable angina, CCBs may be considered for adjunctive treatment but not as monotherapy unless beta blockers and RAAS inhibitors are not tolerated. Early administration of nifedipine in acute myocardial infarction, unless it is specifically indicated in vasospastic angina, is contraindicated. The nondihydropyridines, possibly because of their heart rate–lowering properties, are the only CCBs to show benefit in the post–myocardial infarction population. They also increase the risk for subsequent CHF in post–myocardial infarction patients with reduced ejection fractions and should probably be reserved for patients without heart failure who cannot tolerate a beta blocker or in whom a beta blocker is contraindicated. Amlodipine and felodipine, given their neutral effect on cardiac morbidity and mortality and ease of tolerance, may be considered in the management of hypertension or coronary artery disease (CAD) in patients with heart failure (see Table 11-1 ).
Beta Blockers
Beta blockade reduces myocardial workload and oxygen demand through a reduction in heart rate, blood pressure, and cardiac index. A decrease in sympathetic activity reduces fatty acid metabolism and reduces catecholamine levels, helping to redistribute flow to ischemic territories. In addition, beta blockers moderately lower plasma renin activity, even in patients receiving treatment with an ACE inhibitor, in whom the biologic effects of renin are already attenuated.
Beta blockers differ in the degree to which they antagonize the effects of the sympathetic nervous system. The predominant effect of this class of medications is through interaction with the beta 1 receptor, primarily located within cardiac muscle. However, each drug has varying effects on beta 2 receptor antagonism regulating norepinephrine release, intrinsic sympathomimetic activity (which provides a measurable beta agonist response), and other adrenergic receptors such as the alpha 1 receptor. Nonselective beta antagonists block beta 1 and beta 2 receptors; an example is propranolol. Selective beta antagonists block beta 1 receptors; examples include atenolol metoprolol, bisoprolol, and nebivolol. Carvedilol and nebivolol block three adrenergic receptors (alpha 1 , beta 1 , and beta 2 ) and reduce endothelin and oxidative stress.
Role of Beta Blockers
For decades, beta blockers have been widely used in the treatment of uncomplicated hypertension. However, no trial has shown that lowering of blood pressure with a beta blocker reduces the risk of cardiovascular events in patients with hypertension compared with placebo. More recently, beta blocker treatment has been associated with a substantially higher (16%) risk of stroke than treatment with other antihypertensive agents. A proposed mechanism may be differences in the hemodynamic effects of beta blockade.
Treatment with a beta blocker results in reduced brachial blood pressure but does not lower central aortic blood pressure as much as treatment with an ACE inhibitor, diuretic, or CCB does. Central aortic blood pressure may be more predictive of cardiovascular events than traditional brachial blood pressure measurements. The outcome of both the Anglo-Scandinavian Cardiac Outcomes Trial–Blood Pressure Lowering Arm (ASCOT-BPLA) and the Losartan Intervention For Endpoint reduction in hypertension study (LIFE) showed a stroke reduction of approximately 25% when treatment with either a CCB or an ARB, respectively, was given instead of a beta blocker.
Post–Myocardial Infarction and Chronic Stable Angina
Early beta blockade relieves chest pain and reduces infarct size and fatal arrhythmias; long-term beta blockade reduces late mortality and reinfarction. Early trials and meta-analyses performed before reperfusion therapy suggested that beta blockade after myocardial infarction can reduce mortality, with the most marked reduction of about 25% occurring in the first 2 days after infarction. In the Cooperative Cardiovascular Project, beta blockade showed a mortality reduction of 40% across all patient subgroups, including high-risk groups such as the elderly and patients with a severely reduced ejection fraction.
The two largest pre–thrombolytic era trials examining beta blockade in acute myocardial infarction were the Metoprolol in Acute Myocardial Infarction (MIAMI) and the First International Study of Infarct Survival (ISIS-1) trials. In the MIAMI trial, more than 5000 post–myocardial infarction patients received metoprolol within 24 hours of presentation. Mortality was 4.9% in the placebo group and 4.3% in the metoprolol group. Despite the overall nonsignificant reduction in mortality, subgroup analysis revealed a survival advantage of nearly 30% in patients considered at higher risk. ISIS-1 trial evaluated the efficacy of early atenolol in nearly 16,000 patients presenting with myocardial infarction within 12 hours of symptoms. This trial showed a 15% reduction in vascular deaths as well as a 15% reduction in early cardiac arrests and reinfarctions, predominantly noted within the first day after infarction.
This benefit of beta blockade was evident in early administration and short-term continuation, before the standard use of fibrinolytic and antiplatelet therapy. It can be attributed to the prevention of life-threatening arrhythmias and cardiac rupture. The ClOpidogrel and Metoprolol in Myocardial Infarction Trial (COMMIT) is the largest trial to date, including nearly 46,000 patients to evaluate the effects of intravenous and then oral metoprolol in acute myocardial infarction added to current antiplatelet and fibrinolytic therapy. There was no significant difference noted in the rate of composite endpoint of death, reinfarction, or cardiac arrest, with overall mortality balanced by a 22% reduction in late arrhythmic death and a 29% proportional increase in early shock-related death. The overall net effect of metoprolol therapy changed from being significantly adverse during the first 2 days after admission due to shock-related death to being significantly beneficial thereafter due to a reduction in ventricular fibrillation.
In combining a retrospectively defined low-risk subgroup of COMMIT similar to the populations studied in MIAMI and ISIS-1, the overall effects were consistent, showing a significant reduction of 13% in mortality, 22% in reinfarction, or 15% in ventricular fibrillation. Beta blocker therapy for these trials among others, including the Beta Heart Attack Trial (BHAT) and the TIMI IIB trial, was continued up to 2 years. Furthermore, randomized trials of beta blocker therapy in patients undergoing percutaneous coronary intervention without fibrinolytic therapy have not been performed. It does seem reasonable, however, to extrapolate the results from those receiving another form of revascularization to the percutaneous coronary intervention population.
The use of beta blockade for the treatment of chronic stable angina has also been predominantly extrapolated from the evidence of improved mortality of beta blockers in the post–myocardial infarction patient. Given the lack of mortality benefit and increased risk of stroke in patients with uncomplicated hypertension, treatment of patients with chronic stable angina but no prior myocardial infarction with beta blockers is not well supported but commonly used. Other antihypertensive medications, such as CCBs and ACE inhibitors, may be equally beneficial without the potential adverse effects of beta blockade therapy.
Congestive Heart Failure
Because of transient negative inotropic effects of beta blockade, beta blockers used to be contraindicated in CHF. Many trials have since shown that patients with heart failure derive greater benefit from beta blocker therapy after myocardial infarction than do patients without heart failure. In the BHAT trial, propranolol reduced mortality equally in patients with and without systolic dysfunction by approximately 25%. However, there was a 47% reduction in sudden death in patients with heart failure, compared with 13% reduction in patients without heart failure.
The Metoprolol CR/XL Randomized Intervention Trial in Congestive Heart Failure (MERIT-HF), Cardiac Insufficiency Bisoprolol Study II (CIBIS II), and Carvedilol on Survival in Severe Chronic Heart Failure (COPERNICUS) trials evaluated the effects of beta blockade in patients with heart failure, with severely reduced ejection fractions, and receiving stable ACE inhibitor and diuretic therapy. Metoprolol, bisoprolol, and carvedilol, respectively, showed an overall reduction in total mortality of approximately 35%. In addition to improved mortality, beta blockers, when given with ACE inhibitors, improve the patient symptoms and NYHA functional class and reduce heart failure hospitalizations (see Table 11-4 ).
Compelling Indication | Therapeutic Options |
---|---|
High CAD risk | Thiazide * , beta blocker, CCB * , ACE inhibitor * , ACE inhibitor/CCB, ARB/CCB |
Chronic stable angina | Nitrates, CCB, ACE inhibitor, ACE inhibitor/CCB, ARB/CCB |
Post–myocardial infarction | Beta blocker * , ACE inhibitor * , ARB, MRB * , nondihydropyridine CCB |
Heart failure | Beta blocker * , ACE inhibitor * , ARB * , MRB * , diuretics, nitrates |
Chronic kidney disease | |
Reduction in proteinuria | Beta blocker, ACE inhibitor * , ARB * , DRI, ACE inhibitor/ARB, ACE inhibitor/MRB, ARB/MRB, ACE inhibitor/CCB, ARB/CCB |
Preservation of renal function | CCB, ACE inhibitor * , ARB * , ACE inhibitor/CCB, ARB/CCB |
* Classes recommended by the Seventh Report of the Joint National Committee (JNC7).
Renal Effects
Beta 1 receptors alter the release of renin, and beta 2 and alpha 1 receptors alter sympathetic activity responsible for renovascular tone, important in the genesis of hypertension and progression of kidney disease. Although beta blockers improve cardiac mortality, they have not shown the same benefit on renal outcomes compared with other agents that lower blood pressure and albuminuria. Studies in both diabetic and nondiabetic nephropathy do not show a significant reduction in microalbuminuria with beta blockade compared with ACE inhibition.
In the Glycemic Effects in Diabetes Mellitus: Carvedilol-Metoprolol Comparison in Hypertensives (GEMINI) trial, 1235 hypertensive, diabetic patients were enrolled to evaluate the effects of metoprolol versus carvedilol on glycemic control, with a secondary endpoint of change in microalbuminuria. There was a 16.2% reduction in microalbuminuria for patients randomized to carvedilol compared with metoprolol despite similar blood pressure control during 3 years. Among patients with normal albuminuria at baseline, fewer in the carvedilol group (6.6%) than in the metoprolol group (11.1%) progressed to microalbuminuria. GEMINI suggests that beta blockers with alpha 1 receptor activity may reduce the compensatory stimulation of the sympathetic system and RAAS caused by beta blockade and may further reduce the progression of albuminuria, providing cardiorenal protection (see Table 11-2 ).
Summary
Beta blockers have an established benefit for the treatment of patients after myocardial infarction or with heart failure and play an important role in secondary prevention. The role of beta blockade in the treatment of uncomplicated hypertension remains unclear, given the paucity of data and the possible mild increased risk of stroke. Delay of the initiation of beta blocker therapy until after stabilization of the patient from an acute myocardial infarction may avoid the excess risk of shock and early mortality while preserving much of its described benefits. Controversy remains as to the duration of beta blocker therapy in post–myocardial infarction patients, but if the medication remains well tolerated, discontinuation should be discouraged (see Table 11-4 ).
Renin-Angiotensin-Aldosterone System
Renin is a protease produced by the juxtaglomerular cells of the kidney that line the afferent arteriole of the glomerulus. Renin is secreted principally as a result of four mechanisms: renal baroreceptors in the afferent arteriole that sense alterations in renal perfusion pressure, a change in NaCl delivery to the macula densa cells of the distal tubule, sympathetic stimulation through beta 1 -adrenergic receptors, and negative feedback by A II on juxtaglomerular cells. Outside of the kidney, renin is secreted by other tissues including the brain, adrenal gland, ovary, and adipose tissue in addition to the heart and vascular tissue. Renin is the rate-limiting step of the RAAS ( Fig. 11-1 ).

Renin cleaves angiotensinogen to angiotensin I (A I), commonly referred to as Ang-(1-10) for its decapeptide structure. Angiotensinogen is primarily produced by the liver, but expression has been noted in tissue similar to that expressing renin, including the kidney and heart. A I is biologically inactive and is converted by ACE by the removal of a dipeptide to form A II or Ang-(1-8). ACE is a membrane-bound exopeptidase predominantly localized on the membranes of the vascular endothelial and renal proximal tubular cells. ACE also metabolizes other vasodilator peptides, including bradykinin and kallidin, which may have important physiologic effects that will be described later in the chapter.
Although A II is the primary active product in the RAAS, other metabolites of A I and A II may have physiologic activity. These include angiotensin III, (Ang-[2-8]); angiotensin IV, (Ang-[3-8]); and Ang-(1-7). All metabolites are formed by removal of varying amounts of amino acids from either the N or C terminus of A II and have varying effects, some of which are unknown to date.
It is the interaction of A II with the angiotensin receptors that drives many of the RAAS effects. At least four angiotensin receptors have been described; the AT 1 receptor mediates many of the pathophysiologic effects and therefore is a major therapeutic target for secondary prevention. AT 1 receptors are expressed in the kidney, adrenals, liver, brain, vasculature, and heart, and their activity includes vasoconstriction, vascular and cardiac hypertrophy, renal tubular sodium resorption, inhibition of renin release, sympathetic activation, cell growth and proliferation, and increased inflammatory response. AT 2 receptors are not highly expressed in the adult and may oppose some of the AT 1 receptor–mediated pathways. Some physiologic effects of AT 2 receptors may include vasodilation, smooth muscle antiproliferation, and inhibition of cardiac remodeling. AT 3 receptor function is unknown, and AT 4 receptor function has been shown to modulate endothelial function through plasminogen activator inhibitor 1 (PAI-1).
A II also stimulates, through the AT 1 receptor, the release of aldosterone, predominantly from the adrenal cortex, but extra-adrenal sites of aldosterone synthesis have been identified in the brain, vasculature, and heart. Aldosterone is regulated by both A II and dietary potassium. It is an important hormone in sodium and fluid retention as a result of direct stimulation of the mineralocorticoid receptors in the distal renal tubules. Mineralocorticoid receptors are also as widespread as their extra-adrenal sites. Their varied proposed physiologic mechanisms include renal, vascular, and cardiac inflammation; fibrosis and hypertrophy; increases in sympathetic activation; attenuation of platelet aggregation; and impairment of endothelial dysfunction ( Fig. 11-2 ).

Intracellular Renin-Angiotensin-Aldosterone System
Despite the multiorgan involvement, specifically in the kidney, vasculature, and heart, for the full physiologic axis of the RAAS, local tissue or intracellular RAAS has been identified and is believed to play a significant role in cardiovascular pathophysiology. The intracellular RAAS is defined by a functionally active complete RAAS within a cell, including components that participate in A II production and its receptors and effector proteins. In the heart, angiotensinogen is internalized and basal expression of RAAS components is very low. Intracellular RAAS is activated in response to pathologic conditions such as pressure or volume overload, myocardial infarction, and diabetes.
Potential mechanisms of action include direct intracellular A II effects on the heart, which are non–AT 1 receptor mediated, and intracellular A II regulation of the AT 1 receptor, providing an improved response to extracellular A II. These mechanisms have been suggested to have a role in left ventricular hypertrophy, activation of metalloproteinases with facilitation of plaque rupture, and pathogenesis of vascular disease. Intracellular A II actions are not blocked by ACE inhibitors or ARBs because of their inability to enter the cell and to block these pathways. More complete RAAS inhibition may be provided by direct renin inhibitors that can block both extracellular and intracellular pathways.
Alternative Pathways of Angiotensin II Production
In addition to intracellular RAAS, alternative pathways of A II production independent of ACE, termed ACE escape, have been established. These include chymase and ACE-2–dependent pathways. Chymase is a protease stored within the secretory granules of mast cells; it is present within the blood vessels, the heart, and other tissues but does not circulate within the blood. In blood vessels, chymase is localized to mast cells in the adventitia, whereas ACE is localized in the vascular endothelium. In normal vasculature, circulating A I does not readily penetrate into the interstitial space, and hence A I is converted to A II in an ACE-dependent fashion. In the setting of vascular injury or inflammation, mast cells are activated, allowing a chymase-dependent conversion of A I to A II.
Although chymase may have only a limited physiologic role in the generation of A II, residual A II formation may be observed in the presence of ACE inhibitor due to this chymase-dependent pathway. Other effects of chymase, outside of the A II–mediated effects, include promotion of atherosclerosis through the prevention of cholesterol removal by HDL, intimal hyperplasia of injured arteries, potential erosion and rupture of coronary atheroma leading to an acute coronary syndrome, and stimulation of transforming growth factor-β (TGF-β) leading to cardiac fibrosis.
Angiotensin-converting enzyme 2 (ACE-2) is a peptidase present throughout the cardiovascular system, including in macrophages, vascular endothelium, smooth muscle cells, and potentially cardiac myocytes. It has been shown to convert A I and A II to Ang-(1-9) and Ang-(1-7), respectively. The full physiologic importance of this pathway has yet to be determined. However, ACE-2 may have a counterregulatory role with ACE in fine-tuning the RAAS system through increased production of Ang-(1-7), balancing A II vasopressor effects.
Kallikrein-Kinin System
The main effector protein of the kallikrein-kinin system is bradykinin, produced by the proteolytic cleavage of a high-molecular-weight kininogen by kallikrein. Bradykinin has a variety of biologic effects, some cardioprotective. These effects are mediated through BK 1 and BK 2 receptors located on endothelial cells. The BK 1 receptor is rarely expressed in normal tissue but is upregulated in pathologic states associated with inflammation and tissue injury. It causes stimulation of smooth muscle cells, cell proliferation, and collagen synthesis. The BK 2 receptor, on the other hand, leads to increased stimulation of nitric oxide and prostacyclin, exerting vasodilator, anti-ischemic, and antiproliferative effects. Kinins are released in the setting of ischemia, myocardial infarction, and heart failure, and the ability of ACE inhibitor to prevent the breakdown of kinins represents a potential mechanism contributing to the drug’s cardioprotective effects.
Prorenin and Prorenin Receptor
Renin is synthesized as a prohormone, prorenin, and stored in granules within the renal juxtaglomerular cells. Prorenin is activated by removal of its N terminus that covers the active site and blocks access to angiotensinogen. The recent discovery of the prorenin receptor has expanded the physiologic role of this once believed inactive prohormone. The prorenin receptor binds to both renin and prorenin and has been localized to the vascular smooth muscle, the heart, and the distal and collecting tubules of the kidney.
When renin binds to the receptor, its activity is amplified fivefold, accelerating the production of A I and in turn A II. Prorenin, when it is bound to the receptor, can undergo nonproteolytic activation by unfolding of the peptide, exposing its active site. This allows prorenin to assume full activity and to contribute to the production of A I. Independent of A II, both renin and prorenin exert physiologic effects through the prorenin receptor. The activated receptor stimulates the production of TGF-β and heat shock proteins, increasing levels of PAI-1 and collagen involved in organ fibrosis and actin filament dynamics involved in contractility, hypertrophy, and apoptosis.
Plasma Renin Activity
Renin is the primary effector hormone of the RAAS, the first and rate-limiting step in A II synthesis. Plasma renin concentration is a measure of the amount of renin in the circulation, and plasma renin activity is a measure of its catalytic activity. Plasma renin activity correlates with the rate at which A I is generated and is a marker of the activation of the RAAS.
Early data have identified that elevated plasma renin activity has a direct relationship with adverse cardiovascular outcomes, including CHF and death, particularly in patients with CAD. More recently, several studies by the Intermountain Heart Collaborative Study Investigators have demonstrated a correlation of plasma renin activity to both worsened ischemia and heart failure outcomes at 3 years in patients with mild and moderate coronary atherosclerosis. The use of ACE inhibitors or ARBs can increase plasma renin activity by inhibiting the feedback loop that governs activity of the RAAS. The role and importance of prorenin, plasma renin concentration, and plasma renin activity have raised interest in the use of direct renin inhibitors (see Table 11-3 ).
Association Between Renal Insufficiency and Cardiovascular Disease
Many studies have demonstrated that the relationship between renal dysfunction and increased cardiovascular morbidity and mortality extends across the spectrum of renal dysfunction to include even the mildest degree of renal impairment. This relationship is also maintained across varying degrees of baseline cardiovascular health. In the general population and in patients with hypertension, stable CAD, post–myocardial infarction, or heart failure, there is an independent and strong correlation between worsening renal function and an increase in cardiovascular events. Baseline GFR has even been suggested to be a stronger predictor of mortality than either left ventricular ejection fraction or New York Heart Association class.
The increased cardiovascular risk for individuals with renal insufficiency is explained in part by the increased prevalence and severity of diabetes and hypertension ( Fig. 11-3 ). However, other mechanisms have been proposed to contribute to the increased risk of cardiovascular events in renal insufficiency. These mechanisms include an increase in endothelial dysfunction, an increase in oxidant stress and inflammation, progressive dyslipidemia, and stimulation of the RAAS that accompanies this disorder.

In renal disease, the initial insult to the kidney results in a decline in the GFR, noted by an increase in serum creatinine concentration. When nephrons are damaged, the remaining nephrons undergo hypertrophy with alterations in arteriolar tone. This in turn increases hydraulic pressure within the glomerular capillaries. The filtration capacity of the remaining nephrons increases, minimizing the functional consequences of nephron loss. The high glomerular capillary pressure enlarges the glomerular membrane pores through a process partially attributed to angiotensin (A II), which subsequently increases the number of proteins, both albumin and nonselective proteins, filtered. It is these proteins that become reabsorbed by the proximal tubular cells and may stimulate a vasoactive and inflammatory framework leading to a systemic vascular disease that can affect the kidneys as well as other arterial systems in the body, including the heart. Endothelial dysfunction, which is associated with alterations in vasomotor tone, inflammation, and oxidative stress, plays a key role in remodeling of the vasculature and increases the risk for atherosclerosis.
Described mediators of inflammation and coagulation known to be increased during renal insufficiency include C-reactive protein, fibrinogen, homocysteine, interleukin-6, factor VII/VIII, von Willebrand factor, D-dimer, and plasmin-antiplasmin complex. These mediators, derived in part from increased A II and the modifications of lipids, directly affect endothelial function and vascular remodeling through enhanced oxidative stress. Increased oxidative stress produces reactive oxygen species mainly through the activation of nicotinamide adenine dinucleotide (NADPH) oxidase and the uncoupling of endothelial synthase. The production of reactive oxygen species directly reduces nitric oxide activity, promoting endothelial dysfunction and atherosclerosis.
Dyslipidemia associated with renal insufficiency further contributes to the production of reactive oxygen species and inflammation. Notable alterations in lipid synthesis include elevated levels of lipoprotein(a) and triglycerides, decreased levels of apo A and HDL, and the decreased ability to reduce oxidized, small dense LDL. These defects are the result of a combination of overproduction and clearance defects in apo B–containing lipoproteins. The dyslipidemia of renal disease is complex, with an atherogenic profile similar to that of metabolic syndrome. In general, the severity of dyslipidemia correlates with the severity of proteinuria.
It has been demonstrated that the severity of atherosclerosis is highly correlated with severity of glomerulosclerosis and of renal arteriolosclerosis. Renal insufficiency may be a marker for generalized atherosclerotic burden. However, the presence of these altered pathways has been noted in people with renal insufficiency but with no evidence of clinical or subclinical cardiovascular disease. The prevalence of endothelial dysfunction, low-grade inflammation, dyslipidemia, and RAAS stimulation associated with renal disease may explain the acceleration of atherosclerosis. In combination with hypertension and associated left ventricular hypertrophy, this may lead to a reduction in coronary reserve, explaining the prevalence of coronary ischemia and cardiovascular events in people with renal insufficiency.
Association Between Proteinuria and Cardiovascular Disease
Whereas an elevated serum creatinine concentration reflects a decline in the GFR, an increased rate of albumin or protein excretion reflects a derangement in the glomerular filtration barrier. The presence of abnormal quantities of albumin in the urine often precedes renal functional deterioration. Microalbuminuria, an albumin-to-creatinine ratio (ACR) of 30 to 300 mg/g, correlates with nephrosclerosis; macroalbuminuria (ACR > 300 mg/g) generally indicates established renal parenchymal damage.
Proteinuria (or albuminuria) has been shown to be an independent marker of cardiovascular risk. The link between albuminuria and adverse cardiovascular events was first recognized with macroalbuminuria, later with microalbuminuria, and currently with any evidence of albuminuria. It has been suggested that proteinuria is a continuous cardiovascular risk factor, whereas microalbuminuria is a designated threshold for renal functional deterioration in individuals with and without diabetes.
Proteinuria, despite its association with renal injury, may also reflect a systemic increase in endothelial permeability or dysfunction. The vascular endothelium has an important role in regulating the transport of proteins across vessel walls. Endothelial dysfunction, as a result of shear stress, may allow the deposition of lipoproteins in the subendothelial space, promoting atherogenesis. Further injury to the endothelium results in increased cell and platelet adhesiveness, greater permeability to inflammatory cells, and altered production of vasoactive mediators, specifically nitric oxide. This suggests that proteinuria may be a marker of generalized damage to the peripheral vasculature, paralleling the degree of cardiac organ damage.
The Irbesartan Diabetic Nephropathy Trial (IDNT) and Reduction of Endpoints in NIDDM with the Angiotensin II Antagonist Losartan (RENAAL) trial were the first trials to help establish proteinuria as a cardiovascular risk factor. IDNT enrolled subjects with type 2 diabetes, hypertension, and macroalbuminuria. A total of 1715 subjects were randomized to irbesartan, amlodipine, or placebo and were observed for a mean period of 2.6 years. The primary outcome was a composite of serum creatinine doubling, end-stage renal disease (ESRD), or death. Although irbesartan proved to have a significant benefit compared with other therapies, no difference was detected on a secondary outcome of cardiovascular events. Further analysis of baseline albuminuria and cardiovascular composite endpoint showed that the proportion of patients who experienced cardiovascular events progressively increased with increasing quartiles of albuminuria, a 1.3-fold increased risk for each natural log unit increase in ACR.
RENAAL enrolled the same population of subjects as IDNT did, with a total of 1513 subjects randomized to either losartan or placebo. Baseline albuminuria was again shown to be a predictor of composite cardiovascular endpoint as well as heart failure alone. In addition, the change in urinary albumin from baseline to 6 months was the only correlate of adverse cardiovascular outcomes; a 50% reduction in baseline albuminuria translated into an 18% reduction in cardiovascular events and a 27% reduction in the risk of heart failure.
Other trials, such as the Heart Outcomes Prevention Evaluation (HOPE) and the Losartan Intervention For Endpoint reduction in hypertension (LIFE), reconfirmed the association between varying degrees of proteinuria and cardiovascular risk, independent of blood pressure. Reductions in proteinuria over time translate into improved outcomes, and it appears that proteinuria may even be more predictive than the other traditional cardiovascular risk factors. Proteinuria can be used as a potential target or indicator of therapeutic response with antihypertensive therapy.
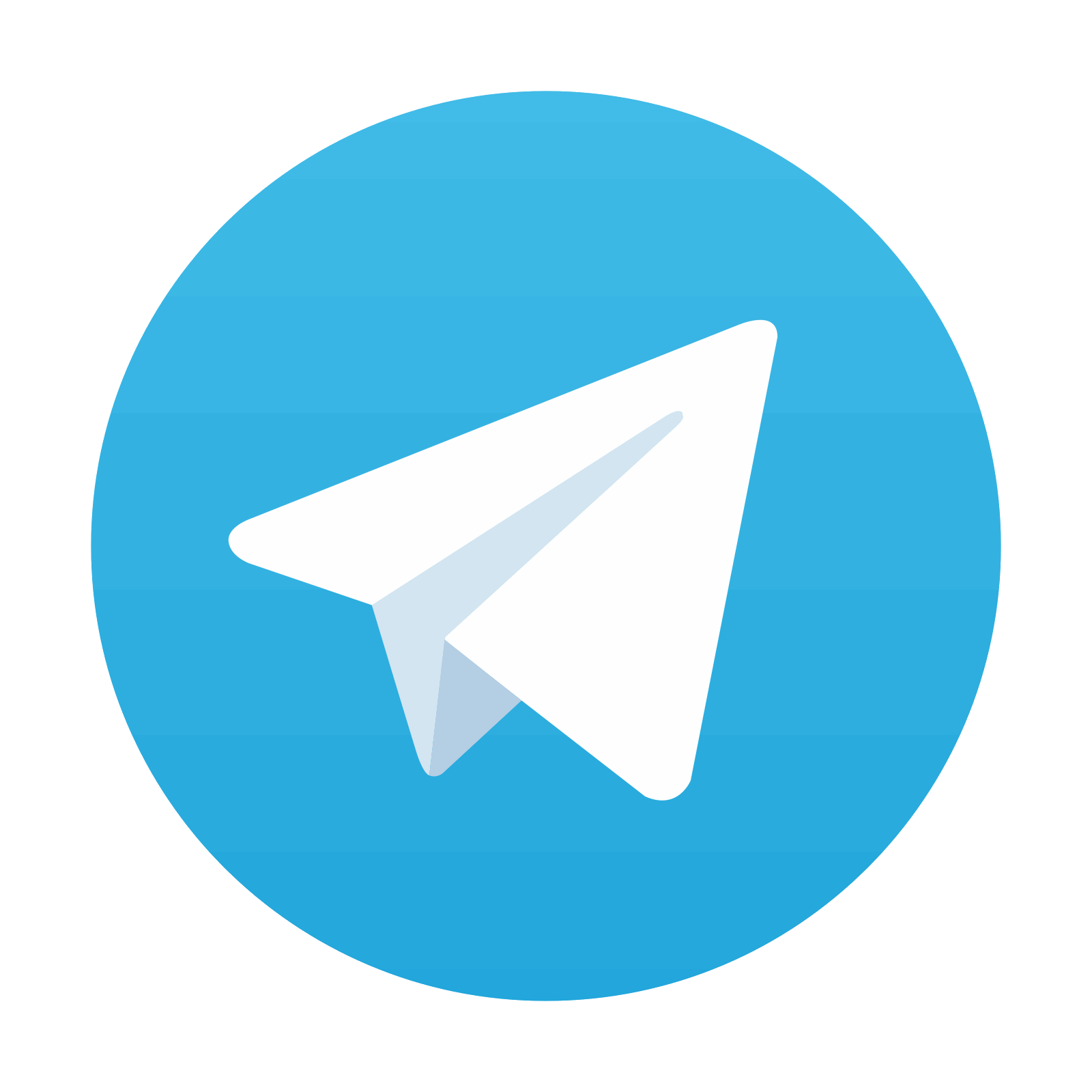
Stay updated, free articles. Join our Telegram channel

Full access? Get Clinical Tree
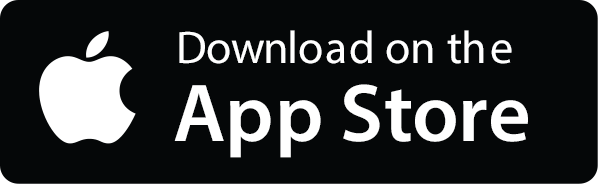
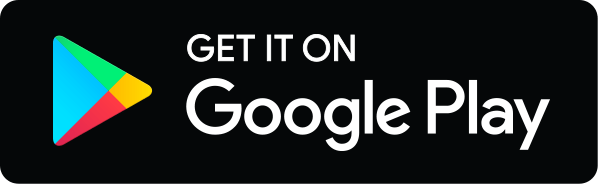