Key Points
- •
The incidence and prevalence of most cardiovascular diseases increase with advancing age.
- •
Age is the dominant risk factor for cardiovascular diseases, but age has traditionally been viewed as a nonmodifiable risk factor.
- •
Physiologic aging of the cardiovascular system is associated with myriad structural and functional alterations in the heart and in the blood vessels, which are themselves risk factors for cardiovascular diseases and thus may help explain the “risky” aspects of aging.
- •
The hallmarks of arterial aging include thickening and stiffening of the walls of the central arteries and endothelial dysfunction.
- •
Cardiac aging includes structural alterations in the heart, deficits in reserve functions, alterations in calcium regulation, and increased generation of reactive oxygen species during stress.
- •
Clinical interventions to retard cardiovascular aging include lifestyle changes (e.g., exercise, smoking cessation) and pharmacologic interventions (e.g., renin-angiotensin-aldosterone antagonists, antihypertensive medications, statins).
- •
Future studies should examine whether interventions aimed at retarding cardiovascular aging can have a positive impact on the adverse cardiovascular effects of accelerated cardiovascular aging and attenuate the impact of age as the dominant risk factor for cardiovascular diseases. Importantly, this should help alter our view of aging from an immutable risk factor to one that is amenable to modification and prevention.
The epidemic of cardiovascular diseases has taken on a global dimension and is no longer restricted to Western societies. Cardiovascular diseases now represent more than 30% of all deaths worldwide, and by the year 2020, they are expected to surpass infectious diseases as the leading cause of mortality and disability. According to the World Health Report, cardiovascular diseases were responsible for 15 million annual deaths worldwide, of which 9 million were in developing countries and 2 million in economies in transition. This situation is expected to worsen as the world population in both industrialized and developing countries is aging. For example, in the United States, 35 million people are older than 65 years, and the number of older Americans is expected to double by the year 2030. In many developing countries, the older population is progressively becoming more predominant as life expectancy increases.
The clinical and economic implications of this demographic shift are staggering because age is the most potent individual risk factor for cardiovascular diseases, especially in individuals older than 50 years. Both the incidence and prevalence of hypertension, coronary artery disease, congestive heart failure, and stroke increase exponentially with age. In older community-dwelling healthy volunteers, the incidence of silent coronary atherosclerosis, assessed by combined electrocardiographic treadmill stress testing and thallium perfusion imaging, increases dramatically with age.
Age influences not only the incidence and prevalence of coronary atherosclerosis but also the severity and prognosis of this disease. In survivors of a myocardial infarction, age is an independent predictor of short- and long-term morbidity, mortality, and disability, even after adjustment for the infarct size and location, the number of diseased vessels, and the extent of coronary artery disease. Similar considerations pertain to the impact of age on other cardiovascular diseases, such as hypertension, congestive heart failure, and stroke.
In spite of the fact that age is the dominant risk factor for cardiovascular diseases, most of the research efforts on prevention of these diseases have ignored age and have focused instead on development of interventions that target “traditional” cardiovascular risk factors (such as hypertension and hyperlipidemia) or identification of newer risk markers. This is because age has usually been viewed as an unmodifiable risk factor and therefore one that is not amenable to prevention or treatment. However, this concept has recently been challenged by an emerging school of thought, which proposes that aging can indeed be construed as a modifiable risk factor.
The key paradigm shift is that the components of aging associated with cardiovascular disease risk should no longer be simplistically attributed to an increased time of exposure to other established cardiovascular risk factors. Instead, the risky components of aging need to be recognized as accelerated or dysregulated age-associated alterations in the cardiovascular system, at the molecular, enzymatic, biochemical, cellular, histologic, and organismal levels. In this chapter, we review some of these alterations, at both the microscopic and clinical levels, with a view toward illustrating the overlapping features of aging with some cardiovascular diseases and risk factors. We provide an integrated view of the risky components of cardiovascular aging and of age-disease interactions, which are in dire need of targeted preventive and therapeutic strategies.
Epidemiology Of Cardiovascular Diseases In Older Individuals
The incidence and prevalence of most cardiovascular diseases increase with advancing age in both sexes. For example, the incidence of heart failure in the Framingham Heart Study (1980-2003) per 1000 person-years was 9.2 and 4.7 in men and women between the ages of 65 and 74 years, 22.3 and 14.8 in men and women between the ages of 75 and 84 years, and 41.9 and 32.7 in men and women older than 85 years. The prevalence of heart failure according to NHANES (2003-2006) was 2% and 1% in men and women between the ages of 40 and 59 years, 9% and 5% in men and women between the ages of 60 and 79 years, and 15% and 12% in men and women older than 80 years.
The prevalence of hypertension (defined as systolic blood pressure higher than 140 mm Hg or diastolic blood pressure higher than 90 mm Hg) in NHANES (2003-2006) was 39% and 38% in men and women between the ages of 45 and 54 years, about 54% in men and women between the ages of 55 and 64 years, 65% and 71% in men and women between the ages of 65 and 74 years, and 65% and 77% in men and women older than 75 years.
The incidence of stroke in ARIC (1987-2001) per 1000 person-years was 2.4 for white men and women between the ages of 45 and 54 years and 9.7 and 7.2 for black men and women in the same age range. These numbers increased to 6.1 and 4.8 for white men and women between the ages of 55 and 64 years and 13.1 and 10.0 for black men and women in this age range. The incidence of stroke increased to 12.1 and 9.8 in white men and women between the ages of 65 and 74 years and to 16.2 and 15 in black men and women in this age range. The prevalence of stroke in NHANES (2003-2006) was 1% and 6% in men and women between the ages of 40 and 59 years, 7% in men and women between the ages of 60 and 79 years, and 15% and 13% in men and women older than 80 years.
Last, the prevalence of coronary heart disease in NHANES (2003-2006) was 7% in both men and women between the ages of 40 and 59 years, 25% and 17% in men and women between the ages of 60 and 79 years, and 37% and 23% in men and women older than 80 years.
Importantly, age also influences the prognosis after a cardiovascular event, such that within 1 year after a myocardial infarction, the rate of death is 8% and 12% in white men and women between the ages of 40 and 69 years and 14% and 11% in black men and women in this age range. This rate increases to 27% and 32% of white men and women older than 70 years and to 26% and 28% of black men and women in this age range. Similarly, the risk of heart failure 5 years after a myocardial infarction increases with age. It is 7% and 12% in men and women between the ages of 40 and 69 years and 22% and 25% in men and women older than 70 years.
Aging of Central Arteries in Apparently Healthy Humans
The large central arteries had originally been construed as pipes that simply allowed blood to flow from the heart to distal organs and thus were originally dubbed “conduit” arteries. Changes in their mechanical properties with aging were considered to result largely from passive wear and tear in response to the unrelenting stress and strain incurred with each heartbeat throughout life. However, recent insights into the multiple signaling mechanisms that modulate their ability to adapt, repair, and remodel and that govern their structural and functional properties have left us marveling at these dynamic organs, which play pivotal roles in regulating pulsatile flow and modulating vascular impedance. The age-associated changes in central arterial structure and function in primates and in apparently healthy humans are summarized in Table 25-1 . Some of these features are discussed in the following sections.
Aging | ||||
---|---|---|---|---|
Humans >65 years | Monkeys 15-20 years | Rats 24-30 months | Rabbits 2-6 years | |
Luminal dilation | + | + | + | + |
↑ Stiffness | + | + | + | + |
Endothelial dysfunction | + | + | + | + |
Diffuse intimal thickening | + | + | + | + |
Collagen degradation | + | + | + | + |
Collagen deposition | + | + | + | + |
VSMC number | + | + | + | + |
Macrophages | + | − | − | − |
T cells | + | − | − | − |
↑ Local angiotensin II–ACE | + | + | + | + |
MMP dysregulation | + | + | + | ? |
↑ MCP-1/CCR2 | + | + | + | + |
↑ ICAM | + | + | + | ? |
↑ TGF-β | + | + | + | + |
↑ NADPH oxidase | + | + | + | + |
↓ VEGF | + | ? | ? | + |
↓ Nitric oxide bioavailability | + | + | + | + |
Overall cardiovascular structure and function vary dramatically among older individuals. Identification of cardiovascular structural and functional changes that reflect an “aging process” is a formidable task. Neither functional differences among individuals in cross-sectional studies nor changes over time within a given individual in longitudinal studies are necessarily manifestations of an aging process. Rather, interactions among aging, disease, and lifestyle must be considered in interpreting age-associated changes in cardiovascular structure and function as measured in various studies.
Lumen Diameter
Cross-sectional studies show that on average, the central aorta dilates with age, leading to an increase in lumen size ( Fig. 25-1A ). Studies found an inverse and independent association between aortic root diameter and pulse pressure, suggesting that luminal size may play a role in the pathogenesis of systolic hypertension. This provocative concept awaits the results of longitudinal studies to clarify the significance of the cross-sectional relationship between aortic root diameter and pulse pressure, which could have important implications for putative future therapies to delay or to prevent systolic hypertension, the dominant form of hypertension in older individuals.

Wall Thickness
Aging is also associated with thickening of the walls of the central arteries ( Fig. 25-1B ). Intima-media thickness rises nearly threefold between the third and ninth decades of life, an increase that is mainly attributable to an increase in intimal thickness. The age-associated intima-media thickening is often ascribed to atherosclerosis, and intima-media thickness is often equated with subclinical atherosclerosis. This is because a growing body of literature has shown that traditional cardiovascular risk factors and prevalent cardiovascular diseases are associated with intima-media thickening and that intima-media thickening is a potent and independent predictor of adverse cardiovascular events. However, intima-media thickness is only modestly correlated with the extent and severity of coronary artery disease, and it can be influenced by factors other than atherosclerosis (e.g., aging, hypertension).
For example, intima-media thickening increases with advancing age in animal models that are devoid of atherosclerosis, thus indicating that these age-associated alterations are due to the aging process and not to superimposed atherosclerosis. This thickening is due to myriad age-associated biochemical, cellular, and morphologic changes in the arterial wall, which are modulated by the same factors that have been implicated in the genesis of various cardiovascular diseases. Thus, intima-media thickening should not be construed as synonymous with “subclinical atherosclerosis,” particularly in the absence of plaques. Nonetheless, it remains a useful marker of subclinical vascular disease.
Wall Remodeling
Although we have discussed arterial wall thickness and luminal diameter separately, arteries undergo a remodeling process, in response to hemodynamic and metabolic stimuli, that can be better characterized by an index that combines the diameter, wall thickness, and vascular mass variables than by each of these variables individually. For example, in the carotid artery, three remodeling patterns that deviate from normal can be described : (1) concentric remodeling, due to a smaller lumen in the setting of a normal vascular mass; (2) vascular hypertrophy, due to an increase in vascular mass, which results from wall thickening, in the setting of a normal lumen size; and (3) eccentric hypertrophy, due to an increase in vascular mass in the setting of a dilated lumen. In a study of normotensive and untreated hypertensive Taiwanese subjects, these geometric patterns yielded prognostic information, with the two hypertrophic groups being associated with an increased risk of cardiovascular events. This underscores the importance of integrating the markers of arterial aging to better appreciate their clinical utility.
Arterial Stiffness
One of the hallmarks of central arterial aging is an age-associated increase in stiffness ( Fig. 25-1C ). Central arterial stiffness can be assessed by several invasive and noninvasive indexes. Recently, carotid-femoral pulse wave velocity has been anointed the “gold standard” for the noninvasive assessment of central arterial stiffness. Pulse wave velocity has been shown to be an independent predictor of morbidity and mortality in healthy subjects and in individuals with various levels of cardiovascular risk. It is likely that arterial stiffness is not only a risk marker for cardiovascular diseases but also a risk factor for these diseases. Indeed, increased central arterial stiffness leads to an increase in the load (afterload) on the left ventricle, leading to increased hypertrophy and increased oxygen consumption. Furthermore, increased stiffness is associated with a decrease in diastolic blood pressure, which could compromise coronary blood flow, which occurs predominantly in diastole.
The age-associated increase in stiffness has traditionally been attributed to the fraying and breakdown of elastin due to the lifelong repeated cycles of distention and recoil of the central aorta as well as the increased deposition and covalent cross-linking of collagen molecules. It is now recognized that arterial stiffening can be modulated by several factors including lifestyle considerations (e.g., salt intake, exercise, weight loss), signaling pathways (e.g., nitric oxide), inflammation, and genetics. Interventions to prevent or to delay arterial stiffening have predominantly focused on pharmacologic antihypertensive therapies. However, these strategies are aimed at lowering blood pressure, whereby the reduction in stiffness is a secondary effect due to reverse remodeling of the arterial wall in response to the lower pressures.
Because arterial stiffness is a potent predictor of mortality and morbidity, independent of blood pressure, a more direct approach that would target the stiffening process is desirable. However, there is a dearth of interventions at present that have attempted this, with the one notable exception being the advanced glycation end products cross-link breaker Alagebrium (ALT-711). This compound cleaves the covalent cross-link bonds that form between adjacent collagen fibrils in the medial layer of the arterial wall and that contribute to increasing the tensile strength of the collagen molecules. Alagebrium has been shown to reduce arterial stiffness in rodents and in nonhuman primates.
In humans with systolic hypertension and increased arterial stiffness, a randomized, double-blind, placebo-controlled study showed that treatment with Alagebrium for 8 weeks resulted in a significant decrease in arterial stiffness, without an apparent disproportionate decline in mean arterial pressure, systemic vascular resistance, heart rate, or cardiac output. Although a multicenter, randomized, double-blind, placebo-controlled clinical trial failed to show an effect of Alagebrium on blood pressure, the primary effect of this compound should be a reduction in stiffness, and a reduction in stiffness may not necessarily translate into a reduction in blood pressure (at least in the short or intermediate term) because the lowering in stiffness could be accompanied by an increase in stroke volume (lower afterload on the heart), which could potentially offset the effects of a lower stiffness on blood pressure. Unfortunately, these hemodynamic features were not assessed in the aforementioned clinical study.
In contrast to the central elastic arteries, the stiffness of the muscular arteries (e.g., brachial and femoral arteries) does not increase with age. Thus, the manifestations of arterial aging may vary among the different vascular beds, reflecting differences in the structural compositions of the arteries and perhaps differences in the age-associated signaling cascades that modulate the arterial properties or differences in the response to these signals across the arterial tree.
Reflected Waves
The increased load that is imposed on the heart by increasing stiffness is due not only to an increase in characteristic impedance (intrinsic stiffness of the vessel) but also to an increase in central systolic blood pressure. The latter is due, in part, to a shift in the return of reflected waves to an earlier time during systole, which leads to an increase in central pressure augmentation and thus to a higher central systolic blood pressure and a higher central pulse pressure. It is now appreciated that the timing and amplitude of reflected waves are governed both by arterial stiffness and by properties of the distal reflecting sites (usually areas of impedance mismatch). These reflected waves play an important role in influencing the central to peripheral pressure augmentation across the arterial tree. Even though peripheral systolic pressure and pulse pressure increase with age, the central to peripheral pressure amplification decreases with age.
Central Blood Pressure
Recent methodologic advances have made it possible to noninvasively estimate central blood pressures with relative ease. This is fueling a growing clinical interest in assessing central blood pressures. The Conduit Artery Functional Endpoint (CAFÉ) study showed that the combination of the calcium channel blocker amlodipine with or without the angiotensin-converting enzyme (ACE) inhibitor perindopril in the treatment of hypertension achieved a greater reduction in central blood pressure than the beta blocker atenolol with or without a diuretic, even though the peripheral blood pressures were not significantly different between the two groups. CAFÉ was a substudy of the Anglo-Scandinavian Cardiac Outcomes Trial (ASCOT), which showed that patients in the amlodipine-perindopril arm had better clinical outcomes than those in the atenolol-diuretic arm.
The findings from CAFÉ suggest that the lower central blood pressures achieved with amlodipine-perindopril may explain, in part, the differences in outcomes between the two groups. The usefulness and feasibility of integrating measurements of central arterial blood pressures into routine medical practice are areas of intense ongoing research. As a first step, studies have established that central pressures are independent predictors of outcomes and may be even better predictors of cardiovascular mortality than peripheral blood pressures.
Increased central arterial stiffness is associated with an increase in central systolic blood pressure and a decrease in diastolic blood pressure, resulting in a widening of the pulse pressure. This is a likely explanation of the age-associated changes in blood pressures, whereby systolic blood pressure continues to increase with advancing age, whereas diastolic blood pressure increases until the fifth decade, then levels off and starts to decrease after the age of 60 years. Numerous clinical and epidemiologic studies in several different populations with varying prevalence of cardiovascular diseases have demonstrated that pulse pressure is an important predictor of adverse outcomes, often more potent than systolic or diastolic blood pressures. However, the clinical role of pulse pressure has remained limited to a few clinical conditions (e.g., advanced heart failure, severe aortic regurgitation), although it could serve as an easily obtainable surrogate marker of stiffness.
Arterial-Ventricular Coupling
In addition to the age-associated alterations in arterial properties, advancing age is also associated with alterations in left ventricular (LV) structure and function. Importantly, the left ventricle and the central arteries have bidirectional constant interactions. One useful index of this interaction, termed arterial-ventricular coupling, can be assessed by the ratio of effective arterial elastance (Ea), a measure of the net arterial load exerted on the left ventricle, to LV end-systolic elastance (ELV), a load-independent measure of LV chamber performance. Ea increases with advancing age, predominantly because of the age-associated increase in arterial stiffness, as peripheral vascular resistance and heart rate (the other main determinants of Ea) do not significantly change with age.
ELV also increases with advancing age. Interestingly, even though Ea and ELV both increase with advancing age, their ratio, Ea/ELV, remains relatively unchanged across the age spectrum in men, suggesting that the increases in Ea and ELV are matched. This tight coupling is thought to allow the cardiovascular system to optimize energetic efficiency. In contrast, in healthy women, Ea/ELV declines slightly with advancing age, which is due to a disproportionate age-associated increase in ELV compared with the age-associated increase in Ea. This suggests that in women, but not in men, aging exerts a greater impact on ventricular than arterial properties.
Therapies that improve the coupling of ventricular and vascular elastances are likely to improve cardiac function and exercise tolerance in healthy subjects. This concept is supported by two studies in healthy older subjects. Administration of the direct vasodilator sodium nitroprusside caused reductions in reflected waves (manifested as a reduction in preload), resulting in reduced cardiac volumes and higher ejection fraction at rest and during maximal exercise compared with placebo therapy. Administration of intravenous verapamil reduced noninvasive indexes of arterial and ventricular systolic stiffness and improved exercise tolerance and oxygen consumption before reaching the anaerobic threshold.
Aging of Endothelial Cells
An important feature of arterial aging is age-associated compromise in endothelial function. In humans, the function of endothelial cells in central arteries has not been directly assessed. Nonetheless, endothelial function has been assessed in the brachial artery, where measurement of vasoreactivity by both agonist- and flow-mediated techniques has shown that it declines with advancing age.
Endothelial cells play a pivotal role in modulating myriad arterial structural and functional properties, including vascular tone, vascular permeability, angiogenesis, production of extracellular matrix proteins and of growth factors, neurohormonal signaling (nitric oxide, renin-angiotensin-aldosterone system, sympathetic nervous system), and response to inflammation. The critical role of endothelial cells in several cardiovascular diseases is increasingly being appreciated. For example, endothelial dysfunction has been described in patients with hypertension and, interestingly, in the normotensive offspring of hypertensive individuals, suggesting that endothelial dysfunction may precede the development of clinical hypertension. Endothelial dysfunction has also been implicated in the pathogenesis of atherosclerosis and is one of the earliest pathologic manifestations of this disease. Interestingly, arterial regions with low shear stress are particularly vulnerable to the development of atherosclerosis, and vascular wall shear stress is an important modulator of endothelial cell morphology and endothelial cell metabolic and synthetic functions. Endothelial dysfunction, in both the coronary and peripheral arterial beds, has been shown to be an independent predictor of future cardiovascular events.
Endothelial dysfunction can also be induced by loss of telomere function. Telomeres are DNA-protein complexes that form the ends of chromosomes, and they shorten with each replicative cycle, which is why they have been proposed to be possible indicators of biologic aging. Interestingly, inhibition of telomere shortening can suppress the age-associated endothelial dysfunction.
Vascular cell senescence is increasingly recognized as an important feature of arterial aging. Thus, there is growing interest in and excitement at the promises held by regenerative therapies. Endothelial cells play a pivotal role in angiogenesis, the process through which new vessels grow from existing microvasculature. However, studies in animal models of aging indicate that angiogenesis is impaired with advancing age. Endothelial progenitor cells offer the prospects of putatively replenishing or replacing senescent endothelial cells. However, both the number and activity of endothelial progenitor cells appear to be reduced with aging, suggesting an age-associated impairment in regenerative and repair capacities, which, experimentally, can be reversed with growth hormone–mediated increase in insulin-like growth factor 1 (IGF-1) levels.
Plasminogen Activator Inhibitor 1
Thrombotic cardiovascular diseases increase in incidence in the elderly, a tendency dependent on the age-related changes in vascular and hemostatic systems that include platelets, coagulation, and fibrinolytic factors as well as in the endothelium. The hypercoagulability of and advanced sclerotic changes in the vascular wall may contribute to the increased incidence of thrombosis in the elderly. One of the important key genes for aging-associated thrombosis is plasminogen activator inhibitor 1 (PAI-1), a principal inhibitor of fibrinolysis.
The expression of PAI-1 not only is elevated in the elderly but also is significantly induced in a variety of pathologic conditions associated with the process of aging. These conditions include obesity, insulin resistance, emotional stress, immune responses, and vascular sclerosis and remodeling. Several cytokines and hormones, including tumor necrosis factor-α (TNF-α), transforming growth factor-β (TGF-β), angiotensin II, and insulin, positively regulate the gene expression of PAI-1.
The recent epidemic of obesity with aging in industrialized societies may heighten the risk for thrombotic cardiovascular disease because adipose tissue is a primary source of PAI-1 and cytokines. Emotional or psychosocial stress and inflammation also cause the elevated expression of PAI-1 in an age-specific pattern. Thus, PAI-1 could play a key role in the progression of cardiovascular aging by promoting thrombosis and vascular (athero)sclerosis. Further studies on the genetic mechanism of aging-associated PAI-1 induction will be necessary to define the basis for cardiovascular aging in relation to thrombosis.
Cardiac Aging
The preceding section focused on age-associated changes in the vasculature. Aging is also associated with structural and functional changes in the heart.
Cardiac Aging in Humans
With advancing age, the walls of the left ventricle increase in thickness, largely because of an increase in ventricular myocyte size and an increase in vascular impedance, and this helps moderate the increase in LV wall tension. Modest increases in collagen levels also occur with aging.
A unified interpretation of identified cardiac changes that accompany advancing age in otherwise healthy persons suggests that at least in part, these are adaptive, occurring to some extent in response to arterial changes that occur with aging ( Fig. 25-2 ). Prolonged contraction of the thickened LV wall maintains a normal ejection time in the presence of the late augmentation of aortic impedance. This preserves the systolic cardiac pumping function at rest. One disadvantage of prolonged contraction is that at the time of the mitral valve opening, myocardial relaxation is relatively more incomplete in older than in younger individuals and causes the early LV filling rate to be reduced in older individuals.

Structural changes and functional heterogeneity occurring within the left ventricle with aging may also contribute to this reduction in peak LV filling rate. However, concomitant adaptations—left atrial enlargement and an enhanced atrial contribution to ventricular filling—compensate for the reduced early filling and prevent a reduction of the end-diastolic volume. Age-associated changes in the tissue levels of or responses to growth factors (catecholamines, angiotensin II, endothelin, TGF-β, or fibroblast growth factor) that influence myocardial or vascular cells or their extracellular matrices (see later) may also have a role in the schema depicted in Figure 25-2 .
Biologic sex is a well-recognized factor in the physiology and pathophysiology of the cardiovascular system, including the aging heart (reviewed in references 40 and 41). Postmortem morphometric assessments in nonfailing human hearts have shown extensive age-related myocyte loss and hypertrophy of the surviving myocytes in male hearts but preserved ventricular myocardial mass and average cell diameter and volume in aging female hearts. These sex differences may stem, in part, from differences in the replicative potential of cardiac myocytes. Analysis of gene expression differences by sex and age in samples of the left ventricle from patients with dilated cardiomyopathy has identified more than 1800 genes displaying sexual dimorphism in the heart. A significant number of these genes were highly represented in gene ontology pathways involved in ion transport and G protein–coupled receptor signaling.
Cardiovascular Reserve
Impaired heart rate acceleration and impaired augmentation of blood ejection from the left ventricle, accompanied by an acute modest increase in LV end-diastolic volume, are the most dramatic changes in cardiac reserve capacity that occur with aging in healthy, community-dwelling persons ( Table 25-2 ). Mechanisms that underlie the age-associated reduction in maximum ejection fraction are multifactorial and include a reduction in intrinsic myocardial contractility, an increase in vascular afterload, and arterial-ventricular load mismatching.
Oxygen consumption | ↓ (50%) |
(A-V)O 2 | ↓ (25%) |
Cardiac index | ↓ (25%) |
Heart rate | ↓ (25%) |
Stroke volume | No change |
Preload | |
EDV | ↑ (30%) |
Afterload | ↑ |
Vascular (PVR) | ↑ (30%) |
Cardiac (ESV) | ↑ (275%) |
Cardiac (EDV) | ↑ (30%) |
Contractility | ↓ (60%) |
Ejection fraction | ↓ (15%) |
Plasma catecholamines | ↑ |
Cardiac and vascular responses to beta-adrenergic stimulation | ↓ |
Ventricular load is the opposition to myocardial contraction and the ejection of blood; afterload is the component of load that pertains to the time after excitation, as opposed to preload, before excitation. Although these age-associated changes in cardiovascular reserve are insufficient to produce clinical heart failure, they do affect its clinical presentation, that is, the threshold for symptoms and signs, or the severity and prognosis of heart failure secondary to any level of disease burden (e.g., chronic hypertension that causes either systolic or diastolic heart failure).
A sizeable component of the age-associated deficit in cardiovascular reserve is composed of diminished effectiveness of the autonomic modulation of heart rate, LV contractility, and arterial afterload. The essence of sympathetic modulation of the cardiovascular system is to ensure that the heart beats faster; to ensure that it retains a small size, by reducing the diastolic filling period, reducing LV afterload; to augment myocardial contractility and relaxation; and to redistribute blood to working muscles and to skin to dissipate heat. Each of the deficient components of cardiovascular regulation with aging, that is, heart rate (and thus filling time), afterload (both cardiac and vascular), myocardial contractility, and redistribution of blood flow, exhibits a deficient sympathetic modulation.
Multiple lines of evidence support the idea that the efficiency of postsynaptic beta-adrenergic signaling declines with aging. One line of evidence stems from the observation that cardiovascular responses to beta-adrenergic agonist infusions at rest decrease with age. A second type of evidence for a diminished efficacy of postsynaptic beta-adrenergic receptor signaling is that acute beta-adrenergic receptor blockade changes the exercise hemodynamic profile of younger persons to make it resemble that of older individuals. Significant beta blockade–induced LV dilation occurs only in younger subjects ( Fig. 25-3A ). The heart rate reduction during exercise in the presence of acute beta-adrenergic blockade is greater in younger versus older subjects ( Fig. 25-3B ), as are the age-associated deficits in LV early diastolic filling rate, both at rest and during exercise ( Fig. 25-3C ). It has also been observed in older dogs that the age-associated increase in aortic impedance during exercise is abolished by acute beta-adrenergic blockade.

Apparent deficits in sympathetic modulation of cardiac and arterial functions with aging occur in the presence of exaggerated neurotransmitter levels. Plasma levels of norepinephrine and epinephrine, during any perturbation from the supine basal state, increase to a greater extent in older compared with younger healthy humans. The age-associated increase in plasma levels of norepinephrine results from an increased spillover into the circulation and, to a lesser extent, reduced plasma clearance. The degree of norepinephrine spillover into the circulation differs among body organs; increased spillover occurs within the heart. Deficient norepinephrine reuptake at nerve endings is a primary mechanism for increased spillover during acute graded exercise. During prolonged exercise, however, diminished neurotransmitter reuptake might also be associated with depletion and reduced release and spillover. Cardiac muscarinic receptor density and function are also diminished with increasing age and might contribute to the decrease in baroreflex activity observed in aged subjects.
Cardiac Aging in Animal Models
Cardiac Structure
Cellular and molecular mechanisms implicated in age-associated changes in myocardial structure and function have been largely studied in rodents. Some underlying mechanisms that drive age-related cardiac remodeling are listed in Table 25-3 . The altered cardiac structural phenotype that evolves with aging in rodents includes an increase in LV mass due to an enlargement of myocyte size and focal proliferation of the matrix in which the myocytes reside, which may be linked to an altered cardiac fibroblast number or function. The number of cardiac myocytes becomes reduced because of necrosis and apoptosis, with necrosis predominating.
Structural Change | Functional Change | Ionic, Biophysical, and Biochemical Mechanisms | Molecular Mechanisms |
---|---|---|---|
↑ Myocyte size | Prolonged contraction | Prolonged cytosolic Ca 2+ transient | |
↓ Myocyte number | ↓ SR Ca 2+ pumping rate ↓ Pump site density | ↓ SR Ca 2+ pump mRNA No change in calsequestrin mRNA | |
Prolonged action potential | ↓ I Ca inactivation | ↑ Na + /Ca + exchanger mRNA | |
↓ I To density | |||
Diminished contraction velocity | ↓ α-MHC protein ↑ β-MHC protein ↓ Myosin ATPase activity ↓ RXRβ1 and γ mRNA ↓ RXRβ1 and γ protein ↓ Thyroid receptor protein | ↓ α-MHC mRNA ↑ β-MHC mRNA No change in actin mRNA ↓ RXRβ1 and γ mRNA | |
Diminished beta-adrenergic contractile response | ↓ Coupling beta-adrenergic receptor–acyclase No change in G i activation No change in BARK activity ↓ TNI phospholamban ↓ Phospholamban phosphorylation ↓ I Ca augmentation ↓ Ca i transient augmentation ↑ Enkephalin peptides ↑ Proenkephalin mRNA | ↓ Beta 1 -adrenergic receptor mRNA No change in BARK mRNA | |
↑ Matrix connective tissue | ↑ Myocardial stiffness | ↑ Hydroxyline proline content ↑ Activity of myocardial RAS | ↑ Collagen mRNA ↑ Fibronectin mRNA ↑ AT 1 R mRNA |
↑ Atrial natriuretic peptide | ↑ Atrial natriuretic peptide mRNA | ||
↓ Growth response | ↑ Induction of immediate-early genes | ||
↓ Heat shock response | ↓ Activation of HSF |
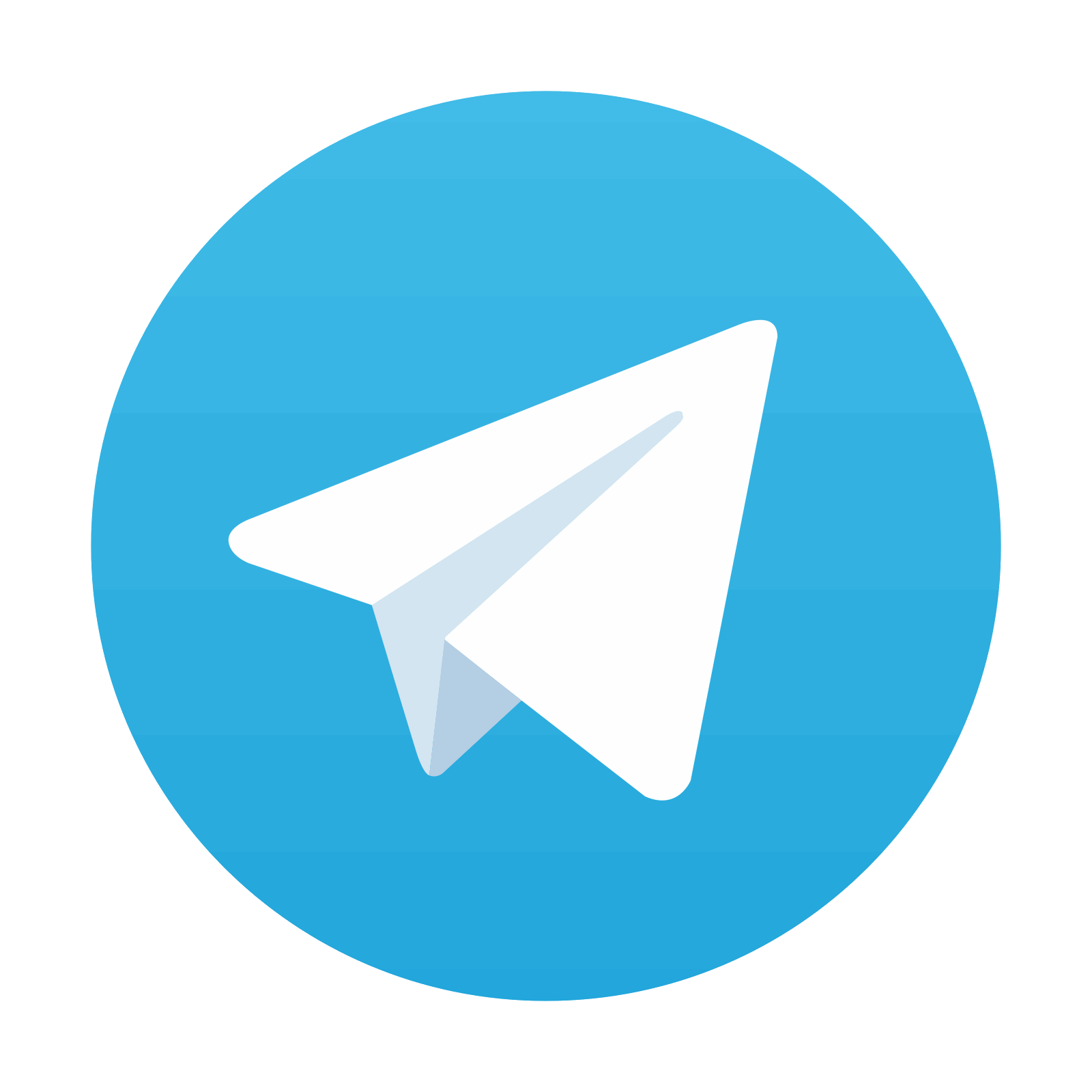
Stay updated, free articles. Join our Telegram channel

Full access? Get Clinical Tree
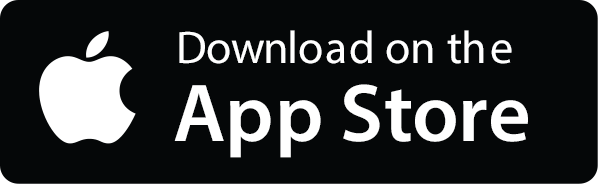
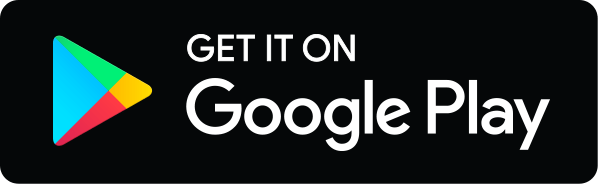
