Key Points
- •
Among patients with dyslipidemia, LDL-C is the primary target of therapy. LDL-C lowering reduces risk for CHD and its clinical sequelae, including unstable angina, ischemic stroke, myocardial infarction, and death.
- •
Atherogenesis is a complex disease whose etiology and progression are strongly influenced by the severity of a large number of risk factors. Low serum levels of HDL-C, hypertension, impairments in glucose metabolism, heightened systemic inflammatory tone, cigarette smoking, and others induce progressive arterial wall injury.
- •
The metabolic syndrome is characterized by a set of five risk factors: abdominal obesity; elevated blood pressure, triglycerides, and fasting glucose concentration; and low HDL-C. Metabolic syndrome develops secondary to the effects of insulin resistance and obesity.
- •
A number of preventive strategies can be used to target reductions in LDL-C to decrease the burden of CHD. The NCEP ATP III guidelines identify therapeutic lifestyle changes as the initial intervention to lower LDL-C.
- •
Statins, or 3-hydroxy-3-methylglutaryl-coenzyme A reductase inhibitors, are the most widely used drugs for lowering of LDL-C. The statins reduce cardiovascular morbidity and mortality.
- •
Bile acid sequestrants, nicotinic acid, fibrates, cholesterol absorption inhibition, and omega-3 fish oils also play important roles in LDL-C reduction and dyslipidemia management.
- •
Whereas LDL-C reduction has become the cornerstone of lipid management, apo B and non–HDL-C may be more accurate indicators of cardiovascular risk as well as measures by which to gauge LDL-lowering therapy.
- •
Clinical trials using statins and non-statin approaches to lipid lowering have demonstrated that for every 1 mg/dL reduction in serum LDL-C, there is a 1% reduction in risk for acute cardiovascular events.
Cardiovascular disease (CVD), including coronary heart disease (CHD), cerebrovascular disease, hypertension, and ischemic heart disease, is a major cause of mortality and morbidity worldwide, accounting for 17 million deaths. Aggressive risk reduction therapies are indicated for patients with and without CHD to improve survival, to reduce initial or recurrent events, and to improve quality of life for these patients. Among patients with dyslipidemia, low-density lipoprotein cholesterol (LDL-C) is the primary target of therapy as research has substantiated that elevated LDL-C is a major cause of morbidity and mortality and LDL-C lowering reduces the risk for CHD and its clinical sequelae, including unstable angina, myocardial infarction, and death. This chapter reviews the role of LDL-C in the development of atherosclerosis and outlines management strategies to reduce serum levels of this lipoprotein.
Historical Perspectives on Hypercholesterolemia
The importance of hypercholesterolemia in the development of atherosclerosis can be traced historically as a medical controversy, as atherosclerosis was considered an inevitable part of aging. Studies on rabbits demonstrating the impact of high-cholesterol diets on atherogenesis, published in 1913 by Anitschkow and Chalatov, substantiated the role of hypercholesterolemia, yet it took a decade of research to confirm that the findings from experimental atherosclerosis in animals could be extrapolated to humans.
The Framingham Heart Study and other prospective epidemiologic investigations demonstrated that risk for myocardial infarction, stroke, and death was proportional to serum cholesterol levels. In the Seven Countries Study, a direct relationship was found between serum cholesterol and risk for CHD among 12,763 middle-aged men. This relationship has been confirmed and extended in cohorts from 26 nations in the Multinational Monitoring of Trends and Determinants in Cardiovascular Disease (MONICA) study. The Ni-Hon-San study evaluated the impact of migration and progressive westernization of the diet among Japanese men residing in Nippon, Japan, or in Honolulu and San Francisco. As the intake of animal fat increased, serum cholesterol and risk for CHD mortality both increased. In the Multiple Risk Factor Intervention Trial (a cohort of 356,222 men), there was a clear gradient of continuously rising risk for CVD as serum cholesterol levels increased. Whereas the majority of total cholesterol is composed of LDL-C in most patients, it was important to evaluate whether LDL-C is an independent predictor of CHD. The Cooperative Lipoprotein Phenotyping study, the Atherosclerosis Risk in Communities study, and the Framingham study all demonstrated a direct and independent relationship between LDL-C and risk for cardiovascular morbidity and mortality. These relationships between total cholesterol and LDL-C and risk for CVD are remarkably consistent in populations studied throughout the world.
Acceptance of the lipid hypothesis that dyslipidemia is a causative factor in the development of atherosclerosis and CHD did not begin until the early 1960s, when research findings as well as the release of the American Heart Association’s recommendation for dietary modification as a first step toward implementation of a cholesterol-lowering strategy refocused attention on hypercholesterolemia. Advances in knowledge that ultimately validated the lipid hypothesis including understanding of the pathogenesis of atherosclerosis, large-scale clinical trial results including the Lipid Research Clinics Coronary Primary Prevention Trial, establishment of the National Cholesterol Education Program, and development and use of statins all led to confirmation of the role of dyslipidemia in the development of CHD.
Lipoprotein Metabolism
Cholesterol derived from both biliary and dietary sources is transported into jejunal enterocytes from the intestinal lumen by a sterol translocase known as Niemann-Pick C1-like 1 protein. Cholesterol, triglycerides, and phospholipids are packaged together with apoprotein B48 to form chylomicrons. Chylomicrons enter the enteric lymphatic system and are secreted into the central circulation through the lymphatic duct. The triglycerides in chylomicrons can be hydrolyzed by lipoprotein lipase in serum, yielding chylomicron remnant particles. Both chylomicrons and chylomicron remnants are taken up by hepatocytes. Intrahepatic cholesterol and lipids can be repackaged with apoprotein B100 into very-low-density lipoproteins (VLDL) and secreted into the central circulation.
The triglycerides in VLDL particles are hydrolyzed by lipoprotein lipase to yield, in sequence, intermediate-density lipoproteins (IDL) and then low-density lipoproteins (LDL). LDL particles tend to be highly enriched with cholesterol. All of the apo B100–containing lipoproteins are atherogenic. The sum of all atherogenic lipoproteins (VLDL + IDL + LDL) is defined as non–high-density lipoprotein (non-HDL). Non-HDL is a fairly sensitive measure of the total atherogenic lipoprotein burden in serum. LDL particles can be taken up into arterial walls. Alternatively, LDL particles are cleared from serum by the LDL receptor (LDLR) and LDLR-related protein expressed on the surface of hepatocytes. The cholesterol in LDL particles can then be shunted toward bile acid formation (rate-limiting step catalyzed by 7α-hydroxylase), it can be secreted into bile, or it can be repackaged into VLDL.
In patients with loss-of-function mutations in lipoprotein lipase, serum VLDL and triglyceride levels tend to be elevated. This impairs the conversion of VLDL into smaller, triglyceride-depleted lipoproteins. With this excess availability of triglyceride in VLDL, cholesteryl ester transfer protein catalyzes a 1 : 1 stoichiometric exchange for cholesterol between VLDL and LDL. This leads to progressive enrichment of the LDL particles with triglyceride, rendering them more vulnerable to lipolysis by the enzyme hepatic lipase. Hepatic lipase converts large buoyant LDL particles into smaller, denser and more numerous particles. Smaller LDL particles also tend to be more atherogenic: (1) biophysically, they can gain access into the subendothelial space more easily because they are smaller; (2) they are more easily oxidized than their larger counterparts; and (3) they have a lower affinity for the LDLR and hence reduced systemic clearance.
Some patients have a genetic predisposition to elevated serum levels of LDL-C. Hereditary hypercholesterolemias are frequently associated with premature multivessel coronary artery disease (CAD). Patients with familial hypercholesterolemia harbor a variety of mutations (these are indexed at www.ucl.ac.uk/fh/ ) in the gene for LDLR, resulting in reduced capacity for LDL clearance from serum. Heterozygotes often present with serum LDL-C of 250 to 350 mg/dL. Homozygotes can have LDL-C levels of 500 mg/dL or more and usually require LDL apheresis to control their serum levels of this lipoprotein.
Loss-of-function mutations in cholesterol 7α-hydroxylase are also associated with elevations in LDL-C and decreased bile acid biosynthesis. Patients with familial defective apo B100 have impaired capacity for LDL-C clearance secondary to polymorphisms in apo B100 (henceforth apo B), which reduce the affinity of this apoprotein for the LDLR. Another fascinating group of mutations linked to hypercholesterolemia localizes to the gene coding for the enzyme proprotein convertase subtilisin/kexin type 9 (PCSK9). This enzyme modulates the activity of the LDLR. Gain-of-function mutations lead to increased degradation of the LDLR, whereas loss-of-function mutations allow increased expression of the LDLR; these mutations are associated with increased and decreased risk for CAD, respectively.
Low-Density Lipoprotein Cholesterol and Atherosclerotic Vascular Disease
Atherogenesis is a complex disease whose etiology and progression are strongly influenced by the severity of a large number of risk factors. LDL is an apo B–containing particle ( Fig. 14-1 ) and as such contributes to high levels of atherogenic lipoproteins ( Fig. 14-2 ). In addition, low serum levels of high-density lipoprotein cholesterol (HDL-C), hypertension, impairments in glucose metabolism, heightened systemic inflammatory tone, cigarette smoking, and other risk factors induce progressive arterial wall injury. An early event in atherogenesis is the development of endothelial cell dysfunction. Under normal physiologic conditions, the endothelium serves as an efficient barrier between blood and the arterial wall. The endothelium produces nitric oxide (a potent vasodilator) and maintains an antithrombogenic surface by producing prostacyclin and tissue plasminogen activator. As the endothelium becomes stressed in response to risk factors, it becomes dysfunctional, resulting in a maladaptive inflammatory response ( Fig. 14-3 ).



Endothelial dysfunction is highly associated with atherosclerosis. Dysfunctional endothelial cells increase expression of adhesion molecules, such as vascular cell adhesion molecule 1, intercellular adhesion molecule 1, and different types of selectins. These cell surface receptors interact with counterreceptors on the surface of inflammatory white blood cells, such as monocytes, T cells, and mast cells. These receptor-counterreceptor interactions facilitate the binding, rolling, and stable arrest of these cells along the endothelial surface.
Under normal conditions, endothelial cells maintain appropriate intercellular contacts through gap junctions, which allow these cells to communicate with one another and to maintain tight contacts among themselves. These gap junctions also become dysfunctional and allow the transmigration of inflammatory white cells into the subendothelial space by following a gradient of monocyte chemoattractant protein 1 down into the subendothelial space. Once they are in the subendothelial space, monocytes can transform into resident macrophages in response to macrophage colony-stimulating factor. As gap junctions and endothelial cells become more dysfunctional, their barrier function becomes progressively more compromised, allowing greater influx of atherogenic lipoproteins into the arterial wall. Lipoproteins can be trapped in the vessel wall by intercellular matrix proteins such as proteoglycans, fibronectin, and vitronectin, among others.
In the subendothelial space, LDL particles can be oxidized by such enzymes as myeloperoxidase, 5′-lipoxygenase, lipoprotein-associated phospholipase A 2 , NADPH oxidase, and others. T cells can present oxidized LDL to macrophages. The macrophages are activated and increase expression of families of scavenging receptors (e.g., CD36, scavenging receptor A). Scavenging receptors take up oxidized LDL, leading to foam cell formation. Foam cells can coalesce to form fatty streaks, and fatty streaks are the progenitors to the formation of atherosclerotic plaque. Macrophages can maintain a certain level of lipid homeostasis as long as there is adequate availability of HDL particles. HDL particles interact with macrophages and foam cells to promote the mobilization and externalization of intracellular lipid.
If there is inadequate availability of HDL in the face of excess LDL in the extracellular milieu, there is continued net accumulation of lipid by the macrophage, ultimately resulting in apoptosis or programmed cell death. As cellular debris and more lipid accumulate, and as the capacity to phagocytose and clear this debris is progressively more compromised, the volume of the atherosclerotic plaque increases and develops a lipid core ( Fig. 14-4 ).

National Cholesterol Education Program Guidelines
A high serum level of LDL-C is an established risk factor for the development of CHD. For this reason, LDL-C reduction is the primary goal of therapy in patients with dyslipidemia. This recommendation is emphasized by the National Cholesterol Education Program Adult Treatment Panel III (NCEP ATP III), the American Heart Association/American College of Cardiology guidelines for secondary prevention of CHD, and the European Guidelines on Cardiovascular Disease Prevention. NCEP ATP III defines an LDL-C of <100 mg/dL as optimal. Among patients in the primary prevention setting, it is recommended that 10-year projected risk for sustaining a CHD-related event be estimated with the Framingham risk score. Non–HDL-C is a surrogate measure of total atherogenic lipoprotein burden in serum and represents the sum of VLDL, IDL, LDL, and lipoprotein(a) as well as remnant particles. It is calculated by subtracting HDL-C from total cholesterol. Among patients with baseline triglycerides >200 mg/dL, non–HDL-C is the secondary target of therapy. Non–HDL-C has been shown to be a better predictor than LDL-C of risk for cardiovascular events in both men and women. Goals for both LDL-C and non–HDL-C are risk stratified, and the goals for both of these lipoprotein fractions decrease as risk increases ( Table 14-1 ). Among patients with CAD or a CAD risk equivalent (diabetes mellitus, abdominal aortic aneurysm, peripheral arterial disease, symptomatic carotid artery disease, or 10-year Framingham risk score >20%), it is recommended that patients achieve LDL-C <100 mg/dL and non–HDL-C <130 mg/dL. Among patients defined as very high risk (CAD complicated by a recent acute coronary syndrome, diabetes mellitus, or multiple poorly controlled risk factors), it is a therapeutic option to reduce LDL-C below 70 mg/dL and non–HDL-C below 100 mg/dL. In a more recent advisory statement from the American Heart Association, it was recommended that an LDL-C target of <70 mg/dL be an option for any patient with established CAD.
Risk Category 1 | LDL Goal | LDL Level at Which to Initiate TLC | LDL Level at Which to Consider Drug Therapy |
---|---|---|---|
CHD or CHD risk equivalents (10-year risk >20%) | <100 mg/dL (optional goal <70) 2 | ≥100 mg/dL All patients regardless of LDL | ≥130 mg/dL (100-129 mg/dL: drug optional) ≥100 mg/dL 3 (<100 mg/dL: drug optional) |
2+ risk factors (10-year risk 10%-20%) | <130 mg/dL (optional goal <100) | ≥130 mg/dL All patients regardless of LDL | ≥130 mg/dL (>100 mg/dL: drug optional 4 ) |
2+ risk factors (10-year risk ≤10%) | <130 mg/dL | ≥130 mg/dL | ≥160 mg/dL |
0-1 risk factor | <160 mg/dL | ≥160 mg/dL | ≥190 mg/dL (160-189 mg/dL: LDL-lowering drug optional) |
1 Risk factors included in Framingham risk evaluation are age, systolic blood pressure, total cholesterol, HDL-C, and smoking status.
2 The optional goal of <70 mg/dL is particularly targeted at patients who are “very high” risk (e.g., patients with a recent acute coronary syndrome, poorly controlled diabetics with multiple risk factors).
3 When statin therapy is initiated in these patients, the goal for LDL-C reduction should be 30% to 40% from baseline.
Evidence from clinical trials has established the merits of aggressive LDL-C risk reduction therapies for patients with CHD and other atherosclerotic vascular diseases in improving survival, reducing recurrent coronary events and need for revascularization, and improving quality of life. Data from studies including the Framingham Heart Study, Multiple Risk Factor Intervention Trial, and the Lipid Research Clinics trial established the direct relationship between levels of LDL-C and the rate of development of CHD in asymptomatic patients. Other studies including the Heart Protection Study (HPS), the Treating to New Targets (TNT) trial, and the Incremental Decrease in Endpoints through Aggressive Lipid Lowering (IDEAL) established the benefits of aggressive statin therapy in secondary prevention.
However, despite the substantial body of research establishing the benefit of LDL-C reduction, only a small percentage of patients with CHD are reaching lipid goals. In the National Cholesterol Education Program (NCEP) Evaluation Project Utilizing Novel E-Technology (NEPTUNE) survey of physicians regarding the care of 4885 patients with dyslipidemia, 75% of patients with CHD met the definition of “very high risk,” yet only 18% had an LDL-C level <70 mg/dL and only 4% had an LDL-C level <70 mg/dL and a non–HDL-C level <100 mg/dL when triglycerides were >200 mg/dL.
In a retrospective study from a national outpatient electronic medical record data base of 10,637 patients with a diagnosis of atherosclerosis, 57% of the 4067 patients with a baseline LDL-C ≥100 mg/dL were not prescribed statin treatment after diagnosis. Among patients receiving statin or any other cholesterol-lowering therapy after diagnosis who had baseline and follow-up LDL-C values (n = 682), 43% had a post-diagnosis LDL-C ≥100 mg/dL. The proportion of patients with LDL-C <100 mg/dL at baseline was 46.8%, and 12% of patients had LDL-C cholesterol <70 mg/dL. Less than 5% of patients were currently receiving hypercholesterolemia therapy at the time of diagnosis, and 25% had hypercholesterolemia treatment after their condition had been diagnosed. Among patients receiving hypercholesterolemia therapy, LDL-C levels in 12 months after diagnosis of atherosclerosis were similar to levels at the time of diagnosis. These data substantiate the need for more aggressive statin therapy and implementation of combination therapy to reduce residual risk for cardiovascular events and highlight the importance of monitoring and managing lipid levels in patients with atherosclerosis.
Metabolic Syndrome and Cardiometabolic Risk
In the United States, it is estimated that 35% to 40% of adults have the metabolic syndrome, a cluster of lipid and nonlipid disorders. The metabolic syndrome is characterized by a set of five risk factors: abdominal obesity; elevated blood pressure, triglycerides, and fasting glucose concentration; and low HDL-C ( Table 14-2 ). The presence of any three or more of the following risk factors in a single individual is diagnostic of the metabolic syndrome according to the revised ATP III/American Heart Association/National Heart, Lung, and Blood Institute definition : triglycerides ≥150 mg/dL, HDL-C <40 mg/dL in men and ≤50 mg/dL in women, serum glucose concentration ≥100 mg/dL, blood pressure ≥130/85 mm Hg (can be either systolic or diastolic or on therapy with antihypertensive medication), and waist circumference ≥40 inches in men and ≥35 inches in women. Metabolic syndrome develops secondary to the effects of insulin resistance and obesity. Although the metabolic syndrome significantly increases risk for atherosclerotic disease and diabetes mellitus, it is not defined as a CAD risk equivalent.
Risk Factor | Defining Level |
---|---|
Abdominal obesity | |
Men | Waist >40 inches |
Women | Waist >35 inches |
Triglycerides | ≥150 mg/dL |
HDL-C | |
Men | <40 mg/dL |
Women | <50 mg/dL |
Blood pressure | ≥130/≥85 mm Hg or taking antihypertensive medication |
Fasting glucose | ≥100 mg/dL or taking hypoglycemic medication |
* Patients having any three of the five risk factors meet criteria for the diagnosis of the metabolic syndrome.
The primary goal of clinical management of the metabolic syndrome is to reduce CHD risk and diabetes mellitus. This focuses on intensified LDL-C lowering and modification of the underlying risk factors including obesity, physical inactivity, and other risk factors associated with the metabolic syndrome, such as blood pressure control ( Table 14-3 ). The NCEP ATP III guidelines emphasize the modification of metabolic syndrome risk factors through lifestyle changes, especially because both weight loss and exercise reduce insulin resistance and favorably modify risk factors for the metabolic syndrome.
Dietary Component | Recommendation Allowance |
---|---|
Polyunsaturated fat | Up to 10% of total calories |
Monounsaturated fat | Up to 20% of total calories |
Total fat | 25%-35% of total calories |
Carbohydrate | 50%-60% of total calories |
Dietary fiber | 20-30 g/day |
Protein | Approximately 15% of total calories |
Dietary cholesterol | <200 mg/day |
Therapeutic Lifestyle Changes
A number of preventive strategies can be used to target reductions in LDL-C to decrease the burden of CHD. The NCEP ATP III guidelines identify therapeutic lifestyle changes (TLC) as the initial intervention for lowering of LDL-C with a focus on smoking cessation, weight loss, total calories, physical activity to maintain desirable weight, and moderate alcohol intake (see Table 14-3 ). The NCEP guidelines outline TLC as a multifaceted lifestyle approach for LDL-C lowering. The recommendations for TLC include a reduction in saturated fat intake to <7% of total calories and cholesterol to <200 mg/day, weight reduction, increased physical activity, therapeutic options to enhance LDL lowering such as the use of plant stanols or sterols (2 g/day), and increased viscous (soluble) fiber (10 to 25 g/day) to reduce cholesterol absorption. The aim of primary prevention is to reduce long-term risk (>10 years) as well as short-term risk (≤10 years). Whereas TLC is the basis of clinical primary prevention, pharmacologic therapy is often indicated in the management of elevated LDL-C to achieve risk-stratified target goals.
Statin Therapy
Statins, or 3-hydroxy-3-methylglutaryl-coenzyme A reductase inhibitors, have been the most widely used therapy for the treatment of dyslipidemia to reduce the risk of CHD. Statins are used to target the reduction of elevated LDL-C and to improve the lipid level profile. The statins are recognized as the first-line treatment of dyslipidemia. Statins are used to target the reduction of elevated LDL-C and to improve all components of the lipid level profile.
Data from the major statin trials including the Scandinavian Simvastatin Survival Study (4S), Cholesterol and Recurrent Events (CARE), HPS, Long-term Intervention with Pravastatin in Ischemic Disease (LIPID), and TNT have established that LDL-C reduction correlates with cardiovascular event reduction in a linear fashion. The HPS, the largest statin trial, demonstrated the benefits of aggressive statin therapy with simvastatin 40 mg/day, with a 24% reduction in CVD events compared with placebo ( Table 14-4 ). The beneficial effects of LDL-C reduction were demonstrated even in patients with baseline LDL-C levels below 100 mg/dL and in persons with diabetes.
Primary Prevention Studies | |||
Study | Drug | Design | Outcomes |
AFCAPS/TexCAPS | Lovastatin 20 to 40 mg/day versus placebo | 6605 men and women | 40% reduction in fatal and nonfatal MI; 37% reduction in first ACS; 33% reduction in coronary revascularizations; and unstable angina reduced by 32% |
ASCOT | Atorvastatin 10 mg/day versus placebo | 10,305 hypertensive men (n = 8463) and women (n = 1942) with treated high blood pressure and no previous CAD | 36% reduction in total CHD/nonfatal MI; 27% reduction in fatal and nonfatal stroke; total coronary event reduced by 29%; fatal and nonfatal stroke reduced by 27% |
CARDS | Atorvastatin 10 mg/day versus placebo | 2838 patients with type 2 diabetes mellitus and 1 CHD risk factor | 37% reduction of major cardiovascular events; 27% reduction of total mortality; 13.4% reduction of acute cardiovascular events; 36% reduction of acute coronary events; 48% reduction of stroke |
Heart Protection Study | Simvastatin 40 mg/day versus placebo | 20,536 high-risk individuals (previous CHD, other vascular disease, hypertension among men aged >65 years, or diabetes) | 25% reduction in all-cause and coronary death rates and in strokes; need for revascularization reduced by 24%; fatal and nonfatal stroke reduced by 25%; nonfatal MI reduced by 38%; coronary mortality reduced by 18%; all-cause mortality reduced by 13%; cardiovascular event rate reduced by 24% |
PROSPER | Pravastatin 40 mg/day versus placebo | 5804 men (n = 2804) and women (n = 3000) aged 70 to 82 years | 15% reduction in combined endpoint (fatal/nonfatal MI or stroke); 19% reduction in total/nonfatal CHD; no effect on stroke (but 25% reduction in TIA) |
WOSCOPS | Pravachol therapy 40 mg/day versus placebo | 6595 men | CHD death of nonfatal MI reduced by 31%; CVD death reduced by 32%; total mortality 22% reduction |
Secondary Prevention Studies | |||
Study | Drug | Design | Outcomes |
4S | Simvastatin 20 mg/day versus placebo | 4444 patients with angina pectoris or history of MI | Coronary mortality reduced by 42%; myocardial revascularization reduction of 37%; all-cause mortality reduced by 30%; nonfatal major coronary event reduced by 34%; fatal and nonfatal stroke reduced by 30% |
AVERT | Atorvastatin 80 mg/day versus angioplasty + usual care | 341 patients with stable CAD | 36% reduction in ischemic event; delayed time to first ischemic event reduced by 36% |
CARE | Pravastatin 40 mg/day versus placebo | 3583 men and 576 women with history of MI | Death from CHD or nonfatal MI reduced by 24%; death from CHD reduced by 20%; nonfatal MI reduced by 23%; fatal MI reduced by 37%; CABG or PTCA reduced by 27% |
IDEAL | Atorvastatin 80 mg/day versus simvastatin 20-40 mg/day | 8888 men and women with CHD | Major cardiac events reduced by 13%; nonfatal MI reduced by 17%; revascularization reduced by 23%; peripheral arterial disease reduced by 24% |
JUPITER | Rosuvastatin 20 mg/day versus placebo | 17,802 men (>50 years) and women (>60 years) with no history of CAD or diabetes mellitus, entry LDL <130 mg/dL, and CRP >2.0 mg/L | 44% reduction in primary endpoint of major coronary events; 65% reduction in nonfatal MI; 48% reduction in nonfatal stroke; 46% reduction in need for revascularization; 20% reduction in all-cause mortality |
LIPID | Pravachol 40 mg/day versus placebo | 9014 patients | Coronary mortality reduced by 24%; stroke reduced by 19%; fatal CHD or nonfatal MI reduced by 24%; fatal or nonfatal MI reduced by 29% |
LIPS | Fluvastatin 40 mg/day versus placebo | 1667 men and women aged 18-80 years after angioplasty for CAD | 22% lower rate of major coronary events (e.g., cardiac deaths, nonfatal MI, or reintervention procedure) |
MIRACL | Atorvastatin 80 mg/day versus placebo | 3086 patients with ACS | Reduction in composite endpoint by 16%; ischemia reduced by 26%; stroke reduced by 50% |
PROVE IT | Atorvastatin 80 mg/day versus pravastatin 40 mg/day | 4162 patient with ACS | 16% reduction of composite endpoint; 14% reduction in CHD death, MI, or revascularization; revascularizations reduced by 14%; unstable angina reduced by 29% |
REVERSAL | Atorvastatin 80 mg/day versus pravastatin 40 mg/day | 654 patients with CAD | Atheroma: atorvastatin −0.4%, pravastatin 2.7%, difference of −3.1%, P = 0.02 |
TNT | Atorvastatin 10 mg/day versus 80 mg/day | 10,003 patients with CHD and LDL cholesterol 130-250 mg/dL | 22% reduction in composite endpoint; MI reduced by 22%; stroke reduced by 25% |
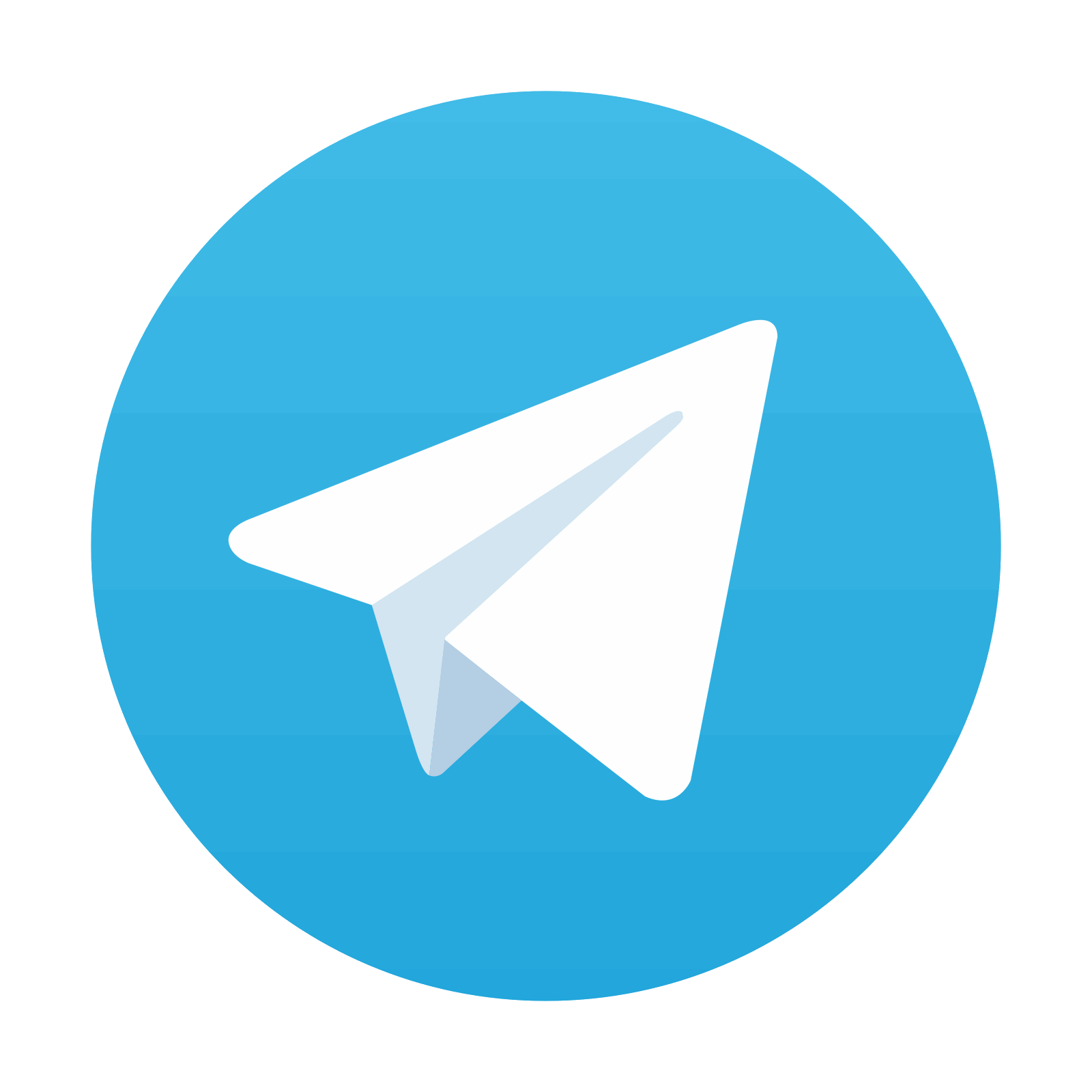
Stay updated, free articles. Join our Telegram channel

Full access? Get Clinical Tree
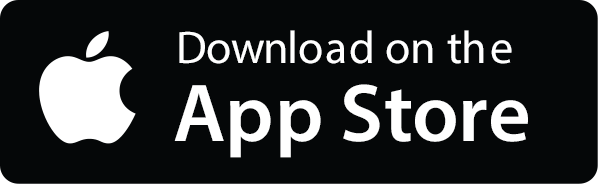
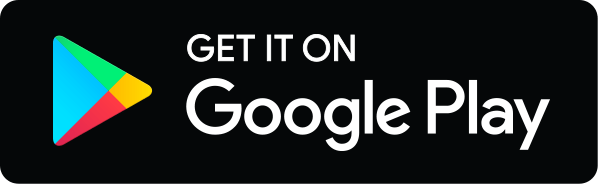
