A. Polyenes
Amphotericin B (AmB) and its various formulations, deoxycholate AmB (D-AmB), AmB colloidal dispersion (ABCD), AmB lipid complex (ABLC), and liposomal AmB (L-AmB), belong to the class of polyene antifungals. For many years, AmB was the “gold standard” and has proven to be a reliable antifungal agent, but a toxic compound. However, details regarding its pharmacokinetics, especially the impact of either renal or hepatic organ dysfunction on its pharmacokinetics, are not available (Lepak and Andes 2011). Thus, whereas the dose-limiting toxicity is predominantly nephrotoxicity, dose reductions are unlikely to produce sufficient infection site concentrations (Lepak and Andes 2011). Among the lipid formulations there are marked differences in kinetic profiles (Groll et al. 2000a, 2006; Lewis et al. 2007). Lewis et al. compared the pharmacokinetics of AmB lung accumulation and fungal clearance in ABLC- and L-AmB-treated mice; both formulations were effective in reducing the fungal burden at a dose of ≥5 mg/kg/day. Though ABLC produced a more rapid antifungal clearance than L-AmB at 5 mg/kg/day, there was no difference at a higher dose of 10 mg/kg/day (Groll et al. 2006). Within the cerebrospinal fluid (CSF), the concentrations of all four polyenes were remarkable similar. L-AmB achieved 6–10 times higher brain tissue concentrations than all other AmB formulations (Groll et al. 2000a). In another study, the distributions of D-AmB, ABCD, ABLC, and L-AmB were investigated. At a dose of 5 mg/kg/day all AmB lipid formulations achieved at least fourfold higher concentrations in the bone marrow than conventional AmB at a dose of 1 mg/kg/day. In contrast, all AmB formulations accumulated relatively poorly within fat tissue, with L-AmB achieving the highest absolute concentration (Groll et al. 2000b).
B. Echinocandins
Echinocandins are also only available for intravenous administration. They all share a low oral bioavailability, high protein binding, and extensive distribution into most tissues including liver, spleen, lungs, and kidneys (Eschenauer et al. 2007; Dowell et al. 2007; Hebert et al. 2005; Goicoechea et al. 2004; Carver 2004; Pfizer 2006; Merck 2001; Astellas 2005). However, due to their high protein binding and large molecular weights, they only exhibit low penetration into urine, CSF, brain, and ocular fluid (Vazquez and Sobel 2006; Deresinski and Stevens 2003; Chandrasekar and Sobel 2006; Okugawa et al. 2007). The pharmacokinetics of all three echinocandins are linear, but whereas caspofungin and micafungin are mainly degraded in the liver by hydrolysis and N-acetylation (Eschenauer et al. 2007), anidulafungin undergoes chemical degradation within the plasma and not within the liver (Pfizer 2006). The mainly inactive metabolites of the echinocandins are then slowly excreted over many days, primarily via the bile. Echinocandins are usually administered once daily and have half-lives ranging from 9 to 26 h, i.e., 9–11 h for caspofungin, 11–17 h for micafungin, and 24–26 h for anidulafungin (Astellas 2005; Merck 2001; Pfizer 2006).
C. Triazoles
Among the currently used triazole antifungals, fluconazole is the only drug that is soluble in water without the need for a solvent. Fluconazole is very well absorbed from the gastrointestinal tract and it exhibits very low protein binding and good distribution in all kinds of tissues, including ocular and central nervous system (CNS) sites (Brammer et al. 1990; O’Day et al. 1990; Savani et al. 1987; Mian et al. 1998; Arndt et al. 1988; Foulds et al. 1988). In the CSF, the fluconazole concentrations reach ~70% of the serum concentration. The pharmacokinetics of fluconazole are linear over dosages ranging from 50 to 800 mg/day (McLachlan and Tett 1996; Anaissie et al. 1995). Most of the drug (~80%) is excreted unchanged via the urine; hence, the hepatic CYP2C9 enzyme only plays a minor role in its metabolism. Fluconazole dosage reduction is recommended in cases of marked renal dysfunction (Pfizer 1990).
Voriconazole is available as an oral and as a sulfobutylether-β-cyclodextrin intravenous formulation (Pfizer 2002). Despite its structural similarity to fluconazole it is only poorly soluble in water. The oral bioavailability of voriconazole as tablet or solution is 96%; however, its absorption may be decreased by 22% by the concomitant intake of food. Voriconazole disseminates into most infection sites, including the CNS and eye (Lutsar et al. 2003; Hariprasad et al. 2004). It is metabolized in the liver, mainly by CYP2C19, CYP3A4, and to a lesser extent by CYP2C9; hence, the genotype status for CYP2C19 and/or co-administration of drugs that modulate CYP2C19 or CYP3A4 activities may affect voriconazole plasma levels (Hyland et al. 2003).
The effect of CYP2C19 polymorphism on the pharmacokinetics of voriconazole was studied for healthy CYP2C19 homozygous extensive metabolizers (EMs), heterozygous extensive metabolizers (HEMs), and poor metabolizers (PMs) (Lee et al. 2012). The steady state trough concentrations and AUCs were five and three times higher for PMs than for EMs, respectively. Thus, the CYP2C19 polymorphism is a major covariate and accountable for some of the wide pharmacokinetic variability of voriconazole (Lee et al. 2012). But still, the total variability of voriconazole plasma concentrations is only partly accounted for by sex, age, and CYP2C19 genotype (FDA 2001).
Although voriconazole is usually well tolerated (Walsh et al. 2002; Ally et al. 2001), high concentrations may be associated with visual changes and hepatotoxicity, which mainly manifests by elevation of AST/ALT (Tan et al. 2006). Congruently, various studies have shown that therapeutic drug monitoring of trough concentrations is important as a guide to therapy (Smith et al. 2006; Pascual et al. 2008; Denning et al. 2002). Because the sulfobutylether-β-cyclodextrin, which is used as solvent for the intravenous formulation, may accumulate in patients with impaired renal function extended use of this formulation should be avoided in patients with moderate to severe renal dysfunction, defined as a creatinine clearance (CrCl) below 50 ml/min or a creatinine concentration above 2.5 mg/dl (FDA 2001).
Itraconazole is another member of the triazole antifungal class. Like voriconazole, it is poorly soluble in water at physiological pH, hence its liquid formulations, i.e., the liquid oral (LO) and intravenous formulations, contain cyclodextrin as a solubilizing agent (Janssen 1999). Similar to the cyclodextrin-containing parenteral formulation of voriconazole, the parenteral formulations of itraconazole are indicated in patients with severe renal impairment (defined as CrCl below 30 ml/min) only if the benefit outweighs the risk (Janssen 1999). In patients with mild (defined as CrCl 50–80 ml/min) and moderate (defined as CrCl 30–49 ml/min) renal impairment, parenteral formulations should be used with caution, while monitoring serum creatinine concentrations (Janssen 1999). The pharmacokinetic parameters of the drug are not markedly affected by renal or hepatic dysfunction. Capsules containing itraconazole (i.e., solid oral formulations) do not require any solvent; however, its absorption is influenced by the gastric acidity and significantly varies with the intake of food or acidic beverages (Barone et al. 1993; Jaruratanasirikul and Kleepkaew 1997). The absorption of the LO formulation is not affected by the gastric acidity and, in contrast to the solid oral formulation, is best absorbed without a meal (Janssen 1999; Johnson et al. 2003; Barone et al. 1998; Van de Velde et al. 1996). One study compared the relative bioavailability of the solid oral and LO formulation in adult cystic fibrosis patients; relative bioavailability for itraconazole capsules was 82% compared with the solution (Hennig et al. 2007). Nausea and diarrhea is more common with the LO formulation, possibly due to the osmotic effects of the cyclodextrin. The distribution of itraconazole into most infection sites, except the CNS and eye, is good (Janssen 1999; Savani et al. 1987). The pharmacokinetics of itraconazole have been described using nonlinear (Hardin et al. 1988) and linear concentration–time profile models (Hardin et al. 1988; Hennig et al. 2006, 2007; Koks et al. 2003). In healthy volunteers, the terminal half-life was 24 h, and the steady state was reached after 13–14 days (Hardin et al. 1988). Itraconazole is mainly metabolized within the liver and/or the intestine, facilitating the CYP3A4 pathway (Poirier and Cheymol 1998). The first step consists of the formation of hydroxy-itraconazole (OH-itraconazole), which, unlike metabolites of other azoles, exhibits similar antifungal activity to the native drug (Odds and Bossche 2000). Later on, this metabolite is converted to the inactive keto-itraconazole and N-dealkyl-itraconazole (ND-itraconazole). Both active compounds (i.e., itraconazole and OH-itraconazole) usually circulate at comparable concentrations, whereas the concentrations of keto-itraconazole and ND-itraconazole are significantly lower (Templeton et al. 2008). Itraconazole is predicted to partially saturate CYP3A4, but its metabolites, and ND-itraconazole in particular, also contribute to inhibition of CYP3A4-mediated metabolism (Templeton et al. 2008). Because the half-life of ND-itraconazole appears to be longer it might be responsible for the clinically observed persistent inhibition of CYP3A4-mediated clearance of immunosuppressants co-administered with itraconazole (Templeton et al. 2008; Banerjee et al. 2001; Kwan et al. 1987).
Posaconazole is currently only available as an oral solution (Schering 2006), but solid oral and intravenous formulations are in development (Merck 2010; Merck 2013). Posaconazole displays linear pharmacokinetics between 50 and 800 mg, with saturation of absorption occurring above 800 mg/day (Courtney et al. 2003). The terminal half-life ranges from 25 to 31 h, supporting once- or twice-daily dosing (Courtney et al. 2003). However, divided daily doses (every 12 or 6 h) significantly increases the posaconazole exposure in healthy adults under fasting conditions (Ezzet et al. 2005), while administration with food also increases the bioavailability (Courtney et al. 2004). The relative oral bioavailability of posaconazole significantly depends on the prandial state. When posaconazole is administered with a high-fat or non-fat meal, its mean AUC (0, 72 h) and maximum concentrations (Cmax) are increased fourfold and 2.6- to 3.0-fold, respectively, compared with administration in the fasting state (Courtney et al. 2004). Still, the pharmacokinetics of posaconazole are not very predictable and subject to huge inter-individual variability (Smith et al. 2009). The elimination of posaconazole is increased in patients with diarrhea (Kohl et al. 2010). However, despite the relatively frequent occurrence of low posaconazole serum concentrations, no cut-off for its prophylactic efficacy has yet been established. Therefore, it is currently unclear whether therapeutic drug monitoring (TDM) may increase its clinical efficacy (Kohl et al. 2010; Hope et al. 2008a). Posaconazole is mainly metabolized by hepatic glucuronidation, but not by the CYP450 system enzymes; however, it is a moderate inhibitor of CYP3A4 (Wexler et al. 2004). The drug is primarily excreted via the bile and the urine as unchanged drug or inactive metabolites.
Common to all systemic triazole antifungals is that they are inhibitors of cytochrome P450 (CYP) isozymes, such as CYP3A4, CYP2C9, and CYP2C19, to varying degrees. Whereas fluconazole and voriconazole inhibit CYP2C9, CYP2C19, and CYP3A4; itraconazole and posaconazole only inhibit CYP3A4. Some triazoles are substrates (fluconazole, itraconazole, and posaconazole) and/or inhibitors (itraconazole) of drug transporters such as the multidrug resistance-1 gene product, P-glycoprotein (Nivoix et al. 2008). A detailed review of this important topic has been published by Nivoix et al. (2008).
III. Pharmacodynamics of Common Antifungal Drugs
Different indices are commonly used to measure or describe the relationship between the pharmacokinetic parameters of a drug and the MIC of a pathogen. Various studies have been undertaken to address the question of which index best describes the activity of a certain antifungal drug against different pathogens in order to predict treatment outcomes. For AmB it was shown that the Cmax:MIC is closely linked to treatment efficacy, with drug concentrations exceeding the MIC two- to tenfold (Andes et al. 2001; Lewis et al. 2005; Wiederhold et al. 2006).
In a neutropenic mouse model of disseminated candidiasis, AmB efficacy correlated with the percentage of time above MIC (%t > MIC), AUC:MIC, and Cmax:MIC (Andes et al. 2001). The best predictive index to determine the treatment outcome was Cmax:MIC, whereas the AUC:MIC and %t > MIC were only slightly less predictive (Andes et al. 2001). For filamentous fungi, a species-specific concentration-dependent activity of AmB (Lewis et al. 2005) and a close correlation between increased survival and Cmax:MIC ratio was shown (Wiederhold et al. 2006). For the echinocandins, similar results regarding their activity against Candida spp. have been shown, with a maximum efficacy at free-drug AUC:MIC ratios close to 10 (Ernst et al. 1999, 2002a; Andes et al. 2008a, b, 2003a; Andes and Safdar 2005; Gumbo et al. 2006, 2007; Groll et al. 2001; Hope et al. 2008b; Clancy et al. 2006; Walsh et al. 1991). In a murine model of invasive pulmonary aspergillosis, a Cmax:MEC ratio for caspofungin appeared to be the parameter most closely associated with the reduction of pulmonary fungal burden (Wiederhold et al. 2004). However, increasing drug concentration of echinocandins above the organism’s MIC may result in a paradoxical increase of in vitro fungal growth (Pound et al. 2010). For the triazole antifungals, exposure–response relationships are well studied, with concentrations one to two times above the MIC showing optimal time–dependent (t > MIC) antifungal activity (Turnidge et al. 1994; Ernst et al. 2000, 2002b; Andes and van Ogtrop 1999; Andes et al. 2003b, c, 2004, 2006; Ernst et al. 1998; Warn et al. 2009; Louie et al. 1998). A study on the pharmacodynamics of fluconazole in patients with candidemia showed that AUC:MIC ratios below 11.5 were associated with an increased mortality (Baddley et al. 2008). For multiple Candida isolates, efficacy was observed with free-drug 24 h AUC:MIC ratios near 25 (Lepak and Andes 2011). The in vivo efficacy of posaconazole against four clinical Aspergillus fumigatus isolates, with posaconazole MICs ranging from 0.03 to 16 mg/l, was assessed in a non-neutropenic murine model of disseminated aspergillosis. Near-maximum and 50% survival were reached at AUC:MIC ratios of nearly 1,000 (corresponding to a free-drug AUC:MIC ratio of 10) and 321.3, respectively (Mavridou et al. 2010). Similar results were observed using a murine inhalational model of invasive pulmonary aspergillosis, with a dose-dependent decline in serum galactomannan concentrations. AUC:MIC ratios of 89.88, 166.90, 308.51, and 440.91 resulted in 20%, 50%, 80%, and 90% of the maximum antifungal effect, respectively (Howard et al. 2011).
Transparency Declarations
FF received travel grants from Astellas and Schering-Plough. OAC is supported by the German Federal Ministry of Research and Education (BMBF grant 01KN1106) and has received research grants from, is an advisor to, or received lecture honoraria from 3 M, Actelion, Astellas, Basilea, Bayer, Biocryst, Celgene, Cubist, F2G, Genzyme, Gilead, GSK, Merck/Schering, Miltenyi, Optimer, Pfizer, Quintiles, Sanofi Pasteur, Viropharma.
References
Ally R, Schurmann D, Kreisel W, Carosi G, Aguirrebengoa K, Dupont B, Hodges M, Troke P, Romero AJ (2001) A randomized, double-blind, double-dummy, multicenter trial of voriconazole and fluconazole in the treatment of esophageal candidiasis in immunocompromised patients. Clin Infect Dis 33:1447–1454PubMedCrossRef
Andes D, van Ogtrop M (1999) Characterization and quantitation of the pharmacodynamics of fluconazole in a neutropenic murine disseminated candidiasis infection model. Antimicrob Agents Chemother 43:2116–2120PubMed
Andes D, Forrest A, Lepak A, Nett J, Marchillo K, Lincoln L (2006) Impact of antimicrobial dosing regimen on evolution of drug resistance in vivo: fluconazole and Candida albicans. Antimicrob Agents Chemother 50:2374–2383PubMedCrossRef
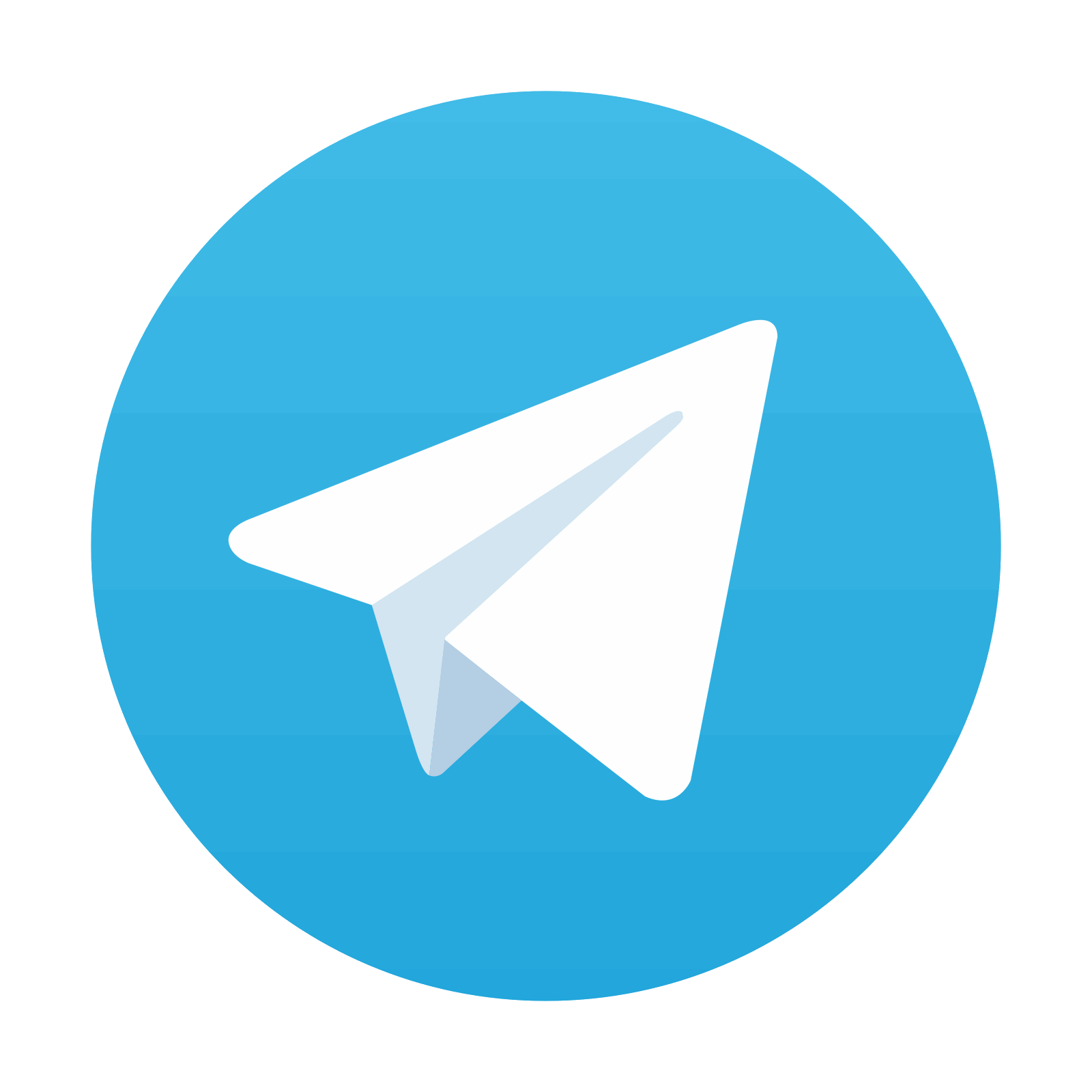
Stay updated, free articles. Join our Telegram channel

Full access? Get Clinical Tree
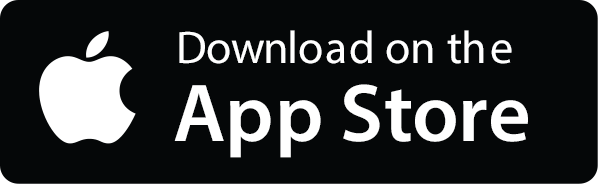
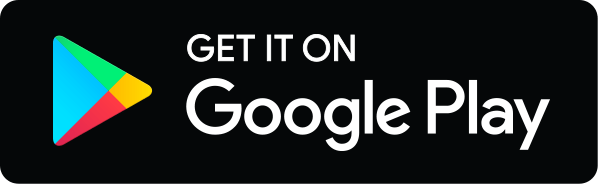