Fig. 21.1
Mechanism of action of unfractionated versus low-molecular-weight heparins (see text)
Warfarin exerts an anticoagulant effect by interfering with an essential posttranslational, vitamin K-dependent step that results in the gamma-carboxylation of specific glutamic acid residues in coagulation factors II, VII, IX, and X [1]. The resulting proteins exhibit dysfunctional calcium-dependent binding onto anionic phospholipid surfaces, interfering with rapid generation of thrombin. Warfarin and other vitamin K antagonists (e.g., dicoumarol, phenprocoumon, acenocoumarol) cause this effect by interfering with the cyclic conversion of vitamin K and its 2,3-epoxide, which blocks the regeneration of the reduced form of vitamin K1. Since the anticoagulant effect of warfarin is dependent on the synthesis of new, dysfunctional coagulation proteins, the antithrombotic efficacy of the drug is determined by the circulating half-life of the functional hemostatic proteins. The half-life of factor X is approximately 48 h and that of factor II is approximately 72 h; consequently, UFH or LMWH is used initially in the treatment of a patient with a new venous thromboembolism (VTE), generally for about 5 days, or until the anticoagulant effect of warfarin has sufficiently impacted the hemostatic proteins to manifest an antithrombotic effect.
21.2 Laboratory Monitoring of Anticoagulant Agents
For both UFH and the vitamin K antagonists, the therapeutic efficacy of the different agents has been shown to be optimal when a specific degree of anticoagulation, as measured by routine coagulation assays, has been achieved. For therapeutic UFH, the degree of anticoagulation is monitored by the activated partial thromboplastin time (aPTT), an assay that measures the intrinsic pathway of coagulation (Fig. 21.2). A subtherapeutic aPTT is associated with an increased risk of recurrent or progressive venous thromboembolism, whereas a supratherapeutic aPTT is associated with an increased risk of hemorrhage. Similarly, warfarin and the other vitamin K antagonists are also monitored for therapeutic efficacy, but in this case the test used is the prothrombin time (PT), an assay that measures the extrinsic pathway of coagulation (Fig. 21.2). To correct for variations in assay results due to differences in thromboplastin reagents and coagulometers, the PT is first converted to a ratio (patient PT by the mean of a normal range of PT values) that is then corrected with an adjustment factor to the international normalized ratio, or “INR.” A target INR of 2–3 is the typical target range for treatment of patients with venous thromboembolism. As with UFH, subtherapeutic warfarin is associated with an increased risk for venous thromboembolism, whereas supratherapeutic warfarin is associated with an increased risk for hemorrhage.
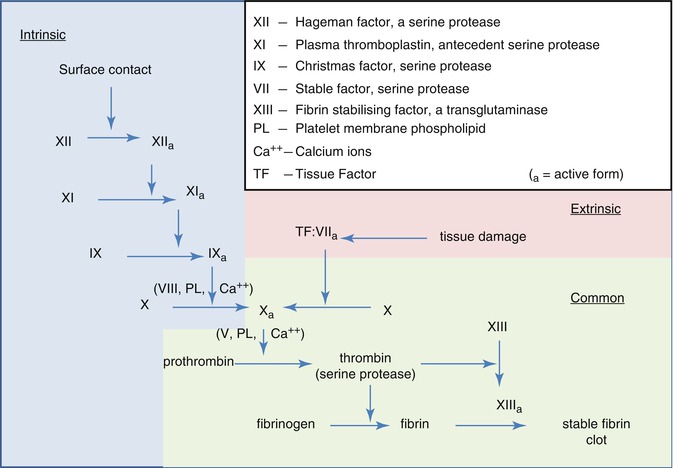
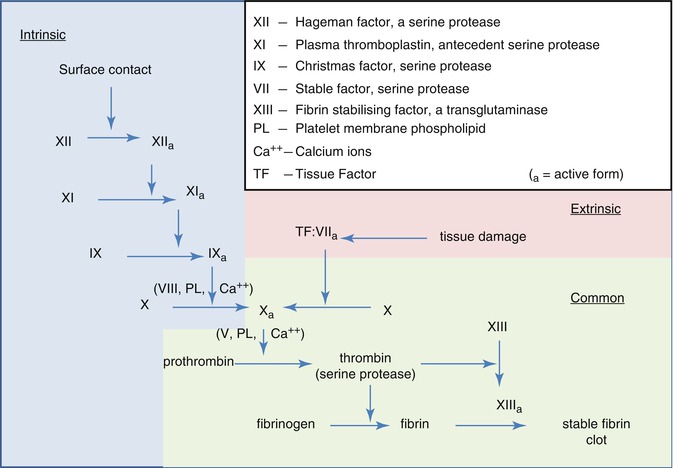
Fig. 21.2
Coagulation pathways (© Graham Colm, used with permission under the Creative Commons Atribution license)
The LMWH, although prepared from UFH, and with a similar, antithrombin-dependent, mechanism of action, does not need to be monitored for antithrombotic efficacy. These agents, typically administered subcutaneously with a weight-based dosage, exhibit a reproducible anticoagulant effect that does not require laboratory monitoring. They are cleared by the kidney, however, and can accumulate in patients with renal insufficiency. In this setting, checking anticoagulant efficacy can be performed using an anti-factor Xa assay.
21.3 Management Strategies
Patients with a new venous thromboembolism will be started on anticoagulation with UFH or LMWH concomitantly with a vitamin K antagonist, and the parenteral agent will be continued until the vitamin K antagonist is therapeutic as determined by the INR, at which time it will be stopped. Warfarin will then be continued for a minimum of 3 months of therapy, with periodic reassessment of the INR to confirm that the drug is still having the desired anticoagulant effect on hemostasis in the individual patient. Importantly, neither the UFH/LMWH nor the vitamin K antagonists actively dissolves the thrombus, but they prevent the thrombus from extending while the body’s endogenous fibrinolytic pathway works to repair the occluded vessels.
Multiple drugs, diet, and various disease states can influence the pharmacokinetic properties of warfarin. The impact of diet is primarily through variations in vitamin K intake, but a vitamin K intake that is too low can actually lead to increased variation in the INR [2]. INR monitoring typically needs to be more frequent with medication or diet changes, and with certain illnesses. In addition to various acquired conditions, allelic variants have been identified in the genes encoding vitamin K epoxide reductase (VKORC1) and in the cytochrome P450 2C9 hepatic microsomal enzyme (CYP2C9) that modify the dose of warfarin necessary to achieve and maintain a target therapeutic INR [3, 4]. Dosing algorithms have been developed that incorporate the results of polymorphisms at CYP2C9 and VKOR1, but implementation of these algorithms more widely is dependent on completion of prospective randomized clinical trials with clinical outcome data.
Multiple studies have demonstrated that the optimal approach to therapeutic monitoring of the INR is through a dedicated anticoagulation management service rather than through routine medical care. Frequently, these anticoagulation management services will use a point-of-care monitor to measure the PT from a finger-stick sample of capillary whole blood to provide rapid access to necessary data for assessing the anticoagulant effect of the vitamin K antagonist. A natural extension following the development of these meters was for patients to have access to their own meter for self-testing, and the first reports using this approach appeared in the late 1980s [5, 6]. Since these initial reports, several randomized controlled clinical trials have confirmed that strategies incorporating patient self-testing can be reliably used to manage a patient’s oral anticoagulant therapy [7]. More recently, Matchar and colleagues used an interactive voice-response reporting system across the telephone with Web-based local monitoring of INR results, comparing this strategy to monthly monitoring in an anticoagulation clinic (THINRS study) [8]. They found a slight improvement in the time in the target therapeutic INR range, but there was no improvement in clinical outcomes. In a more novel approach, Ryan and colleagues demonstrated that an Internet-based, direct-to-patient expert system could be used to effectively manage patients using INR self-testing with home monitors [9].
21.4 Complications of Anticoagulant Therapy
Hemorrhagic complications are the most frequent adverse event associated with the use of vitamin K antagonist therapy. Variables associated with an increased risk for therapy-related bleeding include the intensity of the anticoagulant effect (i.e., the target INR), the amount of time within the target INR (time in therapeutic range [TTR]), patient comorbidities, the concomitant use of drugs that interfere with hemostasis (e.g., antiplatelet agents), and the duration of therapy [1, 10]. The management of patients with elevated INR results is determined by the extent of the elevation of the INR, as well as whether or not the patient is having major bleeding (Table21.1) [1]. Recently, Majeed and colleagues described 160 patients treated with prothrombin complex concentrates for emergency reversal of warfarin either for bleeding or the need for emergent surgery, demonstrating good clinical efficacy in more than 90 % of patients treated [11]. Thromboembolic complications were observed, but the frequency was relatively low (six patients, 3.8 %) [11]. Recombinant factor VIIa can also be used in the treatment of patients with hemorrhagic complications related to anticoagulant therapy, but its use in non-approved indications has been associated with an increased risk for thromboembolic complications [12]. The use of these agents in this setting needs to be individualized and managed by a clinician experienced in their use.
Table 21.1
Inherited thrombophilic disorders
Disorder | Prevalence in normals (%) | Frequency in patients with VTE (%) | Relative risk of first episode of DVT |
---|---|---|---|
Factor V Leiden (heterozygote) | 0.05–4.8a | 18.8 | 7 |
Factor V Leiden (homozygote) | 0.02 | 1.5 | 80 |
Prothrombin G20210A allele | 0.06–2.7a | 7.1 | 2.8 |
Protein C deficiency | 0.2–0.4 | 3.7 | 6.5 |
Protein S deficiency | 0.16–0.21 | 2.3 | 5.0 |
Antithrombin deficiency | 0.02 | 1.9 | 20 |
Dysfibrinogenemia | <0.01 | 0.8 | Unknown |
Elevated homocysteine levelsb | 5–7 | 10 | 2.95 |
Elevated factor VIII level | 11 | 25 | 4.8 |
Elevated factor IX level | 10 | 20 | 2.8 |
Elevated factor XI level | 10 | 19 | 2.2 |
Elevated lipoprotein(a) level | 7 | 20 | 3.2 |
Elevated thrombin-activatable fibrinolysis inhibitor (TAFI) | 9 | 14 | 1.7 |
21.5 The Future of Vitamin K Antagonists
During the past decade, multiple new oral anticoagulants have been developed that do not require frequent laboratory monitoring or dose adjustments. These agents are not without their limitations, however, which are discussed further below. In addition, warfarin is one of the least expensive drugs, particularly when compared to other anticoagulant therapies. Although its management does incur significant health costs, these frequently involve different sources of funding. Consequently, certain patient populations are likely to continue use of warfarin and other vitamin K antagonists for some time, including patients with very stable INRs, patients with mechanical heart valves, and patients with limited financial resources.
21.6 Thrombolytic Therapy
In contrast to the passive reduction of thrombus size allowed by anticoagulant therapy, thrombolytic agents actively promote lysis of fibrin clot [13]. Although this approach is associated with an increased risk for bleeding, thrombolytic therapy should be considered in selected subsets of patients with venous thromboembolic disease [14]. Patients with extensive proximal deep venous thrombosis (DVT) (e.g., iliofemoral DVT) are at highest risk for postthrombotic morbidity, with up to 75 % having chronic painful edema and 40 % having venous claudication when treated with anticoagulant therapy alone (refs). Pharmacomechanical thrombolysis or catheter-directed thrombolysis, without mechanical thrombus fragmentation, would be the optimal approach in patients at low risk for hemorrhagic complications [14]. The ATTRACT (Acute Venous Thrombosis: Thrombus Removal with Adjunctive Catheter-Directed Thrombolysis) trial is a National Heart, Lung and Blood Institute (NHLBI)-sponsored prospective randomized trial that is comparing the use of adjunctive pharmacomechanical catheter-directed thrombolysis to optimal standard DVT therapy alone in patients with acute, symptomatic DVT (clinicaltrials.gov #NCT00790335). Importantly, this study will determine the development of postthrombotic syndrome to assess the development of this complication in patients treated with a more aggressive approach. For programs that lack expertise in interventional vascular procedures, systemic thrombolysis may be used in selected patients with acute DVT15, or the patient can be transferred to a center with the appropriate level of expertise [14].
Patients with pulmonary embolism (PE) should undergo risk stratification to evaluate for the role of thrombolytic therapy. For patients with massive acute pulmonary embolism, defined as acute PE with sustained hypotension, thrombolytic therapy should be considered unless there are contraindications owing to hemorrhagic risk [14]. Fibrinolysis may also be considered for patients with submassive acute PE who have additional clinical evidence of adverse prognosis and a low risk for hemorrhagic complications [14]. In contrast, patients with a low-risk PE, or submassive acute PE with minor right ventricular dysfunction or minor myocardial necrosis, should be treated with anticoagulant therapy and not fibrinolysis [14, 15]. Intravenous therapy via a peripheral vein is indicated in most patients who will be treated with thrombolysis [15].
21.6.1 Inferior Vena Cava Filter
The placement of a filter in the inferior vena cava (IVC) represents a strategy whereby pulmonary emboli (initial or recurrent) can be prevented in a patient who either cannot tolerate anticoagulant therapy (e.g., due to concomitant bleeding) or would be clinically compromised if a pulmonary embolism was sustained. It is important to recognize that these intravascular devices are not intended to treat a venous thromboembolic event but are designed to prevent thromboemboli from traveling to the pulmonary vasculature. Consequently, these devices have been shown to decrease the incidence of pulmonary emboli in the acute setting, but they are associated with an increased risk for IVC thrombosis in patients who are not on anticoagulant therapy with an IVC filter in place [16]. Retrievable filters are currently available and provide the option of subsequently removing the filter when it is no longer needed [17].
21.6.2 Duration of Therapy
Following completion of a standard course of anticoagulation, the next decision that must be made is whether anticoagulant therapy can be safely discontinued in the individual patient. Current evidence would suggest that the risk of recurrent VTE after stopping anticoagulant therapy is largely determined by two factors: (1) whether the acute episode of VTE has been effectively treated and (2) the patient’s intrinsic risk of sustaining a new VTE [15]. Several studies have demonstrated that durations of therapy less than 3 months are associated with an increased risk for recurrent VTE, supporting the current overall recommendation for a minimal duration of therapy for acute VTE of 3 months [15]. The decision to continue therapy beyond 3 months should be based on an assessment of the continued benefit of anticoagulant therapy compared to the risk for bleeding, in addition to patient preference for continued anticoagulation or stopping therapy.
21.7 Spontaneous vs. Provoked Event
For patients with a VTE occurring in the setting of orthopedic surgery or other high-risk procedures, the risk of recurrent thromboembolic events after cessation of anticoagulant therapy is extremely low [18]. Consequently, the American College of Chest Physicians (ACCP) recommends that these patients only need 3 months of anticoagulant therapy which can be stopped after completion [15]. Patients who sustain VTE in the setting of other transient risk factors, such as during pregnancy or after other surgical procedures, also have a relatively low risk for recurrent VTE [18]. Conversely, other acquired risk factors are associated with an ongoing increased risk for recurrent VTE, such as antiphospholipid syndrome or malignancy, and patients with VTE and these conditions generally require chronic anticoagulant therapy. Acquired risk factors associated with an increase in the risk for VTE are listed in Table 21.2.
Table 21.2
Recommendations for management of elevated INR results or bleeding in patients receiving vitamin K antagonist therapy
Condition | Intervention |
---|---|
INR above therapeutic range but <5.0; no significant bleeding | Decrease of hold dose; increase frequency of monitoring; if only minimally elevated, no dose reduction may be required |
INR ≥5.0 but <9.0; no significant bleeding | Hold 1–2 doses, increase frequency of monitoring, and resume at lower dose when INR is in the therapeutic range. In addition, can give vitamin K if the patient is at an increased risk of bleeding or if the patient requires urgent surgery (typically 1–5 mg orally, depending on the indication) |
INR ≥9.0; no significant bleeding | Hold warfarin therapy and give vitamin K; increase frequency of monitoring; give additional vitamin K if necessary. Resume therapy at a lower dose with INR therapeutic |
Serious bleeding at any elevation of INR | Hold warfarin therapy and give vitamin K (10 mg by slow IV infusion). Depending on the urgency of the situation, may supplement with prothrombin complex concentrates, fresh frozen plasma, or recombinant factor VIIa. Vitamin K can be repeated every 12 h |
Life-threatening bleeding | Hold warfarin therapy and give prothrombin complex concentrate or recombinant factor VIIa supplemented with vitamin K (10 mg by slow IV infusion); repeat if necessary depending on INR |
Patients who sustain a VTE in the absence of any acquired risk factors, on the other hand (referred to as “spontaneous” or “unprovoked” VTE), exhibit an increased risk for recurrent VTE after the cessation of anticoagulation [19]. The risk for recurrence has been reported to be as high as 22.6 % by 5 years after completion of therapy [20]. Consequently, the ACCP guidelines recommend that patients with a first unprovoked VTE should receive treatment with a vitamin K antagonist for at least 3 months [15]. After 3 months of therapy has been completed, it is recommended that all patients should be evaluated for the risk-benefit ratio of continued anticoagulant therapy, and for those patients with a first unprovoked VTE that was a proximal DVT or PE, in whom risk factors for hemorrhage are minimal and for whom good anticoagulant monitoring is available, “long-term” treatment is recommended [15]. The definition of long-term frequently represents an indefinite course of therapy (or until hemorrhagic risk exceeds antithrombotic benefit), since patients who are treated with anticoagulant therapy for longer than 3 months exhibit the same risk for recurrent VTE as individuals treated for 3 months only [21].
Given the cumulative hemorrhagic risk associated with long-term anticoagulant therapy, investigators have studied multiple strategies to try and identify which patients are at low risk for recurrent VTE and could therefore safely stop anticoagulant therapy. Various approaches that have been used in this regard are summarized herewith.
21.8 Inherited Thrombophilia
Individuals who sustain an unprovoked VTE will frequently have an underlying inherited thrombophilic risk factor (Table 21.3) [22]. Common thrombophilic states, such as factor V Leiden or prothrombin G20210A, which may occur in as many as 20 and 9 % of patients with a first-time VTE, confer a relatively mild risk for recurrent venous thrombosis [23]. Deficiency states of the natural anticoagulant proteins antithrombin, protein C, or protein S confer a higher risk for recurrent VTE [24], and certain investigators have recommended that patients with these disorders might merit a longer course of anticoagulant therapy after an initial thrombotic event [25]. Elevated levels of coagulation factors, particularly factor VIII, can also be associated with an increased risk for VTE. When considering whether or not an individual patient could discontinue anticoagulation after a course of therapy has been completed, however, it is important to recognize that the absence of one of these disorders has not been found to identify a subset of individuals who appear to have a lower risk for recurrent VTE [26]. Consequently, thrombophilia evaluations, while identifying a potential risk factor for the initial event, are generally not particularly helpful when determining an individual patient’s risk for recurrent VTE.
Table 21.3
Acquired prothrombotic disorders
Antiphospholipid antibodies |
Malignancy |
Myeloproliferative disorders |
Paroxysmal nocturnal hemoglobinuria |
Nephrotic syndrome |
Inflammatory bowel disease |
Pregnancy/postpartum |
Therapy-related (oral contraceptives, hormone replacement therapy) |
Trauma |
Surgery and the postoperative state |
Prolonged bed rest or immobilization |
Presence of a central venous catheter |
Elevated homocysteine levels |
21.9 Gender
Multiple studies have demonstrated that men have a higher risk for recurrent VTE after an initial event than women [27]. This increased risk remains after adjustment for previous hormonal therapy-related VTE in women [19]. This difference in risk is not observed in patients with a provoked VTE [19].
21.10 D-Dimer
The D-dimer reflects an ongoing natural hemostatic process, fibrinolysis, which is enhanced in individuals presenting with an acute VTE. Thrombin converts fibrinogen to soluble fibrin, which forms soluble dimers, trimers, and larger multimers that eventually form an insoluble plug at the site of vascular injury. The strength of the nascent clot is then enhanced by cross-linking of individual fibrin molecules to one another by the transglutaminase factor XIIIa. The clot is subsequently remodeled and broken down by plasmin, which cleaves the cross-linked mass of fibrin, releasing various fragments into the circulation. The D-dimer is one of these degradation products, consisting of two D-domains originating from two different fibrin molecules that were cross-linked to one another and then released from the fibrin clot by the process of fibrinolysis.
In patients with an acute VTE, the D-dimer is characteristically markedly elevated, reflecting ongoing thrombus formation and endogenous fibrinolysis. In fact, the presence of a normal D-dimer level in a patient with a low clinical suspicion for VTE can effectively rule out that diagnosis [28]. Several recent studies have shown that the presence of an elevated D-dimer level while on anticoagulant therapy, or the new development of an elevated D-dimer level after anticoagulation has been stopped, is associated with an increased risk for recurrent VTE [29]. Neither the timing of measurement of the D-dimer after stopping anticoagulation nor the age of the individual patient affected the ability of this test to detect patients at an increased risk for recurrent VTE [30]. Furthermore, patients with an elevated D-dimer level who continue (or resume) anticoagulant therapy exhibit a lower risk for recurrent thromboembolic events, in some cases even lower than the risk for recurrence observed in individuals with a normal D-dimer level after stopping anticoagulation [31]. Consequently, the D-dimer does appear to be a useful laboratory test in the assessment of risk for recurrent VTE after an initial event.
21.11 Residual Venous Thrombosis
Several investigators have studied the role of residual venous thrombosis as a predictor for patients at risk of recurrent VTE. Patients with no evidence for residual vein thrombosis identified at the completion of 3 months of anticoagulant therapy exhibited a very-low risk for recurrent VTE in the Duration of Anticoagulation based on Compression UltraSonography (DACUS) study [32]. In addition, those patients with residual vein obstruction detected exhibited a lower incidence of recurrent VTE if they continued anticoagulant therapy, supporting the concept of an extended course of therapy [32]. In contrast, the REVERSE investigators found that the presence of residual vein obstruction at the time of withdrawal of oral anticoagulant therapy was not associated with a statistically higher risk of recurrent VTE in a cohort study [33]. A systematic review and meta-analysis of 14 studies (five randomized controlled trials and nine cohort studies) found that residual vein obstruction was associated with a modestly increased risk for recurrent VTE in all patients (provoked and unprovoked), but not in unprovoked patients alone [34]. Limitations to this analysis included a lack of standardization of how residual vein obstruction should be assessed and prior reports of poor interobserver agreement [34]. Further prospective studies are necessary to assess the role of residual vein obstruction in patients with DVT.
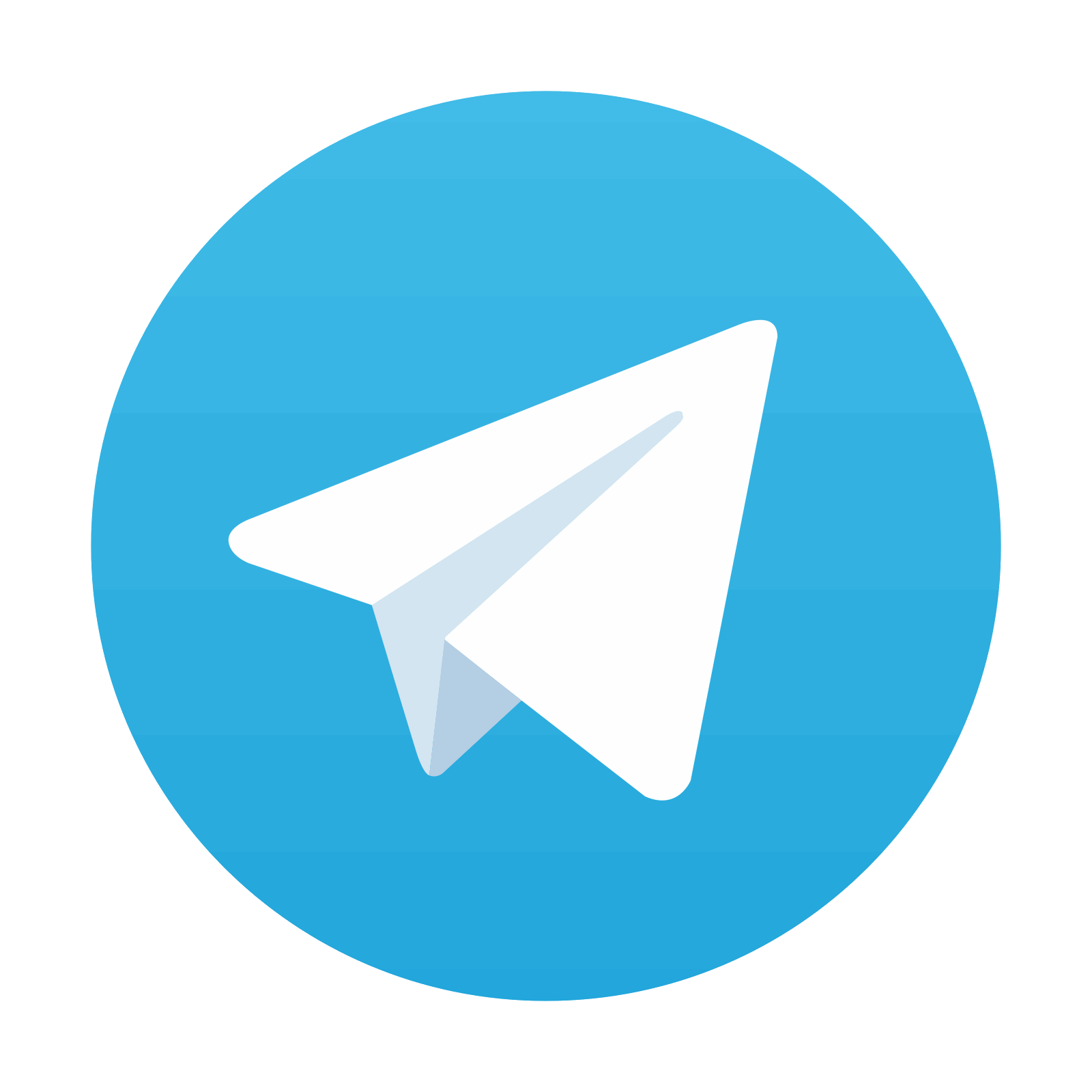
Stay updated, free articles. Join our Telegram channel

Full access? Get Clinical Tree
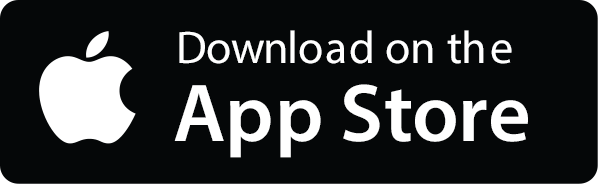
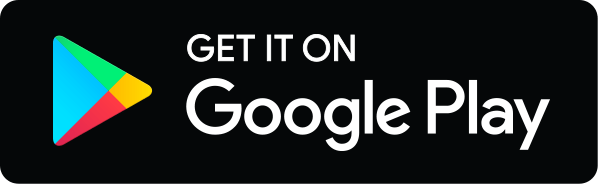