In the arterial system, hemoglobin saturation is generally above 93 %, whereas in the venous system, it may be 60–80 % in ordinary circulation (mixed venous blood) and lower in the setting of venous stasis with prolonged local recirculation times. Both oxyhemoglobin and deoxyhemoglobin molecules absorb laser energy over a broad range of wavelengths with differential peaks in the visible (blue/green/yellow) portion of the electromagnetic spectrum between 418 and 577 nm [3]. Examples of devices that produce wavelengths in this range include pulsed dye lasers (PDL), potassium titanyl phosphate lasers (KTP), and IPL sources used with the appropriate filters [19]. There is also a broad hemoglobin absorption peak from 800 to 1,000 nm, which is of special interest because longer wavelengths can penetrate more deeply into the dermis and thus offer the potential to reach deeper vessels.
13.7.4 Thermal Relaxation
Thermal relaxation time is defined as the time required for a given chromophore to lose 50 % of its heat through diffusion. If a laser pulse is longer than the thermal relaxation time, the chromophore will have absorbed all the energy it can for that pulse, and the remaining energy will be delivered to other tissues, reducing the tissue selectivity of the treatment. In contrast, if the desired energy can be delivered using a pulse of shorter duration than the thermal relaxation time of the target, the largest possible proportion of the light energy can be delivered directly to the desired target, with minimal heating of surrounding tissues.
13.7.5 Pulse Duration/Repetition
Pulse duration, often referred to as pulse width, is the time at which the laser power remains above half its maximum value. Pulse repetition is the number of pulses delivered per second, reported as Hz. In general, pulse duration should not exceed the practical thermal relaxation time. The size of the target is also a factor when considering thermal relaxation times: the larger the vessel the greater the amount of total thermal energy that must be delivered to raise its temperature, the greater the amount of energy available to injure adjacent tissues, and the longer it takes to cool down by thermal diffusion.
Delivering the same amount of energy over longer pulse durations (or delivering energy with repetition using multiple pulses) may exploit differential thermal relaxation times in different tissue types, potentially allowing delivery of greater amounts of energy to deeper tissues. Reducing the energy per unit time can also help to reduce epidermal heating. However, when vessels are small and superficial, the more selective thermal targeting of shorter pulse durations offers many advantages.
13.7.6 Fluence
Fluence, sometimes referred to as “radiant exposure,” is a measure of the number of photons delivered per unit area. For a fixed set of wavelengths, this is also a measure of the total energy delivered per unit area (usually measured in joules per square centimeter [J/cm2]). At a fixed power output and pulse width, the fluence will decrease if the spot size increases (the same energy is delivered over a larger area). Given the same laser power and the same spot size, a shorter pulse will deliver a lower fluence than a longer pulse.
13.7.7 Power Density (PD)
The power density or intensity of the laser beam is defined as the beam power per unit of cross-sectional area. If the same amount of energy is delivered over the same amount of time in a smaller spot size, the power density will be increased.
13.7.8 Epidermal Cooling
Absorption of laser light by epidermal melanin causes epidermal heating. In general, the shorter the wavelength, the greater the superficial absorption of energy and the greater the likelihood of epidermal injury. Surface cooling may help to protect the dermis, allowing the delivery of higher fluences to the targeted vessels. Epidermal cooling can also help with analgesia [12]. Epidermal cooling may be particularly useful when longer pulse durations are needed, because heat accumulates in the epidermis more quickly than it accumulates in the blood vessels being treated, increasing the risk of epidermal injury [13]. Commonly used methods for extrinsic cooling of the epidermis include cryogen spray, air cooling, and contact cooling with ice, cold gel, or sapphire or quartz crystals.
13.7.9 Summary
Selective photothermolysis leverages differences in laser power density, pulse width, and wavelength, along with differences in tissue chromophores, thermal relaxation time, and other ambient factors to produce targeted, selective damage to specific tissues while minimizing the effects on surrounding tissue. Selective treatment of a targeted chromophore occurs when a laser system is chosen with a wavelength matching the absorption spectrum of the target, using an appropriate energy level to sufficiently heat the tissue through energy absorption by the targeted chromophore, with a pulse duration shorter than the thermal relaxation time of the target.
13.8 Lasers Commonly Used in Treatment of Vascular Lesions
Lasers can produce light energy in several different modes, including continuous wave (CW), pulsed wave, and Q-switched. Continuous wave lasers deliver energy continuously, which makes them unable to exploit differential thermal relaxation times and reduces the selectivity of energy delivery, thus increasing the likelihood of thermal damage to surrounding tissue [2]. Pulsed lasers can deliver large amounts of energy over pulse durations measured in milliseconds. The term “Q-switching” refers to a process whereby the laser is first placed in a mode where it is unable to emit light yet is pre-saturated with energetic photons and then is suddenly allowed to emit light, resulting in a very fast emission of all the pre-stored energy in a very short pulse in the range of 10–250 ns. Lasers commonly used in the treatment of cutaneous vascular lesions are mostly pulsed or Q-switched lasers. A variety of suggested treatment parameters have been published for each class of laser. However, in practice, each patient is unique and each device performs differently, thus suggested parameters can be taken only as a rough guide to clinical therapy (Table 13.1).
Table 13.1
Summary of some laser applications
1,064 nm Nd:YAG | 125–150 J/cm2 | 6 mm spot | 25 ms pulse duration |
75–100 ms pulse duration (reticular veins) | |||
120–170 J/cm2 | 3 mm spot | 5–40 ms pulses | |
PDL | 6.5–7.5 J/cm2 | 3–5–7–10 mm spot | Pulse duration 20–40 ms |
5–8 J/cm2 | |||
532 nm KTP | 16–22.5 J/cm2 | 500–700 μm spot | Pulse duration 10–30 ms |
17 J/cm2 | 2 mm spot | ||
Long-pulsed 532 nm Nd:YAG | 12–14 J/cm2 | ||
IPL | 35–20 J/cm2 | 10 ms delay through a 550 nm cutoff filter |
13.8.1 Neodymium: Yttrium Aluminum Garnet (Nd:YAG)
The long-pulsed 1,064 nm Nd:YAG laser is most effective when treating facial telangiectasias and blue reticular vessels on the face. It has also been used to treat leg veins up to 4 mm in diameter, spider angioma, and cherry angiomas. The principal advantage of the 1,064 nm Nd:YAG is the fact that the longer wavelength allows for deeper penetration and weaker melanin absorption. The longer pulse duration at lower fluences translates into slower heating of the vessels. It has been claimed that this can cause photocoagulation without vessel rupture, minimizing the risk of purpura. This wavelength is well absorbed by both methemoglobin and deoxyhemoglobin and thus can deliver energy to darker blue veins [4].
Published recommendations include: for superficial vessels less than 1 mm in diameter, small spot size (2 mm), short pulse durations (15–30 ms), and high fluences (350–600 J/cm2) and for reticular veins of 1–4 mm in diameter, increased spot size (2–8 mm), longer pulse durations (25–60 ms), and fluences (90–370 J/cm2) [3].
In a study of 20 patients with size-matched superficial telangiectasias of the lower extremities, Lupton et al. compared sclerotherapy treatments to vein irradiation with the 1,064 nm Nd:YAG laser [5]. The telangiectasias responded best to the sclerotherapy, with fewer treatment sessions required, and similar adverse sequelae occurred in both groups. The conclusions were that lower extremity telangiectasias can be effectively treated with both modalities and that laser treatment may be more effective for patients with contraindications to sclerotherapy, including those with needle phobias, telangiectatic matting, or allergies to sclerosant solutions.
Sadick demonstrated longer term (12 months) successful photosclerosis of blue venulectasias and reticular feeder veins in 25 patients treated with the 1,064 nm Nd:YAG laser using a spot size of 6 mm [6]. Treatment parameters for vessels 0.2–2.0 mm: double pulse of 7 ms at 120 J/cm2; vessels 2.0–4.0 mm were treated with a single pulse of 14 ms and fluences of 130 J/cm2.
When using the 1,064 nm Nd:YAG laser with the proper settings, effective treatment of many cutaneous vascular lesions can be obtained, especially if epidermal cooling is available. However, complications are not uncommon and can include crusting, hyperpigmentation, hypopigmentation, scarring, transient erythema, bruising, edema, and telangiectatic matting.
13.8.2 Potassium Titanyl Phosphate (KTP) Laser
The potassium titanyl phosphate (KTP) laser, a quasi-CW system, uses an Nd:YAG source passed through a KTP crystal to double the frequency (halve the wavelength), producing a laser with 532 nm wavelength. The system has been further modified to produce millisecond (ms) pulse durations [7]. The resulting KTP laser system delivers high-energy pulses in spot sizes ranging from 0.25 to 4.0 mm and pulse durations of 1–50 ms.
The wavelength of 532 nm allows for some selective absorption by the hemoglobin chromophore, but epidermal melanin is also a target. Compared to the Nd:YAG laser, the shorter wavelength decreases the potential for deep tissue penetration, but this can be offset by extending the pulse duration up to 50 ms [4]. The KTP laser has been used in the treatment of telangiectasias on the face and legs, rosacea, spider angioma, and cherry angiomas. Weiss and Goldman [8] suggest the KTP laser system as one of the useful near-infrared pulsed lasers for the treatment of bright red vessels. Their most encouraging results were achieved with using a spot size of 3–5 mm, longer pulse durations of 10–50 ms, and fluences of 14–20 J/cm2, with a train of pulses delivered over the vessel until spasm occurs.
To evaluate the efficacy of the 532 nm KTP laser in the treatment of superficial leg telangiectasias, Fournier et al. [9] treated 14 patients with leg vessels, 0.5–1.0 mm in size. Using a nonuniform stacked pulse sequence, veins were treated with a total fluence of 60 J/cm2, 0.75 mm collimated spot size, with a pulse delay of 250 ms between pulses. The stacked pulses were 100, 30, and 30 ms delivering 38, 11, and 11 J/cm2, respectively. They demonstrated safe and effective treatment with minimum adverse effects. Side effects seen were transient and included erythema, edema, scabbing, hypopigmentation, and telangiectatic matting.
In addition, Woo et al. [13] compared treatment of telangiectatic leg veins in ten patients using a 532 nm Nd:YAG and a 595 nm PDL using ultra long pulse durations. Leg veins treated measured up to 1.0 mm in diameter. Both lasers showed improvement and some vessel clearance after one treatment with minimum side effects. Treatment parameters used with the Nd:YAG were a fluence of 20 J/cm2 and a pulse duration of 50 ms using a contact cooling device. The PDL laser used a fluence of 25 J/cm2, a pulse duration of 40 ms, and cryogen spray precooling.
13.8.3 Intense Pulsed Noncoherent Light (IPL)
The IPL is a noncoherent light source emitting light as a continuous spectrum within the 500–1,200 nm portion of the electromagnetic spectrum. It is used primarily in the treatment of facial telangiectasias and rosacea but is also indicated in the treatment of a variety of vascular lesions, including larger diameter vessels, spider angioma, and cherry angiomas. Light is delivered in a train of pulses, single, double, or triple, with varying time intervals between pulses. The IPL system uses a filtered flashlamp with filters used to remove lower wavelengths of visible light, while pulse durations can be adjusted to match desired thermal relaxation times [8, 19]. Using a light source longer than 600 nm potentiates deeper penetration of thermal energy, targeting the chromophore of deoxyhemoglobin.
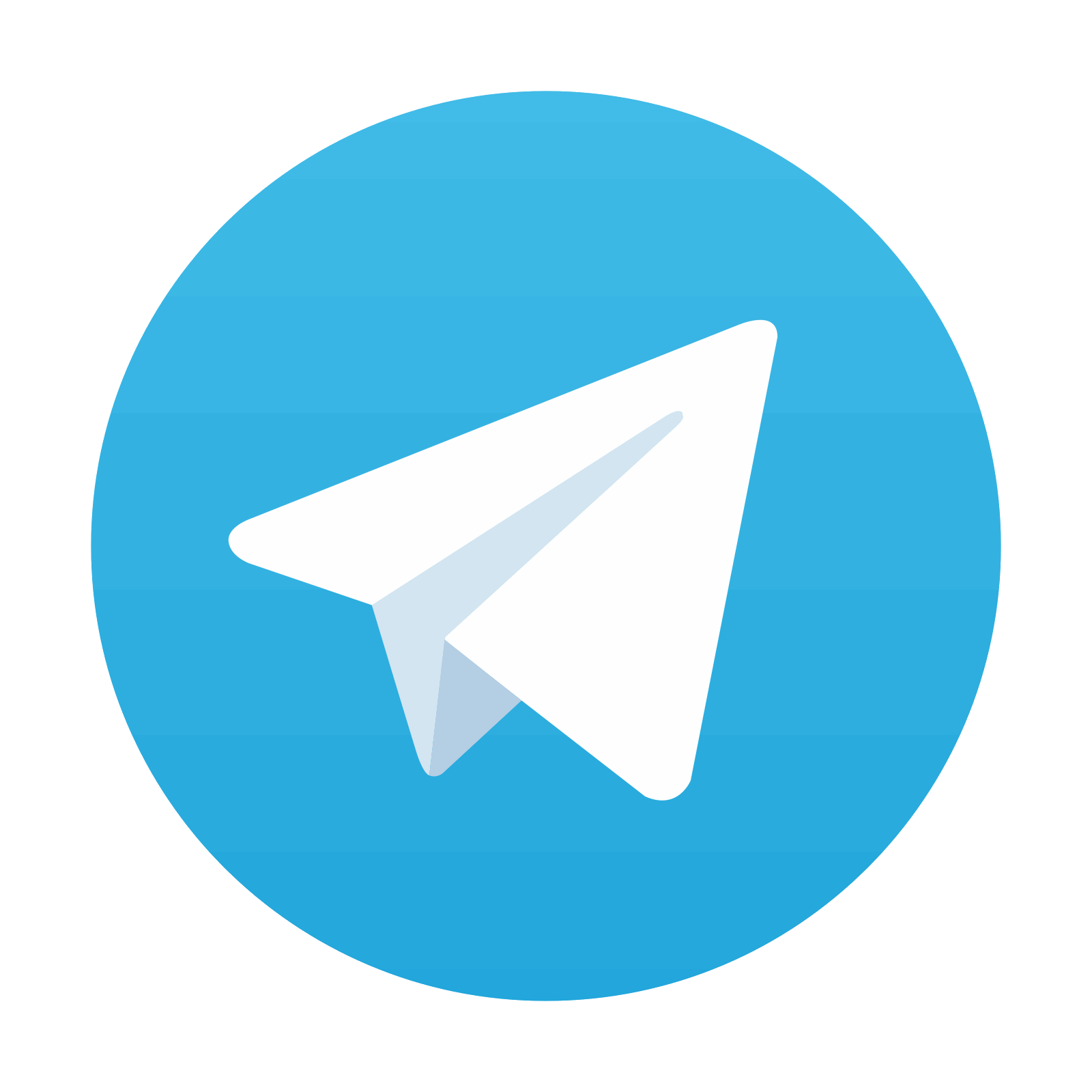
Stay updated, free articles. Join our Telegram channel

Full access? Get Clinical Tree
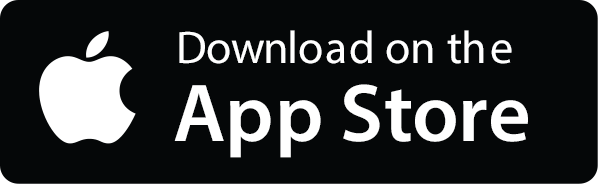
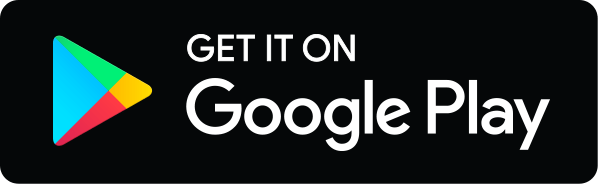