Antibody-Mediated Lung Defenses and Humoral Immunodeficiency
Antibody-mediated, or humoral, immunity is essential for host defense against respiratory pathogens. Defects in humoral immunity are common and frequently underappreciated. From a respiratory perspective, patients with impaired humoral immunity are susceptible to recurrent bacterial sinopulmonary infections and bronchiectasis. Antibody responses are also the principal mechanism behind the efficacy of vaccination against respiratory pathogens. This chapter focuses on our understanding of the normal B cell environment in the lung, generation of appropriate antibody responses after antigenic challenge, disease states associated with impaired lung humoral immunity, and the pulmonary response to vaccination.
OVERVIEW OF B CELL DEVELOPMENT (ONTOGENY)
The primary cell responsible for generating humoral immunity is the B lymphocyte. There are two phases in the development of antibody responses. The first phase is antigen independent and is called lymphopoiesis. As with the generation of other immune cells, the process begins with a multipotent stem cell in the bone marrow, which undergoes multiple maturation steps leading to mature but antigen naïve B lymphocytes (Fig. 24-1), which express IgM and IgD on the cell surface.1 This process occurs entirely within the bone marrow (or liver during fetal development). Important cytokines in this process include interleukin (IL)-7,2 ckit-ligand (stem cell factor),3 and IL-11.4 Mature B cells express the surface receptors CD19, CD20, CD21, and CD72.5,6 CD20 is especially relevant as it is the target of the monoclonal antibody rituximab, which is used to deplete B lymphocytes in B cell lymphoproliferative disorders7 and autoimmune disorders characterized by the production of pathogenic autoantibodies.8
Figure 24-1 Overview of B cell ontogeny. Lymphopoiesis, which occurs in the bone marrow or fetal liver, is an antigen independent process, which begins with a multipotent stem cell and ends with an IgM expressing immature B cell, which will migrate to lymphoid tissues. Immunopoiesis begins with antigen stimulation (hence antigen dependent) and results in antibody secreting plasma cells and antibody expressing memory B cells. Class switch recombination leads to the generation of different immunoglobulin subtypes (IgG, IgA, IgE) from activated IgM expressing B cells. BTK, Bruton’s tyrosine kinase, AID, activation-induced cytidine deaminase, UNG, uracil-N-glycosylase.
At the completion of lymphopoiesis IgM expressing B cells traffic to various lymphoid organs, including lymph nodes in the lung, to await antigenic challenge and enter immunopoiesis.9,10 Thus, unlike lymphopoiesis, immunopoiesis is antigen-dependent. The end result of immunopoiesis is the generation of antibody secreting plasma cells1 and long lived memory B cells, which secrete immunoglobulin only upon re-exposure to antigen.11 During immunopoiesis class switch recombination and somatic hypermutation occur resulting in the generation of IgA and IgG secreting cells out of IgM precursors and will be discussed in detail below. Immunopoiesis is most efficient in the presence of antigen activated T cells that provide “help” to B cells in the form of cytokines and cell surface activation signals. The most critical latter signal is the interaction between CD40 on B cells and CD40L on activated T cells. In the absence of such signaling (i.e., due to genetic mutations in CD40 or CD40L) class switching fails to occur, resulting in the accumulation of IgM secreting cells and the hyperimmunoglobulin M syndromes, discussed later in this chapter.
IMMUNOGLOBULIN DEVELOPMENT AND STRUCTURE
Below are discussed the basic structure of immunoglobulins and important characteristics of those immunoglobulins found in the lung.
BASIC STRUCTURE
Immunoglobulin (Ig) molecules are made up of two identical protein heavy chains and two identical kappa (κ) or lambda (λ) light chains.12 Immunoglobulin heavy-chain genes reside on chromosome 14 and immunoglobulin light chains are derived from either the Igκ locus on chromosome 2 or the Igλ locus on chromosome 22. Immunoglobulin heavy chains contain both a variable region that will ultimately contribute to antigen binding and a constant region that binds to cell Fc receptors and complement. Mature immunoglobulin light chains also contain variable and constant regions. Within each variable region, there are hypervariable regions that represent the actual antigen binding site and less variable regions which make up the framework region.
The variable domain of the heavy chain is encoded by three sets of genes, the variable (V), diversity (D), and junctional (J) genes, which must be physically rearranged to result in expression of immunoglobulin heavy-chain protein.13 Similarly, the variable region of the immunoglobulin light-chain protein is encoded by two sets of genes, the V and J genes, which are likewise rearranged to allow transcription of functional protein.13 The rearrangement of DNA to join V, D, and J heavy-chain genes and V and J light-chain genes also results in elimination of unused V, D, and J sequences and noncoding stretches of DNA or introns. The many possible variable regions resulting from these random rearrangements confer a broad range of potential antigen specificities to the mature immunoglobulin molecules.
CLASS SWITCHING
Once immunopoiesis is initiated B cells alter the isotype of antibody that is produced via isotype switching.13,14 This occurs in switch regions on immunoglobulin heavy chains through a process called class switch recombination. By translocation of the IgM variable region to switch regions adjacent to one of the constant regions coding for IgG, IgA, or IgE, the B cell is able to maintain antigen specificity and at the same time generate different immunoglobulin subtypes with different functional capabilities. Class switch recombination requires an enzyme called activation-induced cytidine deaminase (AID). AID also is critical for somatic hypermutation, a process where rapidly proliferating activated B cells have a high rate of point mutations in genes encoding the variable region leading to production of antibodies with different antigen binding affinities.15 This results in a greatly expanded repertoire of B cells expressing surface IgG of different affinities for the triggering antigen. B cells expressing high affinity antibody on their surface (and thus bind greater amount of antigen) have a survival benefit over B cells coated with weaker affinity to antigen, where less signaling through antigen recognition results in B cell apoptosis. In this way the antibody response moves toward production of higher affinity and more specific immunoglobulin. The importance of AID is reflected in the development of one of the hyperimmunoglobulin M syndromes in patients with defects in this enzyme.16 The structure of the various immunoglobulin classes is shown in Figure 24-2.
Figure 24-2 Basic immunoglobulin structure. Immunoglobulin molecules consist of two identical protein heavy chains and two identical light chains joined by disulfide bonds. Both heavy and light chains contain a constant and a variable region. Within each variable region there are hypervariable regions that represent actual antigen binding sites. IgM typically exists as a pentamer and IgA as a dimer.
LUNG IMMUNOGLOBULINS
In the lung immunoglobulins comprise the second largest class of proteins present in bronchoalveolar lavage (BAL) fluid after albumin.17 IgG is the major immunoglobulin in the lower respiratory tract18 In contrast, IgA is the most abundant immunoglobulin in secretions from the upper respiratory tract, exceeding the concentration of IgG by a ratio of 2.5:1. Smaller amounts of IgE are consistently found in the BAL fluid of normal subjects.17
IgG
IgG is the major circulating immunoglobulin found in the vascular compartment. In normal subjects, the amount of IgG measured in BAL (usually expressed as an immunoglobulin/albumin ratio) is in the same proportion as serum, suggesting that under resting conditions most IgG in the lung represents transudation from the vascular compartment.17,19 This is further supported by the relatively small size of IgG (150,000 D). There are four IgG subclasses found in BAL fluid in approximately the same proportions as found in serum. IgG1 represents approximately 60% to 70% of the IgG present in BAL fluid, IgG2 20% to 25%, and IgG3 and IgG4 are present only in small amounts (<5%).18 Responses to protein antigens predominantly reside in the IgG1 and IgG3 subclasses while polysaccharide antigens predominantly give rise to IgG2 antibodies.20,21
The major function of IgG is to opsonize pathogens and target them for clearance either through uptake by phagocytic cells or by fixing complement. In this regard IgG1 and IgG3 fix complement more avidly than IgG2.22 Antibody also binds to Fc receptors on phagocytic cells to facilitate uptake. The three primary Fc receptors are FcRI, FcRII, and FcRIII. IgG1 and IgG3 bind FcRIII receptors on phagocytic cells equally well and more potently than IgG2.23 IgG1 binds to the other Fc receptors more avidly than the other IgG subclasses. Binding of antigen–antibody complexes to Fc receptors is potentially a double-edged sword. On the one hand, not only is uptake of opsonized pathogens through Fc receptors more efficient, so is the intracellular digestion of organisms in phagolysosomes. For example, uptake of opsonized Mycobacterium tuberculosis through the Fc receptor results in intracellular killing of the organism, while uptake through other receptors (i.e., the mannose receptor) allows the organism to escape digestion.24 On the other hand, uptake of opsonized pathogens through Fc receptors represents an “inflammatory clearance” mechanism that is associated with release of potentially harmful inflammatory mediators into the lung environment.25
IgA
In contrast to IgG, IgA is likely locally produced based on an elevated IgA/albumin ratio in BAL compared to serum.26 IgA can exist as monomeric and polymeric proteins. The latter is usually found as dimeric IgA, characterized by two monomers connected by a J chain.27 Most IgA in the lung is in dimeric form, with features of secretory IgA (sIgA) characterized by the presence of a J chain and secretory component (SC).28 The large size of dimeric IgA (385,000 D) also argues against simple transudation of IgA from the vascular compartment into the lung. Finally, the presence of secretory component in BAL is another argument for the local production of IgA in the lung.29
IgA has two subclasses: IgA1 and IgA2.27 IgA1 comprises nearly 80% of serum IgA. In contrast, IgA2 appears to be important in mucosal immunity and nearly half of the IgA present in secretions is IgA2. IgA exerts its protective effect through three mechanisms.30 First, it serves as an immunologic barrier, inhibiting binding of organisms to mucosal surfaces. Second, the normal movement of IgA from the basilar to apical region of epithelial cells suggests that it may be effective in neutralizing intracellular pathogens. Finally, pathogens bound to IgA may be taken up by airway macrophages through the phagocytic process.
IgM
IgM is present only in very low amounts in respiratory secretions from normal subjects.17 It exists as a pentamer and the resulting very large size (900,000 D) limit transudation into the lung under normal conditions. Nevertheless, IgM in BAL is greater than would be expected for simple diffusion suggesting some local production.31 Furthermore, in some disorders characterized by lower respiratory tract inflammation IgM can be detected. However, in this setting it is almost certainly largely derived from serum transudation as part of the inflammatory process.
IgE
Under normal conditions the amount of IgE in BAL is lower than IgG, IgA, or IgM.31 When present the IgE/albumin ratio suggests local production.31 IgE–antigen complexes bind the high affinity receptor for IgE (FcRI) on mast cells, basophils, and eosinophils leading to immediate hypersensitivity responses.32,33 IgE is important for host defense against parasites mediated by eosinophils.34 However, in developed countries the main role of IgE in pulmonary immunity is in the pathogenesis of allergic and asthmatic disease.35,36 The severity of disease is correlated with serum IgE in asthma and allergic patients. The importance of this molecule in the pathogenesis of asthma has led to the development of anti-IgE antibodies for patients with resistant IgE-mediated disease.37 Interestingly, when IgE binds to the low affinity FcRII receptor it inhibits IgE synthesis and reduces inflammation.38 Thus IgE may also have a role in downregulating the immune response.
CELLULAR INTERACTIONS LEADING TO ANTIBODY SECRETING CELLS
A number of important cellular interactions take place that eventuate in antibody secretion by cells.
B1 AND B2 CELLS
B lymphocytes comprise 1% to 10% of the lung lymphocyte population and can be separated into two main classes. Plasma cells constitutively secrete IgG and other immunoglobulin subclasses.1,11 In contrast, memory B cells produce immunoglobulin only in response to re-exposure to particular antigens.11 B cells can be further classified into B1 and B2 cells. B1 cells were first described in the gastrointestinal tract. These cells are IgM+, CD5+ cells that do not require T cell help for development.39 In the lamina propria B1 cells undergo class switching to an IgA secretory cell. B1 cells home to peritoneal and pleural cavities, respond to common bacterial antigens, and are felt to be very important in the production of IgM and IgA against bacterial pathogens at mucosal sites.40 As such, they are thought to be important in innate immunity against conserved bacterial antigens. Because of their autonomous ability to secrete antibody, they are also felt to contribute to autoimmune diseases. These cells are difficult to demonstrate in normal lung. As stated earlier, T cells are not necessary for B1 cells to produce antibody, though the presence of T cells appears to augment the immune response.40–44
In contrast to B1 cells, B2 cells require T cell help, mainly through secretion of the cytokines interleukin IL-4, IL-5, IL-6, and IL-10, as well as ligation between CD40 on B cells and CD40L on T cells.45 T cell dependent antibody responses begin with uptake of antigen by accessory cells (AC) and presentation to T cells. AC function can be carried out by mononuclear phagocytes (monocytes, macrophages) or dendritic cells. Submucosal dendritic cells are likely the principal accessory cell in the lung.46 B cells themselves can also serve as the accessory cell leading to T cell activation.47 B cells in the lung express surface IgG and IgM. Crosslinking of surface IgG or IgM by antigen results in partial activation of B cells leading to expression of IL-2 receptors and MHC class II molecules,1 which allows them to serve as accessory cells in T cell activation. With appropriate T cell help activated B cells eventually differentiate into short lived antibody producing plasma cells or memory B cells, the latter characterized by the coexpression of CD20 and CD27.48 Other surface markers indicating B cell activation and differentiation include CD69, CD80, and CD86.1 The latter two interact with CD28 and CTLA4 on T cells, respectively, thereby enhancing proliferation of helper T cells. Activation results in production of CD38+ CD138+ plasma cells that secrete IgM (primary response) or other immunoglobulin isotypes (secondary immune responses).49
COGNATE VERSUS NONCOGNATE RESPONSES
Activated T cells can provide cognate and noncognate B cell help. Cognate B cell help is antigen and MHC restricted and occurs after helper T cells have been activated through T cell receptor (TCR)–MHC class II/antigen complex mechanisms. It was initially thought that B cells were the obligate accessory cells for cognate responses since B cell–T cell contact was occurring through MHC class II/antigen complexes on activated B cells and the TCR on T cells. In contrast, when T cells are stimulated by accessory cells other than B cells or activated in an accessory cell independent manner (i.e., with immobilized anti-CD3), T cells can induce immunoglobulin production in many B cells in a non-MHC or antigen (noncognate) restricted manner. This latter phenomenon results in stimulation of both antigen specific as well as “bystander” B cells leading to a polyclonal antibody response. Subsequent work has shown that this bystander response is only important when the T cells providing B cell help are highly activated and/or differentiated, such as after exposure to IL-4 and IL-6.49 This latter observation may explain the link between increased IL-6 secretion and nonspecific hypergammaglobulinemia in diseases such as HIV infection.50 Additional work has shown that this bystander effect is mediated through LFA-1 (CD11a/CD18) on B cells and ICAM-1 (CD54) on activated T cells.51,52
CYTOKINE SECRETION
Resting T cells provide poor B cell help. T cells that have been stimulated by antigen can be loosely divided into Th1 and Th2 cells based on the cytokine profile they secrete.53 Th1 cells secrete predominantly interferon-γ (IFN-γ) and interleukin (IL)-2 and are involved primarily in cellular immunity. Th2 cells secrete IL-4, IL-5, and IL-10 and drive humoral immunity. Th2 cells that provide B cell help are called “effector” cells. Signals from T cells to B cells are in two forms: direct cell to cell contact and cytokine secretion. While direct B cell–T cell contact is required for induction of antibody production, both Th1 and Th2 cell membranes can provide this signal.54 What differentiates these two T cell populations is the cytokine profile they secrete. In particular, IL-4 seems to be an early competence factor that increases the number of B cells responsive to T cell help.54,55 In contrast, IL-6 is a late factor55 that promotes continued B cell proliferation and immunoglobulin secretion. IFN-γ downregulates most immunoglobulin secretion.56 Thus Th2 cells secrete a more favorable cytokine pattern for immunoglobulin production. The one exception is IgG2, which is dependent on IFN-γ secretion.56
SPECIFIC B CELL AND T CELL RECEPTOR INTERACTIONS AND DOWNREGULATION OF B CELL RESPONSES
Tight regulation of antibody production and secretion is necessary to turn off the response when it is no longer needed and to prevent production of unwanted autoantibodies (Fig. 24-3). This control is mediated through a combination of signaling pathways between T cells and B cells. CD40L is an activation-induced molecule on CD4 T cells that delivers signals to B cells through its counterreceptor CD40, which is constitutively expressed on B cells. Highly activated T cells express increased CD40L. CD40 on B cells in turn downregulates CD40L on activated T cells.57 These cells are subsequently unable to stimulate resting B cells. Thus this may represent an attempt to downregulate noncognate immunoglobulin production. However, CD40 ligation is still critically important for cognate interactions. Crosslinking of surface immunoglobulin on B cells results in apoptosis of the B cell unless simultaneous binding of CD40 by CD40L occurs.58 Interestingly, CD40 binding alone induces Fas expression on B cells, an effect that is not inhibited even in the presence of B cell tropic factors such as IL-2, IL-4, and IL-10.59 Thus ligation of CD40 acts to costimulate B cells that have been activated by binding of specific antigen while simultaneously increasing sensitivity to apoptosis in B cells not crosslinked by antigen. This drives an antigen-specific antibody response.58
Figure 24-3 Interaction between T cells and B cells during the generation of a humoral response. Multiple signals occur between T cells and B cells. Depending on the presence or absence of crosslinking of surface immunoglobulin on B cells, T cells can either promote B cell growth or induce apoptosis.
The role of Fas/FasL interactions in modulating immune responses is well established. Activation of T cells upregulates expression of both Fas and FasL. This is seen as a mechanism to control chronic T cell activation.60 Fas/FasL interactions are also important in modulating B cell responses.61 As stated earlier, B cells stimulated through CD40 increase expression of Fas. Thus, these cells are susceptible to apoptosis mediated by FasL expressed by activated T cells. B cell apoptosis induced by FasL is blocked if there is simultaneous crosslinking of surface immunoglobulin by antigen, thereby preserving secretion of protective antibody in the presence of persistent pathogen exposure.
PULMONARY ANTIBODY PRODUCTION IN RESPONSE TO ANTIGEN EXPOSURE
Pulmonary immunity to pathogens can be divided into innate and acquired responses. Most pathogens gaining access to the alveolar space are handled by phagocytosis by alveolar macrophages, the principal form of innate immunity. If phagocytosis of pathogens occurs in the absence of opsonizing antibody this usually results in “noninflammatory clearance,” with minimal release of inflammatory mediators and preservation of lung structure and function.62 If phagocytosis is overwhelmed, a specific acquired immune response occurs. This involves the interaction of B and T lymphocytes to produce an antigen-specific cellular and humoral immune response. In the lung, the acquired response has three distinct phases (Fig. 24-4)—afferent antigen processing and transport to regional lymph nodes,63 presentation to naïve lymphocytes, and efferent migration of activated T lymphocytes and mature B cells back to the site of initial antigen challenge in the lung.64 This results in the presence of antigen-specific immunoglobulin in the lung lining fluid and consequent increased effectiveness of professional phagocytes such as alveolar macrophages and neutrophils.65
Figure 24-4 Pathway for generation of antigen-specific immune responses in the lung. Foreign antigen is taken up by antigen presenting cells and transported to regional lymph nodes where the primary cellular and humoral immune response is generated. Effector cells then traffic back to the lung to the site of initial antigen challenge. B cells will become antibody secreting plasma cells while others will become memory B cells. This circuit is significantly shorter in the presence of mucosal-associated lymphoid tissue (MALT).
This general pathway is similar for IgG and IgA production, though the length of the “circuit” is likely much less for IgA. Rather than having antigen travel to regional lymph nodes, in mucosal immunity IgA can be generated in specialized lymphoid tissue just beneath the mucosal surface, called mucosal-associated lymphoid tissue (MALT), suggesting that IgA secreting plasma cells are produced locally and IgA can readily diffuse back into the airspaces. Such submucosal lymphoid tissue in the upper airway, called nasal-associated lymphoid tissue (NALT), is seen in most animal species, including humans.66 In contrast, in the lower respiratory tract bronchus-associated lymphoid tissue, or BALT, is readily demonstrable in mice66 but has been more difficult to find in normal human airways.67 Prior studies claiming that humans have BALT have generally been in subjects with an inflammatory pulmonary process.68 Recent investigations may be shedding some light on this controversy, introducing the concept of inducible bronchus-associated lymphoid tissue, or iBALT. In mice lacking secondary lymphoid tissues (spleen, lymph nodes) a robust protective primary pulmonary B and T cell response was demonstrated after influenza infection.69 This response was associated with induction of B cell follicles centered around follicular dendritic cells in submucosal tissues. Thus collections of antigen presenting cells, B cells, and T cells in the submucosa, which are thought to collectively represent BALT, may only be readily detectable during times of antigenic challenge.
Once antibody producing B cells are formed in secondary lymphoid tissue or MALT, they must traffic back to the original point of entry of the pathogen. This trafficking of lymphocytes back to mucosal sites has been intensely studied, giving rise to a four-step model. The first step is tethering. L-selectin on lymphocytes interacts with addressins on endothelial cells to slow down the movement of lymphocytes through capillaries. In NALT, the responsible endothelial receptor is PNAd70 while in the lung adhesion is mediated by ICAM-1.71 Tethering leads to lymphocyte activation mediated by chemokines and their receptors and subsequent firm adhesion to the endothelium. The latter is mediated by LFA-1 binding to ICAM-1 and α4β7 integrin binding to MADCAM-1.72 Finally, diapedesis through the mucosa occurs, a process that probably involves all the above receptor–counterreceptor interactions.
The end result of this process is the accumulation of antibody secreting plasma cells at the site of initial challenge. Once the invading pathogen has been cleared, plasma cells disappear and are replaced by memory B cells. The great majority of B cells in the normal lung are mature memory B cells. This mirrors the predominance of memory T cells in the lung,73 suggesting that the lung is primed to respond quickly to antigenic challenges. IgG-, IgM-, and IgA-secreting B cells are present in normal subjects; however, poor correlations exist between the numbers of Ig producing BAL cells and the levels of Ig in BAL fluids.17,74 In BAL fluid from nonsmokers IgG is present in the same proportion as in serum, leading to speculation that most IgG enters via transudation from the plasma compartments.17,19 These two observations fit in well with the primary presence of memory B cells in the lung, suggesting that under resting conditions very little local antibody is made. Only after antigenic challenge is local production increased. In support of this is the finding of increased ratios of specific to total antibody in BAL fluid after pneumococcal infection suggesting that local antibody production can be increased after relevant exposures.75
DISORDERS OF HUMORAL IMMUNITY AND LUNG DISEASE
Either quantitative or qualitative defects may be associated with disorders of humoral immunity and resultant lung disease. In addition, lung disease has been noted in conjunction with development of autoantibodies. These topics are presented below.
INTRODUCTION
In general, defects in humoral immunity result in an increased susceptibility to bacterial infections, including recurrent sinusitis, bronchitis, pneumonia, otitis media, and even meningitis. In particular, the patients are susceptible to infection with encapsulated organisms such as Haemophilus influenzae, Streptococcus pneumoniae, Staphylococcus aureus, and Neisseria species. Chronic diseases such as bronchiectasis and obstructive lung disease can occur as a result of recurrent infection. Antibody defects can be either quantitative (an absolute decrease or loss of antibody) or qualitative (poor antigenic specificity and/or poor opsonic function). In addition, the presence of antibodies against host antigens can lead to autoimmune lung disease. Figure 24-5 shows some of the known molecular defects causing immunoglobulin deficiency. The cause of most of the selective immunoglobulin deficiencies described below is unknown.
Figure 24-5 Known sites of molecular defects leading to immunoglobulin deficiency. Note defects that occur past the mature B cell stage tend to lead to hyperimmunoglobulin-M syndromes while defects prior to this stage lead to loss of all immunoglobulin classes. BTK, Bruton’s tyrosine kinase, AID, activation-induced cytidine deaminase, UNG, uracil-N-glycosylase.
QUANTITATIVE DEFECTS
Isolated IgA deficiency is the most common primary immunodeficiency in humans, occurring in roughly 1 in 500 individuals.76 The presence of IgA deficiency is defined by a serum IgA level of less than 5 mg/dL and normal levels of other immunoglobulins. Asthma, IgE-mediated allergic disease, and autoimmune diseases are more common in patients with IgA deficiency.77 Only about a third of patients with IgA deficiency develop recurrent sinus, pulmonary, and gastrointestinal tract infections, usually with encapsulated bacteria. In the absence of an associated IgG subclass deficiency the development of structural lung disease is rare. These patients almost never require immunoglobulin replacement therapy. However, the coexistence of IgA and IgG subclass deficiency is extremely important to document since the latter may require immunoglobulin infusions. IgA deficient patients are at increased risk for anaphylactic reactions after blood product infusions, including immunoglobulin preparations, because of the presence of IgG anti-IgA antibodies in up to 60% of individuals.78,79 As such, when the decision is made to give immunoglobulin infusions to an IgG deficient patient with a coexisting low IgA level, immunoglobulin preparations low in IgA must be used.
In contrast to IgA deficiency, patients with IgG subclass deficiencies frequently exhibit recurrent sinus and respiratory tract infections with encapsulated organisms regardless of whether it is associated with IgA deficiency.80 IgG1 deficiency is the most common and it is frequently linked with deficiency of IgG2 and IgG3 as well. Unlike patients with IgA deficiency, the recurrent infections in IgG subclass deficiency can lead to chronic bronchiectasis, and assessment of immunoglobulin deficiency should be part of the standard evaluation of patients with unexplained recurrent infections with destructive lung disease. Patients with IgG2 subclass deficiency have an impaired ability to respond to polysaccharides.20 As such, their response to the standard polysaccharide pneumococcal vaccine is poor. When IgG subclass deficiency is combined with IgA deficiency the resultant lung disease is more severe than with either deficiency alone.
More severe disease occurs when there are multiple immunoglobulin defects. Perhaps the most common immunodeficiency in this group is common variable immunodeficiency (CVID). Present in 1 in 25,000 individuals, this disease is characterized by hypogammaglobulinemia, decreased antigen-specific antibody responses, and recurrent sinopulmonary infections, frequently leading to chronic lung disease.81 IgG levels are usually below 300 mg/dL and are accompanied by low IgA and IgM levels as well. Specific antibody responses to common antigens such as tetanus toxoid and pneumococcus are usually very low. Cellular immune defects, primarily lymphopenia with a normal or decreased CD4:CD8 ratio, may also be present. Thus, patients may present with infections typically associated with T cell defects (fungi, mycobacteria) in addition to infections caused by encapsulated bacteria. Proliferation of B cells and T cells in response to strong mitogens is impaired as well. Thus CVID is felt to result from a failure of B cells to terminally differentiate, either due to poor T cell help or an intrinsic B cell defect. The impairment in immunity and immunosurveillance probably contributes to the increased incidence of autoimmune disease, malignancies, and gastrointestinal malabsorption in patients with CVID.82–
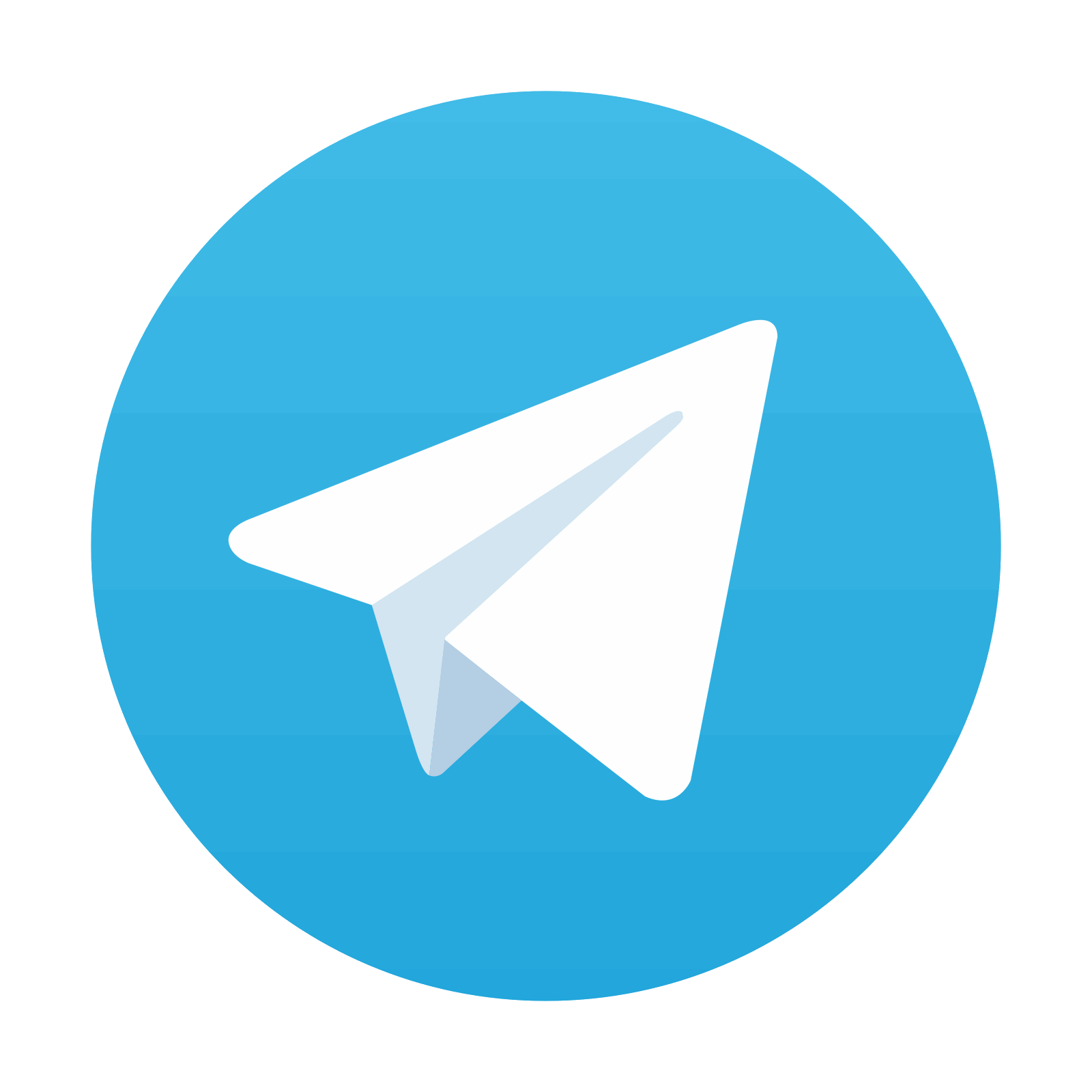
Stay updated, free articles. Join our Telegram channel

Full access? Get Clinical Tree
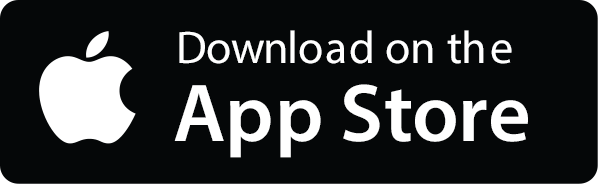
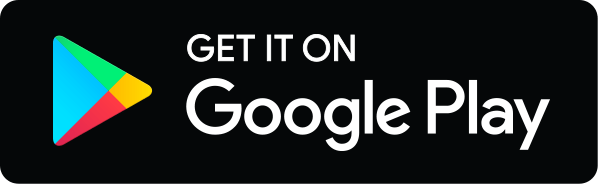