CHAPTER 88 Alternative Approaches to Coronary Artery Bypass Grafting
In 1967, Sabiston and colleagues1 reported the first clinically successful coronary artery bypass graft (CABG) procedure. Other early pioneers included Favaloro,2,3 who is credited with popularizing the use of autogenous saphenous vein, and Kolesov,4 who is generally recognized as having performed the first mammary artery to coronary artery anastomosis. During the several years that followed, centers around the world began performing the procedure in large numbers. Subsequent refinements in cardiopulmonary bypass (CPB), the introduction of cardioplegia, the growing acceptance of the left internal mammary graft, and the development of the intra-aortic balloon pump resulted in continued improvements in outcomes after CABG. By the early 1990s, more than 350,000 CABG procedures were being performed each year in the United States alone, with routine application in octogenarians, patients with severely impaired ventricular function, and patients with serious noncardiac morbidities.5,6
CARDIOPULMONARY BYPASS
The development of the heart-lung machine by Gibbon7 and its early application by Kirklin8–10 were among the most important advances in the ultimate growth of CABG surgery. CPB and, ultimately, the introduction of cardioplegia provided a motionless, blood-free surgical field that enabled the precision and reproducibility necessary for direct coronary anastomosis. Despite the obvious benefits of CPB, however, there has been an increasing awareness of its potential deleterious effects. As the formed elements of the blood come into contact with the surfaces of the various components of the heart-lung machine, they are activated, resulting in a number of hematologic and, ultimately, systemic changes. Typical sequelae include activation of complement, release of endotoxin, activation of leukocytes, increased expression of adhesion molecules, and release of inflammatory mediators (including cytokines, arachidonic acid metabolites, and free radicals).11 These sequelae occasionally are manifested as coagulopathy, third-space fluid retention, and subtle end-organ dysfunction, including neurocognitive changes. The magnitude of this systemic inflammatory response is generally modest, but it can be quite severe, especially when the time on CPB is prolonged. Furthermore, it is well recognized that a small percentage of patients with preoperative end-organ dysfunction suffer significant morbidity when they are subjected to even a moderate degree of systemic inflammatory response.
Ongoing efforts have been directed at reducing the magnitude of the systemic inflammatory response associated with CPB. These efforts include decrease in the surface area and modification of the surface composition of the CPB circuit to minimize surface activation, minimization of hemodilution by decrease of the volume of crystalloid prime needed to institute CPB, avoidance of the use of a cardiotomy sucker, and pretreatment of patients with various anti-inflammatory medications, such as aprotinin (Trasylol).12 Despite progress in these areas, performance of CABG without CPB remains attractive.
MULTIVESSEL OFF-PUMP CORONARY ARTERY BYPASS
Off-pump coronary artery bypass grafting (OP-CAB) is as old as coronary surgery itself. Large case series dating to the 1970s document its feasibility.13–15 Nevertheless, OP-CAB only recently gained widespread acceptance and entered the mainstream of clinical practice, propelled by a greater awareness of the potential morbidity of CPB and aortic manipulation and facilitated by improvements in surgical tools and techniques. OP-CAB is part of the procedural armamentarium of a growing proportion of surgeons worldwide and can be performed in virtually any patient requiring coronary revascularization.
Self-retaining Coronary Stabilizers
Compression-type stabilizers are generally two-pronged forks that are placed lightly on the epicardial surface of the heart, such that the prongs are parallel to and flanking the coronary artery at the intended anastomotic site. The undersurface of the prongs is designed to provide traction to avoid slippage. The fork is attached to the retractor by way of an articulating arm and can be locked tightly once the desired position has been achieved. In general, the best stability occurs when only slight downward force is used on the surface of the heart. Paradoxically, an increase in motion occurs when excessive downward force is applied. Therefore, the exposure techniques described later in this chapter should be used to present the target artery before the stabilizer is positioned, rather than relying on the stabilizer to retract the heart. Compression-type stabilizers are advantageous in that they set up quickly and simply and have an extremely low profile, allowing unrestricted access to the coronary artery. For some aspects of the heart, however, slightly more downward pressure may be required with compression stabilizers to avoid slippage (Fig. 88–1).
Suction-type stabilizers also are generally two-pronged forks attached to the retractor by means of an articulating arm. These stabilizers, however, are constructed with a series of ports on the undersurface of the prongs that are connected by sterile tubing to wall suction (−100 to −300 mm Hg). The epicardium and epicardial fat flanking the vessel are sucked into these ports, allowing the stabilizer to grip the surface of the heart. As such, less downward force is required at the anastomotic site. Suction stabilizers also set up quickly, but they require a regulated vacuum line. They have the added advantage of providing traction and countertraction of the fat surrounding the coronary artery, making them well suited for coronaries deep within the fat. Suction stabilizers tend to be slightly higher profile than compression-type stabilizers, but in general, access to the coronary artery is not a problem (Fig. 88–2).
Capture-type stabilizers are usually fenestrated platforms that frame the intended anastomotic site. Silicone elastic tapes are passed deep to the coronary artery and locked to the platform under tension, pulling the epicardium up against the platform’s undersurface (Fig. 88–3A). Like suction, this effect reduces the demand for downward force to prevent slippage. Capture stabilizers, by virtue of their circumferential stabilization, provide superior anastomotic stability.16 In addition, the silicone elastic tapes compress the coronary against the platform’s posterior aspect, providing integrated biplanar coronary occlusion (Fig. 88–3B). Capture stabilizers arguably are more complex to position, however, and require proper spacing and alignment of the silicone elastic tapes to ensure adequate hemostasis. Furthermore, because of their larger size, they may not be well suited if tightly spaced sequential anastomoses are being constructed.
Mechanisms of Adverse Hemodynamic Effects
Left Ventricle
When a coronary stabilizer is positioned with excessive downward force, the stabilized aspect of the heart is constrained, leading to diastolic dysfunction and decreased left ventricular end-diastolic volume, stroke volume, and cardiac output.17 Whereas volume loading and Trendelenburg positioning attenuate these effects by elevating left ventricular filling pressures, their recourse carries a risk of exacerbating intraoperative third-space fluid sequestration and associated morbidity. Similarly, the use of short-acting α-adrenergic agents can effectively maintain perfusion pressure in this setting, but these agents may be associated with an increased risk of perioperative mesenteric ischemia.18 β-Adrenergic agents should similarly be avoided in the setting of yet-to-be-grafted coronary disease. There are also anecdotal reports of severe mitral regurgitation during exposure of the lateral wall, possibly related to deformation of subvalvular structures in combination with coronary insufficiency. Exposure techniques that minimize or eliminate left ventricular deformation are therefore desirable.
Right Ventricle
Exposure of the lateral wall of the left ventricle displaces the heart toward the right, resulting in the right ventricle’s being compressed against the pericardium and the right side of the sternum. The relatively low pressure in the right ventricle makes it particularly vulnerable to deformation and reduction in right ventricular end-diastolic volume.19,20 The decreased right ventricular stroke volume leads to poor left ventricular filling and a drop in cardiac output. Volume loading and Trendelenburg positioning can compensate for this effect, as noted before, but not without potential risk. Right ventricular compromise is thought by many to be the dominant cause in many cases of hemodynamic instability that occur during exposure of the lateral wall. Various groups have proposed the use of right ventricular assist as palliation for right ventricular compromise during multivessel OP-CAB.21,22 Clearly, however, exposure techniques that minimize right ventricular deformation are desirable.
Myocardial Ischemia
Intraoperative myocardial ischemia, secondary to decompensated coronary disease, transient coronary snaring during grafting, or poor perfusion pressure during heart manipulation, constitutes another mechanism that leads to hemodynamic instability. Communicating closely with the anesthesiologist, planning a revascularization strategy that minimizes myocardial ischemia, and using exposure techniques that maintain hemodynamic stability best prevent it. Although each case should be managed on an individual basis, useful axioms exist. Patients with significant left main stenosis and marginally compensated ischemia may benefit from completion of the left internal mammary artery (LIMA) to left anterior descending (LAD) graft before the heart is manipulated. Similarly, initial revascularization of occluded, collateralized coronary arteries should be carried out before transiently occluding those vessels that supply collaterals to them. The selective use of intracoronary shunts, aorta-coronary shunts, or assisted coronary perfusion devices23 can be essential in grafting a moderately stenosed right coronary artery proximal to the origin of the posterior descending branch or in grafting the proximal portion of a moderately stenosed LAD (Fig. 88–4). The judicious use of an intra-aortic balloon pump can occasionally prove invaluable.24
Exposure Techniques
Depending on the size and shape of the patient’s heart and the dimensions of the chest cavity, visualization of the proximal obtuse marginal and posterolateral branches of the circumflex system may be obstructed by the left hemisternum. Although intuitive attempts at improving exposure may involve the application of additional rightward force with the stabilizer to that aspect of the heart, this maneuver frequently leads to hemodynamic compromise as well as paradoxically poor stabilization. Maximized access and stability at the anastomotic site with minimal compromise of cardiac performance is most readily achieved when the stabilizer is applied with little or no pressure; thus, coronary stabilizers should be used only to stabilize the anastomotic field rather than to assist in retracting the heart. In many patients with relatively normal hearts and readily accessible coronaries, little attention to exposure techniques is required. In contrast, in performing multivessel OP-CAB on patients with large, poorly contracting hearts, attention to these techniques is essential. In these patients, exposure of the lateral wall of the heart is best obtained with maneuvers such as the use of deep pericardial sutures, right pleurotomy and pericardiotomy, right hemisternal elevation, and apical suction devices, each of which is described.
Deep Pericardial Sutures
One of the most important advances in exposure techniques for OP-CAB has been the use of deep pericardial sutures. First described by Lima,25 these sutures, when placed under tension, create a ridge of pericardium that supports the base of the lateral left ventricle adjacent to the atrioventricular groove and allows the heart to be rotated rightward to assume an “apex up” position (Fig. 88–5). In this subluxed position, the apex of the heart points toward the ceiling and protrudes through the sternotomy incision, often above the plane of the sternal retractor. This generally allows adequate exposure of the lateral and inferior aspects of the left ventricle before the coronary stabilizer is applied.
How deep pericardial sutures are placed varies among surgeons and according to the patient’s anatomy. In general, one or two 2-0 silk sutures are placed in the pericardium posterior to the left phrenic nerve, immediately anterior to one or both left pulmonary veins: one deep in the oblique sinus behind the left atrium and one to the left and posterior to the inferior vena cava (Fig. 88–6). Care must be taken in placing these sutures to avoid injury to underlying structures, such as the esophagus and lung.26 The suture in the oblique sinus is particularly important for obtaining good exposure of the lateral wall near the base of the heart. One commonly used technique consists of placing a single, deep pericardial suture in this location, which is used to secure the midpoint of a 50-cm gauze strip deep in the oblique sinus. Subsequent traction on the two ends of the strip can then be adjusted to optimize exposure of different surfaces of the heart.
Right Pleurotomy and Right Vertical Pericardiotomy
Right lateral pericardiotomy can be performed with a right vertical incision extending from the cut edge of the initial anterior pericardiotomy, 2 cm cephalad and parallel to the diaphragm, down to the level of the inferior vena cava. Care must be taken to avoid injury to the right phrenic nerve (Fig. 88–7). The 2-cm rim of pericardium on the diaphragm facilitates closure of the pericardiotomy once grafting is complete. Caution should be exercised in measuring right-sided grafts as closure of the lateral pericardial incision may affect their lie. Many surgeons advise closure of the lateral pericardiotomy before construction of the proximal anastomosis of a right graft to ensure a tension-free, non-kinking graft lie. In some circumstances, the right pericardiophrenic fat pad, which can be quite large, can be removed and tidal volume decreased to provide additional room for the easily deformed right ventricle. These techniques are extremely valuable in obtaining lateral wall access in patients with enlarged hearts and marginal hemodynamic stability; however, they are of some benefit in nearly all patients.
Right Hemisternal Elevation
Asymmetrical right hemisternal elevation can further improve lateral wall exposure when it is used in conjunction with the preceding techniques. Once the right pericardium and pleura are incised, the right half of the sternum and right blade of the retractor limit rightward displacement of the subluxed apex of the heart. By elevation of these structures, the apex can clear the posterior aspect of the chest wall. This allows the entire heart to rotate into the right pleural space with little change in left and right ventricular geometry and end-diastolic volume (Fig. 88–8). As a result of these maneuvers, the proximal obtuse marginal and posterolateral branches are brought toward the center of the operative field and into the surgeon’s view.
Right hemisternal elevation is best accomplished if it is carried out as the sternotomy incision is being performed. By application of anterior traction on the right chest wall when the retractor is first spread, costal microfractures that inevitably form during sternal opening will predominantly occur in the right-sided ribs. The resulting increase in flexibility of the right chest wall will subsequently prevent the need for exaggerated tilting of the sternal retractor, which is often required to overcome the tendency of the left chest wall to rise after internal mammary artery harvest. The diaphragmatic insertion on the inferior aspect of the right hemisternum can also be released to facilitate right hemisternal elevation and create additional room for displacement of the apex of the heart into the right pleural space.
Apical Suction Devices
Self-retaining apical suction retractors have recently been added to the operative armamentarium of OP-CAB surgeons. These tools are used to position the heart for exposure of its lateral and posterior surfaces and have little impact on hemodynamics. These retractors generally consist of a suction cup mounted at the end of an articulating arm that can be locked in position relative to the retractor (Fig. 88–9). The suction cup is placed at or near the apex of the heart and is held in position by vacuum (300 mm Hg). Pulling the apex away from the base and pivoting the cardiac axis can achieve excellent exposure with little impact on left ventricular geometry and left ventricular end-diastolic volume. Although the use of these devices involves additional expense, the easy affixation and their rapid setup make them an attractive adjunct for challenging cases (Fig. 88–10). In many OP-CAB centers, apical suction retractors, in conjunction with right pleurotomy, vertical pericardiotomy, and sternal tilt, are used in the majority of cases.
< div class='tao-gold-member'>
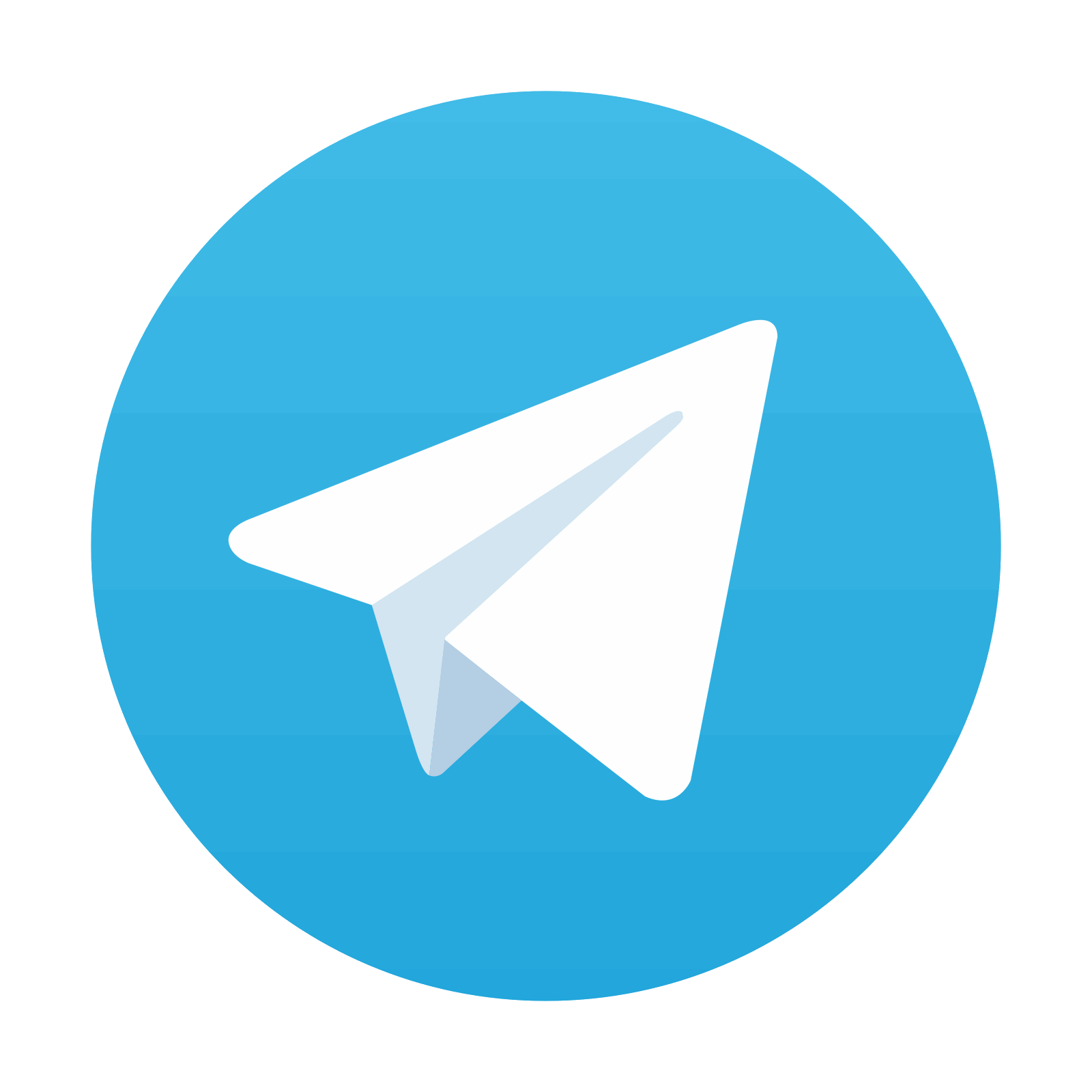
Stay updated, free articles. Join our Telegram channel

Full access? Get Clinical Tree
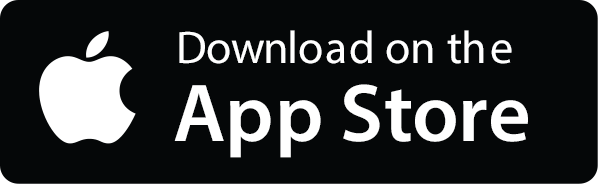
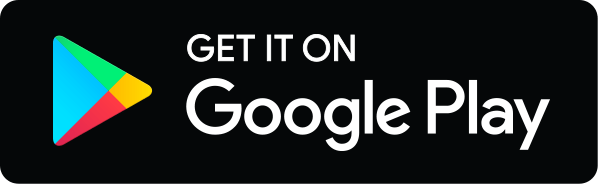