Pulmonary hypertension includes heterogeneous diagnoses with distinct hemodynamic pathophysiologic features. Identifying elevated pulmonary vascular resistance (PVR) is critical for appropriate treatment. We reviewed data from patients seen at referral pulmonary hypertension clinics who had undergone echocardiography and right-side cardiac catheterization within 1 year. We derived equations to estimate PVR using the ratio of estimated pulmonary artery (PA) systolic pressure (PASP Doppler ) to right ventricular outflow tract velocity time integral (VTI). We validated these equations in a separate sample and compared them with a published model based on the ratio of the transtricuspid flow velocity to right ventricular outflow tract VTI (model 1, Abbas et al 2003). The derived models were as follows: PVR = 1.2 × (PASP/right ventricular outflow tract VTI) (model 2) and PVR = (PASP/right ventricular outflow tract VTI) + 3 if notch present (model 3). The cohort included 217 patients with mean PA pressure of 45.3 ± 11.9 mm Hg, PVR of 7.3 ± 5.0 WU, and PA wedge pressure of 14.8 ± 8.1 mm Hg. Just >1/3 had a PA wedge pressure >15 mm Hg (35.5%) and 82.0% had PVR >3 WU. Model 1 systematically underestimated catheterization estimated PVR, especially for those with high PVR. The derived models demonstrated no systematic bias. Model 3 correlated best with PVR (r = 0.80 vs r = 0.73 and r = 0.77 for models 1 and 2, respectively). Model 3 had superior discriminatory power for PVR >3 WU (area under the curve 0.946) and PVR >5 WU (area under the curve 0.924), although all models discriminated well. Model 3-estimated PVR >3 was 98.3% sensitive and 61.1% specific for PVR >3 WU (positive predictive value 93%; negative predictive value 88%). In conclusion, we present an equation to estimate the PVR, using the ratio of PASP Doppler to right ventricular outflow tract VTI and a constant designating presence of right ventricular outflow tract VTI midsystolic notching, which provides superior agreement with catheterization estimates of PVR across a wide range of values.
Right-sided cardiac catheterization is the reference standard for hemodynamic evaluation of patients with pulmonary hypertension, including pulmonary vascular resistance (PVR) estimation. Right-sided cardiac catheterization is invasive and cannot be applied repeatedly to all patients with suspected pulmonary hypertension. The estimated pulmonary artery (PA) systolic pressure (PASP Doppler ) from transtricuspid flow velocity (TTFV) is at the core of the echocardiographic evaluation of suspected pulmonary hypertension. The PASP does not define PVR, however; patients with vastly different PVR values can have identical PASP. Noninvasive PVR estimation would be useful for diagnosis and monitoring response to therapy. Several investigators have proposed echocardiographic PVR prediction models. Some have used the timing of the right ventricular outflow tract flow to estimate PVR. Others have used a ratio of a correlate of PA pressure to PA flow in the denominator. An early model based on the ratio of TTFV to right ventricular outflow tract velocity time integral (VTI) has been validated to predict PVR in patients with cirrhosis and adverse outcomes among coronary disease patients. This model, however, was derived in patients with normal or mildly elevated PVR and has poor agreement at higher levels of PVR. Equations using transtricuspid pressure gradient (4 × TTFV 2 ) in place of TTFV have been derived for patients with markedly elevated PVR. Others have evaluated a multiparameter approach involving the estimation of all variables involved in calculating the PVR (mean PA pressure, left atrial pressure, stroke volume, and heart rate). This approach is time intensive and therefore less clinically useful. Our objectives were to derive and validate a simple equation to accurately estimate PVR for widespread application in a diverse referral population of patients with pulmonary hypertension or suspected pulmonary hypertension.
Methods
The cohort included patients seen by the pulmonary hypertension services at the Hospital of the University of Pennsylvania, Brigham and Women’s Hospital, Boston Children’s Hospital, or Massachusetts General Hospital who had undergone right-sided cardiac catheterization and transthoracic echocardiography from March 2002 to January 2012. The exclusion criteria were >12 months between right-sided cardiac catheterization and transthoracic echocardiography, interval initiation of pulmonary vasodilator or loop diuretic or cardiovascular or abdominal surgery or a change in clinical status, unrepaired congenital heart disease, or intravenous inotropes or vasopressors or positive pressure ventilation at the time of either study. We excluded 44 of 261 (17.1%) otherwise eligible patients because of poor-quality tricuspid regurgitation signals. The median interval between right-sided cardiac catheterization and transthoracic echocardiography was 21.5 days (interquartile range 5 to 55.5). The patients were classified according to the World Health Organization pulmonary hypertension diagnostic groups.
The patients had undergone clinically indicated transthoracic echocardiography with the Philips Sonos 7500 or IE33 (Philips Medical Systems, Andover, Massachusetts) or GE Vivid 7 Ultrasound (GE, Milwaukee, Wisconsin). The measurements were made by an echocardiographer (A.R.O.), who was unaware of the invasive hemodynamics data, in accordance with the American Society of Echocardiography guidelines using AcusonKinetDx (WS3000 Diagnostic Workstation, Siemens Medical Solutions USA, Mountain View, California) or Showcase Premier 5.3 (Trillium Technology, Ann Arbor, Michigan). At least 3 cardiac cycles were measured (5 to 10 with irregular rhythms), and average values were used.
The left atrial anteroposterior end-systolic dimension was measured from the parasternal long-axis view. Right ventricular outflow tract pulse wave Doppler interrogation was performed from the basal short-axis views just proximal to the pulmonic valve. By inspecting the pulse wave Doppler signal from the right ventricular outflow tract, the presence of a midsystolic notch was characterized as a distinct notch or nadir within the initial 2/3 of the systolic ejection period. TTFV was obtained from continuous wave Doppler interrogation in the view that provided the best envelope with the highest estimates. Left ventricular inflow pulse wave Doppler was used to measure the peak E/A velocities. Lateral mitral annular tissue Doppler was used to measure e′ velocity.
Right-sided cardiac catheterization was performed using a balloon-tipped, fluid-filled catheter, as previously described. No sedation was administered for most catheterizations, and minimal to moderate conscious sedation was used for a subset with spontaneous ventilation. Supplemental oxygen was not administered unless the patient used chronic supplemental oxygen at rest, in which case the same dose was used during right-sided cardiac catheterization. The cardiac output was estimated either by triplicate thermodilution (n = 83) or assumed Fick (n = 134). Oxygen consumption was assumed to be 125 ml/m 2 body surface area. No difference (p = 0.74) was seen in the mean cardiac output between the 2 groups, and the method used did not significantly modify the relationship between the right ventricular outflow tract VTI and cardiac output (i.e., no 2-way interaction).
Categorical data are expressed as proportions, and continuous data are presented as the mean ± SD or median and interquartile range, as appropriate. Unpaired t tests and Wilcoxon rank sum test were used to compare the mean values for normally and non-normally distributed continuous variables, respectively. The chi-square test or Fisher’s exact test was performed to compare the proportions for categorical variables.
We compared the derived models to a published model from Abbas et al based on the ratio of TTFV to VTI:
PVR = 10 × TTFV RVOT VTI + 0.16 (model 1)
The overall sample was divided using random sampling into derivation and validation samples. To derive the model, we used linear regression analysis and forward selection, with p <0.10 for entry. The variables were retained if they increased r 2 by ≥0.02. Potential variables included the left atrial anteroposterior dimension, lateral mitral E/e′, TTFV, 4 × TTFV 2 , estimated PASP using a fixed right atrial pressure of 8 mm Hg (PASP Doppler ) or variable right atrial pressure of 5, 10, or 15 mm Hg or 3, 8, or 15 mm Hg according to the inferior vena caval diameter and collapse, PASP Doppler -E/e′, acceleration time, right ventricular outflow tract midsystolic notching, right ventricular outflow tract VTI, and ratios of the following variables to VTI: TTFV, 4 × TTFV 2 , and PASP Doppler (= 4 × TTFV 2 + 8). Given the potential for model instability in the setting of collinear parameters, we also assessed the predictive value of alternative models to confirm the derived model was associated with the highest r 2 .
The derived model was PVR = −0.05 + 1.0 × (PASP Doppler /VTI) + 2.8 × (midsystolic notching), where midsystolic notching was 1 if present and 0 if absent (partial r 2 = 0.596 for PASP Doppler /VTI and partial r 2 = 0.068 for the midsystolic notching term). To simplify, we rounded the coefficients to the nearest integer, given the clinical insignificance of PVR ± 0.25 WU. We further evaluated a model that included only PASP Doppler /VTI, given the potential for increased simplicity and to test the value of midsystolic notching in the validation cohort. Sensitivity analyses were performed to assess model performance in specific subsets of patients (e.g., high PA wedge pressure) and whether alternative parameters might improve the model. Bland-Altman plots were used to assess the agreement between the derived models and PVR. Model discrimination for clinically relevant levels of PVR (>3 or >5 WU) was assessed using receiver operating characteristic curves, area under the curve, and sensitivity, specificity, and negative and positive predictive values.
Thus, the simple PASP Doppler /VTI model was as follows:
where RVOT is the right ventricular outflow tract.
The comprehensive model was as follows:
where RVOT is the right ventricular outflow tract.
We also assessed the agreement between PVR and a model derived by Kouzu et al in a population enriched for elevated PVR (μPVR = 16.2 WU):
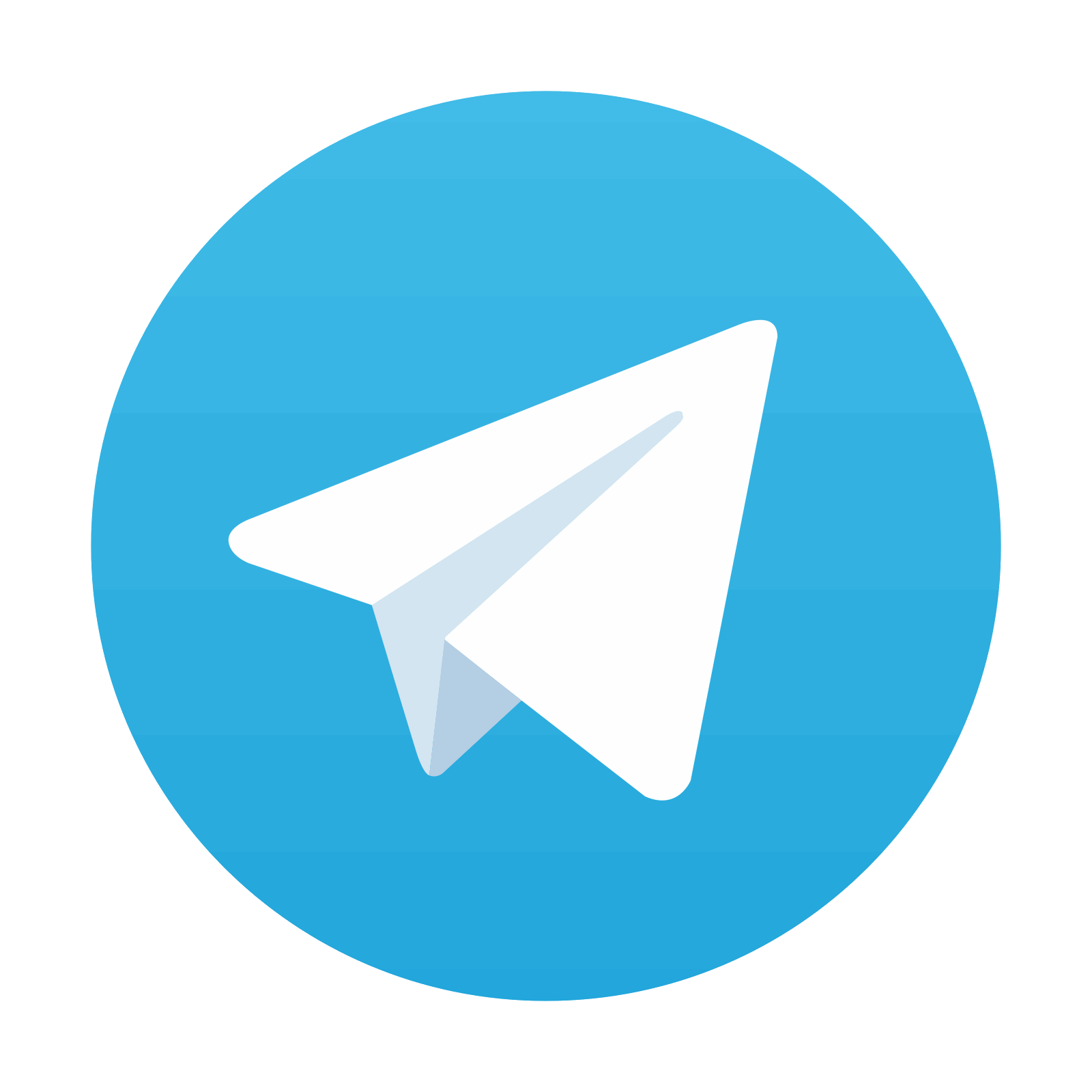
Stay updated, free articles. Join our Telegram channel

Full access? Get Clinical Tree
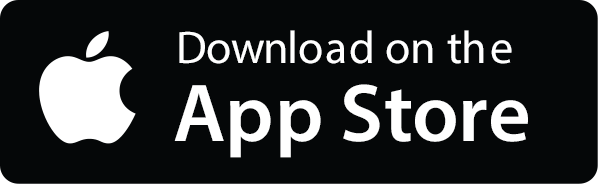
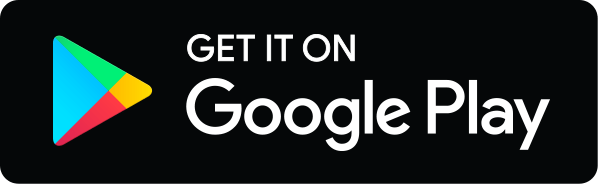