CHAPTER 21 Richard A. Santa‐Cruz and George A. Stouffer The idea of using diastolic augmentation to treat left ventricular failure was first proposed in the 1950s. The earliest method involved removing blood from the femoral artery during systole and replacing this volume rapidly during diastole. In the early 1960s an experimental prototype of the intra‐aortic balloon pump (IABP) was developed, in which inflation and deflation were triggered by the electrocardiogram (ECG). The initial use of IABP in clinical practice was in 1968. The early devices were 15 French in size and required surgical insertion and removal. Subsequent improvements included a dramatic reduction in size, enabling percutaneous insertion. Current indications for IABP include cardiogenic shock or left ventricular failure, unstable ischemic syndromes, mechanical complications of acute myocardial infarction, ischemic ventricular arrhythmias, severe mitral regurgitation, stabilization of patients undergoing coronary artery bypass grafting with high‐risk anatomy (e.g., severe left main disease), failure to separate a patient from cardiopulmonary bypass, high‐risk coronary intervention, or as a bridge to a left ventricular assist device (LVAD) or heart transplantation (Table 21.1). Relative contraindications to IABP usage are listed in Table 21.2. Table 21.1 Indications for IABP. Table 21.2 Relative contraindications for IABP. The IABP consists of a polyurethane balloon placed in the patient’s descending aorta and a console that rapidly shuttles a gas, either helium or carbon dioxide, into and out of the balloon. Helium is most commonly used and has the advantage of a lower density and therefore a better rapid diffusion coefficient. Carbon dioxide, on the other hand, has an increased solubility in blood and thereby reduces the potential consequences of gas embolization following a balloon rupture. The IABP is placed with one tip below the origin of the left subclavian artery (a useful angiographic marker is the left main stem bronchus). The other tip should be positioned above the renal arteries. It is important to match the appropriate balloon size to patient size, since mismatches can cause either ineffective counterpulsation or possible mechanical trauma to the aorta. In general, a 40 cc balloon size is used, although sizes from 25–50 cc are available. An IABP produces hemodynamic effects through the cardiac cycle. During diastole, the IABP inflates, thereby displacing blood from the descending aorta. The balloon deflates immediately before systole, resulting in decreased aortic impedance (Figure 21.1). The primary hemodynamic benefits of IABP treatment are diastolic pressure augmentation and afterload reduction (Table 21.3). Diastolic augmentation can increase coronary perfusion pressure, mean arterial blood pressure, and systemic perfusion, although these effects have not been consistently found in all studies. As shown in Figure 21.2, diastolic pressure augmentation by aortic counterpulsation can be a major support of mean arterial blood pressure in patients with cardiogenic shock. Afterload reduction can decrease left ventricular filling pressures and decrease myocardial oxygen demand. The decrease in afterload generally results in a decrease in left ventricular end‐diastolic pressure (LVEDP) during counterpulsation, with preservation or increase of the left ventricular stroke volume and ejection fraction. Left ventricular work is decreased by a reduction in afterload, which results in lower myocardial oxygen demand. The decrease in systolic pressure is generally less than the increase in diastolic pressure, resulting in an increase in mean arterial blood pressure. Figure 21.1 IABP inflation and deflation. During diastole, the IABP is inflated, increasing diastolic pressure, thus augmenting flow not only into the coronary arteries but also the great vessels and the renal arteries (a). Just prior to systole, the IABP is deflated, creating a void where the inflated balloon was, thus increasing forward flow into the aorta and to the periphery (b). Table 21.3 Hemodynamic effects of IABP therapy. Figure 21.2 Aortic counterpulsation in a patient with cardiogenic shock, left ventricle to aortic pullback. The patient was a 48‐year‐old male who presented with an extensive anterior–lateral myocardial infarction. An IABP was placed via the left femoral artery. An end‐hole catheter was inserted into the left ventricle and pressures were recorded as the catheter was withdrawn into the aorta. Note the decreased left ventricular systolic pressure and increased left ventricular end‐diastolic pressure. IABP diastolic augmentation is a major contributor to aortic pressure. Because an IABP works via diastolic pressure augmentation and afterload reduction, the beneficial effects are dependent on heart rate, cardiac output, and blood pressure. Diastolic augmentation is limited in patients with poor cardiac output independent of how well the IABP is functioning. This is in contradistinction from extracorporeal membrane oxygenation (ECMO) or an LVAD, in which hemodynamic support is generally independent of intrinsic cardiac activity. Counterpulsation has many metabolic effects on both ischemic and nonischemic myocardium. In general, aortic counterpulsation results in decreased myocardial metabolic demands. Increased end‐organ perfusion is probably an important contributor to the overall improvement in patient status associated with aortic counterpulsation among those with hemodynamic compromise, such as in cardiogenic shock. There is a surprisingly limited amount of data on the hemodynamic effects of IABP. In a study of 41 patients with cardiogenic shock with acute myocardial infarction randomized to IABP vs. LVAD, the IABP group (n = 20) showed an increase in cardiac power index, from 0.22 (IQR 0.18–0.30) to 0.28 W/m2 (IQR 0.24–0.36), cardiac output, from 3.0 (2.5–4.0) to 3.3 (2.9–4.3) L/min; and mean arterial pressure, from 64 (57–74) to 67 (62–84) mm Hg; and a decrease in pulmonary capillary wedge pressure, from 27.0 (20.0–30.0) to 21.5 (17.0–26.0) mm Hg. This study found that a percutaneous LVAD (Tandem HeartTM, Cardiac Assist, Pittsburgh, PA, USA) provided more hemodynamic support but resulted in more complications [1]. The IABP Cardiogenic Shock Trial was a prospective, randomized, single‐center trial looking at the effects of IABP vs. inotropic therapy (i.e., dobutamine and/or norepinephrine) in 40 patients with acute myocardial infarction complicated by cardiogenic shock, treated by PCI within 12 hours of onset of hemodynamic instability. There was significant improvement in both groups in cardiac output, systemic vascular resistance, and cardiac power output, and no significant differences between the IABP group and the medical‐alone group in cardiac output or systemic vascular resistance after 24, 48, 72, or 96 hours [2]. To achieve optimal effects of counterpulsation, inflation and deflation need to be correctly timed to the patient’s cardiac cycle. This can be accomplished by using the patient’s ECG signal, the patient’s arterial waveform, or an intrinsic pump rate. The most common method of triggering the IABP is from the R wave of the patient’s ECG signal (Figure 21.3). On identification of the R wave the balloon deflates. Balloon inflation is generally set to start automatically in the middle of the T wave. Tachyarrhythmias, cardiac pacemakers, and poor ECG signals may cause difficulties in obtaining synchronization when the ECG mode is used. In such cases the arterial waveform may be useful for triggering. Figure 21.3 IABP set on a 1:2 ratio in a 60‐year‐old male with cardiogenic shock in the setting of acute myocardial infarction (200 mm Hg scale). For optimal efficacy, the IABP should inflate during early diastole (after the aortic valve closes, which is identified by the dicrotic notch) and deflate just prior to systole (just before the aortic valve opens). IABP inflation and deflation timing, as well as ratio of counterpulsations to cardiac cycles, can be adjusted to optimize performance (Figures 21.4 and 21.5). In general, optimal assistance from the IABP occurs when each cardiac cycle is augmented in a 1:1 ratio. In patients with tachycardia (e.g., rapid atrial fibrillation), a lower assist ratio (1:2 or 1:3) can be used to allow gas delivery to and removal from the balloon. Figure 21.4 Correct IABP timing and hemodynamic effects. Normal timing of the IABP (arrow) with inflation at the dicrotic notch (DN) and good diastolic augmentation, which increases coronary blood flow and increases mean blood pressure. Assisted systolic and end‐diastolic pressures are lower than unassisted systolic and end‐diastolic pressures. Figure 21.5 Aortic pressure with and without IABP. Aortic pressure waveforms prior to (a) and after initiation of IABP at 1:2 ratio (b). [DN = dicrotic notch] The most common cause of inadequate balloon counterpulsation is the inaccurate timing of inflation and deflation. Some errors associated with IABP timing will simply result in poor hemodynamic response to IABP, but others are more dangerous for patients with already tenuous cardiovascular situations. Understanding IABP pressure waveforms is critical to use this device properly. The timing errors can be divided into two groups: systolic errors (early and late inflation) and diastolic errors (early and late deflation). Inflation of the IABP prior to aortic valve closure will increase afterload, since the heart must eject blood against an inflated balloon. This increases myocardial wall stress and LVEDP, thereby increasing LV work, reducing cardiac output, and potentially precipitating early closure of the aortic valve. On the hemodynamic tracing, a sharp or rapid rise in the augmented pressure will be seen prior to the dicrotic notch (Figure 21.6). Figure 21.6 Incorrect IABP timing—early inflation. Aortic pressure waveform demonstrating early inflation of the IABP (arrow) prior to the dicrotic notch (DN) and prior to the closure of the aortic valve. There is a rapid rise in augmented diastolic pressure (*) with the aortic valve still open, which causes a dramatic increase in afterload and reduces cardiac output. Although probably the least dangerous error, late IABP inflation can preclude the patient from obtaining optimal hemodynamic support. Here, the IABP inflates too long after the aortic valve closes. This delay does not allow for adequate diastolic augmentation. There is a blunting or complete lack of the diastolic augmentation waveform (Figure 21.7). Associated with this is a lack of end‐diastolic pressure decrease (afterload reduction) and no increase in diastolic perfusion pressure. Figure 21.7 Incorrect IABP timing—late inflation. Aortic pressure waveform demonstrating late inflation of the IABP. The notable hemodynamic changes are the clearly visible dicrotic notch (DN) with the IABP inflation following (arrow). There is a prolonged dip or U wave (*), then a blunted or decreased diastolic augmentation. Early deflation, similar to late inflation, is not a particularly dangerous situation, although the patient will not receive optimal balloon counterpulsation. The hemodynamic tracing reveals a normal diastolic augmentation, but then a long‐drawn‐out U‐shaped wave prior to the assisted systolic waveform (Figure 21.8). Notice that there is inadequate preload reduction and therefore no change in assisted and unassisted systolic pressure. Simply prolonging the balloon inflation will quickly improve the hemodynamic support that the balloon pump can provide. Figure 21.8 Incorrect IABP timing—early deflation. Aortic pressure waveform demonstrating early deflation of the IABP. Early deflation of the IABP in diastole does not affect the diastolic augmentation, but does prevent optimal end‐diastolic pressure decrease. This is evident by a prolonged dip and U wave (*) post augmentation. There is no reduction in assisted systolic pressure, which is equal to the unassisted systolic pressure, representing a lack of afterload reduction. Large arrow denotes beginning of IABP inflation. Late IABP deflation is potentially the most critical timing error. If the balloon remains inflated when the left ventricle enters systole, the left ventricle is forced to contract against the inflated balloon. The result can be acute hypotension and cardiac arrest. The hemodynamic tracing reveals a long inflation time with an elevated end‐diastolic pressure and a severely reduced assisted systolic pressure (Figure 21.9). Figure 21.9 Incorrect IABP timing—late deflation. Aortic pressure waveform demonstrating late deflation of the IABP. Late deflation of the IABP in diastole causes a dramatic increase in afterload, since systole begins with higher intra‐aortic pressure. This causes late opening of the aortic valve and severely reduces the cardiac output. The pressure waveform reveals an assisted end‐diastolic pressure that is higher than the unassisted end‐diastolic pressure and a drastically reduced assisted systolic pressure. Large arrow denotes beginning of IABP inflation. The IABP is a widely used device in the management of patients suffering from the ill effects of acute and chronic cardiovascular disease. Understanding the characteristic hemodynamic tracings of balloon counterpulsation is critical for proper functioning and ensuring the maximal therapeutic benefit. In turn, recognizing the possible complications and pitfalls of IABP timing is crucial to minimizing potential adverse effects. Since IABP is widely used, it is surprising that there are so few data demonstrating clinical efficacy. Studies in high‐risk PCI, acute MI complicated by cardiogenic shock, and acute MI without cardiogenic shock have shown no benefit to IABP use [3,4].
Hemodynamics of intra‐aortic balloon counterpulsation
History and uses
Complicated acute myocardial infarction
Refractory unstable angina
Cardiogenic shock (including right ventricular failure)
Mechanical complications of acute myocardial infarction or trauma
Severe coronary artery disease with hemodynamic compromise
Left main disease
Support of high‐risk coronary interventions
Induction and weaning of cardiopulmonary bypass
Bridge to cardiac transplantation
Refractory ventricular arrhythmias
Noncardiac surgery for high‐risk cardiovascular patients
Severe mitral regurgitation
Severe peripheral vascular disease
Active hemorrhage
Severe thrombocytopenia
Contraindication to anticoagulation
Moderate to severe aortic insufficiency
Description
Hemodynamic effects
Decreased left ventricular afterload
Decreased aortic SBP
Decreased pulmonary capillary wedge pressure
Decreased LV volume
Decreased LV end‐diastolic pressure
Increased coronary blood flow
Increased cardiac output
Intra‐aortic balloon pump timing
Early inflation
Late inflation
Early deflation
Late deflation
Conclusion
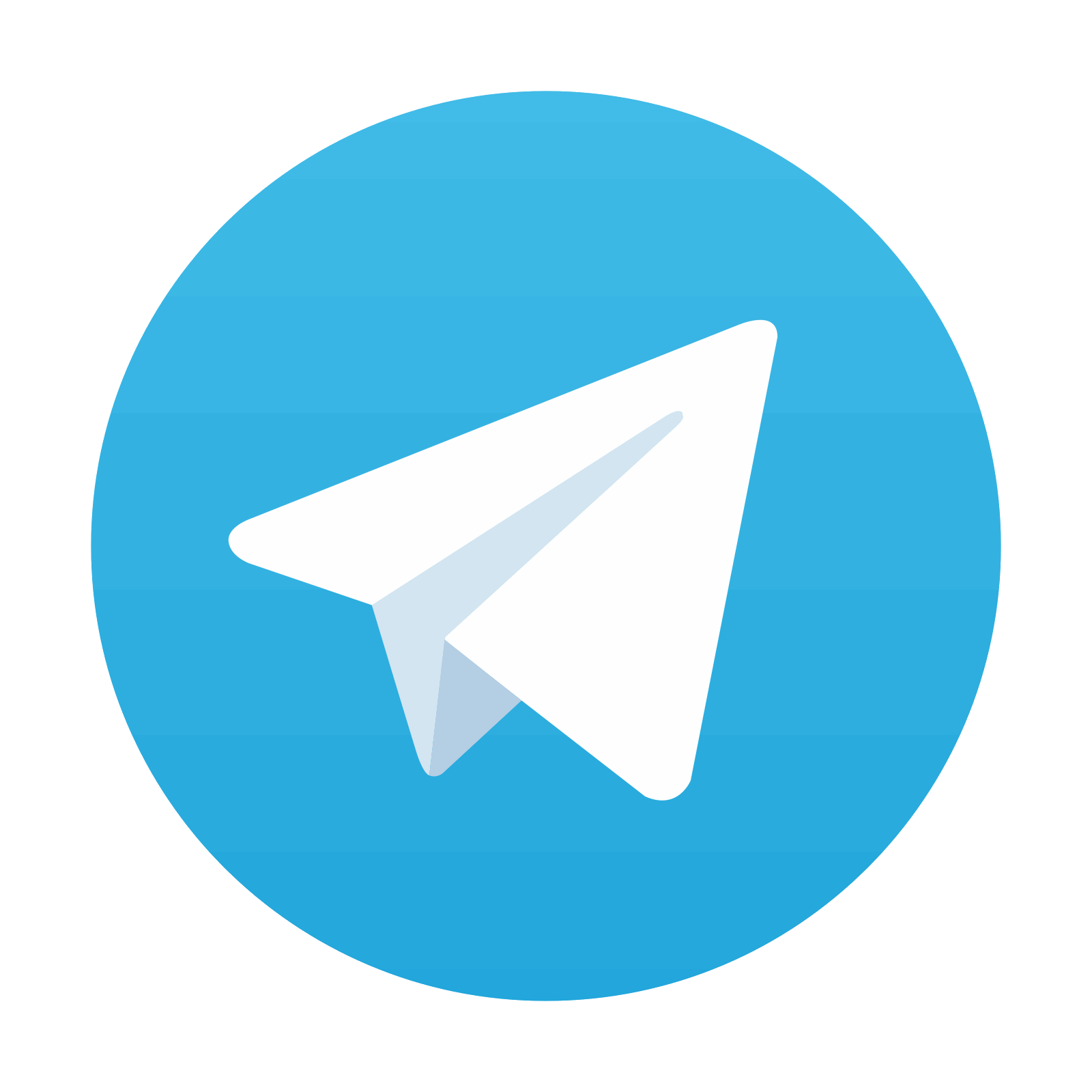
Stay updated, free articles. Join our Telegram channel

Full access? Get Clinical Tree
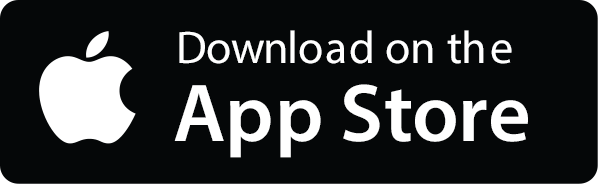
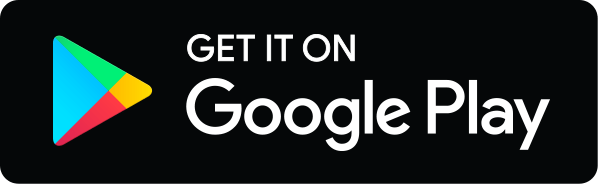