CHAPTER 16 Geoffrey T. Jao, Steven Filby and Patricia P. Chang Heart failure (HF) is a clinical syndrome caused by failure of the heart to meet the demands of the body, with symptoms due to elevated intracardiac filling pressures and/or decreased effective cardiac output. The most common classification of HF is based on the left ventricular ejection fraction (LVEF), as determined by some form of cardiac imaging (most commonly an echocardiogram). The LVEF cutoff for HF with reduced ejection fraction (HFrEF, also known as systolic heart failure) is <50%, or more strictly <40%. Patients with LVEF >50% are considered to have HF with preserved ejection fraction (HFpEF, previously known as diastolic heart failure). While these two entities are indistinguishable based on clinical signs and symptoms alone, they differ in all other aspects: gross anatomy, histology, radiographic and hemodynamic findings, and, not surprisingly, treatment effects. The etiology of HF is due to some form of myocardial insult (Table 16.1), with HFrEF better understood than HFpEF. Table 16.1 Common etiologies of heart failure. The pressure–volume loops best illustrate the hemodynamic differences between HFrEF and HFpEF (Figure 16.1). Since patients with HFrEF have reduced myocardial contractility, the left ventricle dilates to accommodate a higher preload necessary to maintain an effective cardiac output. In hemodynamic terms, a patient with HFrEF has higher left ventricular end‐diastolic volume (LVEDV) and pressure (LVEDP), as well as lower stroke volume, pulse pressure, and effective cardiac output, which then translate to higher left ventricular end‐systolic volume (LVESV) and pressure (LVESP). As the degree of systolic dysfunction worsens, the pressure–volume loop further shifts toward the right. Likewise, in the presence of significant mitral regurgitation, the stroke volume and effective cardiac output are further reduced due to the absence of isovolumetric contraction. Figure 16.1 Left ventricular pressure–volume loops. Left panel: left ventricular pressure–volume loop demonstrates pressure and volume changes during the cardiac cycle: (a) the left ventricle fills during diastole, followed by (b) isovolumetric contraction at end‐diastole, which ends when the aortic valve opens (at the highest LVEDV and LVEDP), followed by (c) left ventricular ejection (systole), followed by (d) isovolumetric relaxation (beginning with the aortic valve closing). The slope of the end‐systolic pressure–volume relationship (ESPVR) represents end‐systolic elastance (Ees), which provides an index of contractility. The end‐diastolic pressure–volume relationship (EDPVR) represents the passive filling (compliance) for the LV, and the slope of this curve is ventricular stiffness (the reciprocal of ventricular compliance). Stroke volume is the difference of LVEDV and LVESV (SV = EDV – ESV). Pulse pressure is represented as the difference of LVESP and LVEDP. Right panel: pressure–volume loops for normal (solid line), HFpEF (thin dash line), and HFrEF (bold dash line). The exact mechanism causing HFpEF is still unknown. An autopsy series of patients with HFpEF showed more cardiac hypertrophy, epicardial coronary artery disease, coronary microvascular rarefaction, and myocardial fibrosis compared to age‐matched control subjects [1]. In contrast to HFrEF, the resulting “stiffer” left ventricle develops higher filling pressures given the same incremental changes in volume (Figure 16.1). Thus, a much lower LVEDV is needed to overcome the aortic pressure, resulting in reduced stroke volume, effective cardiac output, and LVESV. LVEF, defined as the ratio of stroke volume to LVEDV, is preserved because both the numerator and denominator are reduced. History, physical examination, cardiac biomarkers, chest X‐ray, and occasionally cardiac imaging are central in the medical management of acute on chronic heart failure. In some cases it may be difficult to estimate accurately the true hemodynamic status of the chronic HF patient using these tools alone. Physical examination findings of HF can be absent in as many as 40% of patients with elevated pulmonary capillary wedge pressures (PCWP) [2, 3]. Additionally, the chest X‐ray often does not show evidence of pulmonary congestion in advanced HF patients due to increased pulmonary lymphatic drainage [4]. In this light, judicious utilization of right heart catheterization can be very helpful in some clinical scenarios. It is noteworthy that routine pulmonary artery catheter (PAC) use has never been shown to improve mortality or long‐term clinical outcomes in patients with HF [5–12]. In the randomized, multicenter Evaluation Study of Congestive Heart Failure and Pulmonary Artery Catheterization Effectiveness (ESCAPE) trial, the addition of routine PAC to clinical assessment, compared to clinical assessment alone, did not improve clinical outcomes in patients with severe symptomatic and recurrent systolic heart failure [12]. Clinical status significantly and continuously improved in both groups, but there was a consistent trend suggesting greater clinical improvement in the PAC group (especially at the 1‐month but not at the 6‐month follow‐up). Not surprisingly, inpatient mortality and survival at 6 months were similar in both groups and adverse in‐hospital events (driven by PAC infection) were higher in the PAC group. As is true for most advanced HF patients, improvements in functionality and quality of life are more meaningful than prolongation of life. Thus, PAC can be useful for optimizing hemodynamic management of the decompensated HF patient when clinically indicated. Table 16.2 lists some of the indications for right heart catheterization. Table 16.2 Indications for right heart catheterization in patients with heart failure. The hemodynamic findings of HFrEF and HFpEF are often similar in terms of elevated intracardiac filling pressures, but decreased effective cardiac output is usually seen only in advanced HFpEF, whereas it is seen earlier in HFrEF. Patients with chronic HF usually have higher baseline intracardiac pressures than those with acute HF, because chronic HF patients typically require higher filling pressures to maintain optimal cardiac output (as illustrated by the Frank–Starling relationship, Figure 16.2). Thus the “normal” parameters are slightly higher for chronic HFrEF patients than the “ideal” normal range for patients with normal cardiac function. Specific conditions such as restrictive cardiomyopathy, constrictive pericarditis, and hypertrophic cardiomyopathy will be discussed elsewhere in this book. Figure 16.2 Frank‐Starling curve of cardiac output as a function of LVEDP. Idealized Frank–Starling curves with varying degrees of left ventricular contractile dysfunction. In the normal heart, an increase in preload results in a steep increase in cardiac output. With left ventricular systolic dysfunction, the curves are displaced down and rightward, such that higher filling pressures are needed to maintain the same amount of cardiac output. The best measurement of LV preload is left atrial (LA) pressure. In practice, LA pressure is difficult to obtain and thus two surrogate measurements are commonly used (Figure 16.3): left ventricular end diastolic pressure (LVEDP) and pulmonary capillary wedge pressure (PCWP). Figure 16.3 Simultaneous left ventricular and pulmonary capillary wedge pressures in a 59‐year‐old female. [LVEDP = left ventricular end diastolic pressure.] Since LVEDP is measured at the end of diastole, the major contribution is from atrial contraction for patients in sinus rhythm. While it is noteworthy that the end‐diastolic pressure–volume relationship (EDPVR) is curvilinear (Figure 16.1), the more severe the degree of systolic and diastolic dysfunction, the less likely this generalization is to hold. In HFrEF where the left ventricular chamber size is dilated, increased LVEDV is needed to produce a proportionate increase in LVEDP, which in turn is needed to optimize cardiac output. In contrast, the abnormal left ventricular relaxation or ventricular stiffness in HFpEF creates a stiff and poorly compliant LV, thus a relatively small change in LVEDV leads to elevation in LVEDP. Direct measurement of the LVEDP is achieved by placing a catheter into the left ventricle via arterial access (Figure 16.3). The PCWP profile is different in acute and chronic HF patients. For the patient who sustained an acute myocardial injury, for example secondary to sudden complete occlusion of the proximal left anterior descending artery with resultant myocardial dysfunction, a sudden rise in PCWP to 17–20 mm Hg could be sufficient to cause acute pulmonary edema. In contrast, a patient with chronic HF often needs a mean PCWP of 17–20 mm Hg to optimize cardiac output, as explained by the Frank‐Starling mechanism (Figure 16.2). The PCWP waveform in HF patients is often characterized by a prominent V wave (Figure 16.4). This V wave morphology may be due to a noncompliant LV or LA, and/or due to moderate to severe functional mitral regurgitation (from left ventricular dilatation), often seen in HFrEF. The calculated mean PCWP will be elevated and not accurately reflective of LV preload, as the increased pressure in the V waves is transmitted to the pulmonary vessels. Figure 16.4 Pulmonary capillary wedge pressure tracing in a patient with dilated cardiomyopathy and severe mitral regurgitation. The mean PCWP is elevated at approximately 25 mm Hg and prominent V waves are also noted (arrow). Pulmonary hypertension (PH) is defined as mean pulmonary artery pressure (mPAP) >25 mm Hg. World Health Organization Group 2 refers to PH due to left heart disease (i.e., pulmonary venous hypertension or post‐capillary PH) [13], and is by far the most common cause of PH and right‐sided HF. Up to 60% of patients with severe systolic dysfunction and up to 70% of patients with isolated LV diastolic dysfunction may present with PH [14]. PH carries a poor prognosis for patients with chronic heart failure [15]. PH is further discussed in Chapter 26. The PA diastolic pressure should correlate well with the PCWP in the absence of significant pulmonary vasculature remodeling. It is important to mention that the mPAP is used to calculate the transpulmonary gradient, pulmonary vascular resistance, and the right ventricular stroke work index, all of which are important parameters in deciding eligibility for ventricular assist device implantation or heart transplantation. In the absence of pulmonic stenosis, the RV systolic pressure should correlate well with the PA systolic pressure. The RV diastolic pressure should also correlate well with the mean right atrial pressure in the absence of pathologic tricuspid regurgitation. An elevated RV systolic pressure usually indicates preserved RV myocardial contractility. One needs to consider RV failure if the RV systolic pressure is low in the setting of elevated right atrial pressure (>15 mm Hg) and depressed cardiac index (<2.0 mL/min/m2) [13]. Examination of the jugular venous pulse and sonographic assessment of the jugular vein and inferior vena cava are noninvasive estimates of right atrial pressure (RAP). Typically, the mean PCWP is greater than the mean RAP in chronic HF and in normal hearts. Tricuspid regurgitation can raise the mean RAP above the mean PCWP and show a prominent V wave on the RA waveform. In patients with predominantly right‐sided HF, the mean RAP can dominate the right atrial waveform with a prominent V wave and steep Y descent. The mixed venous oxygen saturation (SvO2) is a marker of adequacy of tissue perfusion and decreases when oxygen delivery falls or tissue oxygen demand increases. It is directly measured in a blood sample obtained from the pulmonary artery. SvO2 in HF patients is usually decreased, often less than 60%, and may be as low as 30% in severely decompensated patients. Measurement of SvO2 is useful to monitor progression of disease in HF patients and has been proposed as an easy alternative to the more conventional hemodynamic parameters (i.e., cardiac output) to estimate hypoperfusion, as SvO2 is a critical component of the Fick equation for derivation of cardiac output. SvO2 is directly related to cardiac output, hemoglobin, and arterial saturation and inversely related to the metabolic rate. By using the derivation of the Fick equation, the mixed venous saturation can be determined by:
Heart failure
Coronary artery disease
Hypertension
Primary valvular disease
Tachycardia‐mediated cardiomyopathy
Toxins (ethanol, anthracyclines, her2‐neu receptor antagonist, and tyrosine kinase inhibitors)
Stress‐induced cardiomyopathy (aka Takotsubo cardiomyopathy)
Congenital heart disease
Infectious (HIV, Chagas’ disease, coxsackie, echovirus, Lyme disease)
Infiltrative disease (hemochromatosis, amyloidosis, sarcoidosis)
Peripartum cardiomyopathy
Myocarditis (giant cell myocarditis, lymphocytic myocarditis, eosinophilic myocarditis/hypersensitivity)
Connective tissue disease (systemic lupus erythematosis)
Nutritional deficiencies (selenium, copper)
Metabolic (thyroid disease)
Hereditary (neuromuscular disorders [Duchenne’s muscular dystrophy], X‐linked, mitochondrial [MELAS syndrome], storage disease)
High‐output states (arteriovenous fistula, hyperthyroidism, beriberi)
Hypertrophic cardiomyopathy
Restrictive cardiomyopathy
Idiopathic
Determination of true hemodynamic/intravascular status when noninvasive clinical evaluation alone is insufficient
Initiation of and dose optimization of inotropes and/or vasodilators
Perioperative monitoring and management of the high‐risk patient undergoing cardiothoracic surgery
Gold standard for diagnosis and management of pulmonary hypertension
Hemodynamic monitoring, including evaluation of pulmonary vascular resistance, of end‐stage HF patients awaiting cardiac transplantation
Differentiation of constrictive pericarditis from restrictive cardiomyopathy (together with left heart catheterization)
Determination of valvular heart disease severity when other diagnostics (e.g., echocardiography) are equivocal
Directly measured intracardiac pressures
Left ventricular end‐diastolic pressure
Pulmonary capillary wedge pressure
Pulmonary artery pressure
Right ventricular pressures
Right atrial pressure (normal 2–8 mm Hg)
Derived parameters from measured intracardiac pressures
Mixed venous oxygen saturation (normal ~75%)
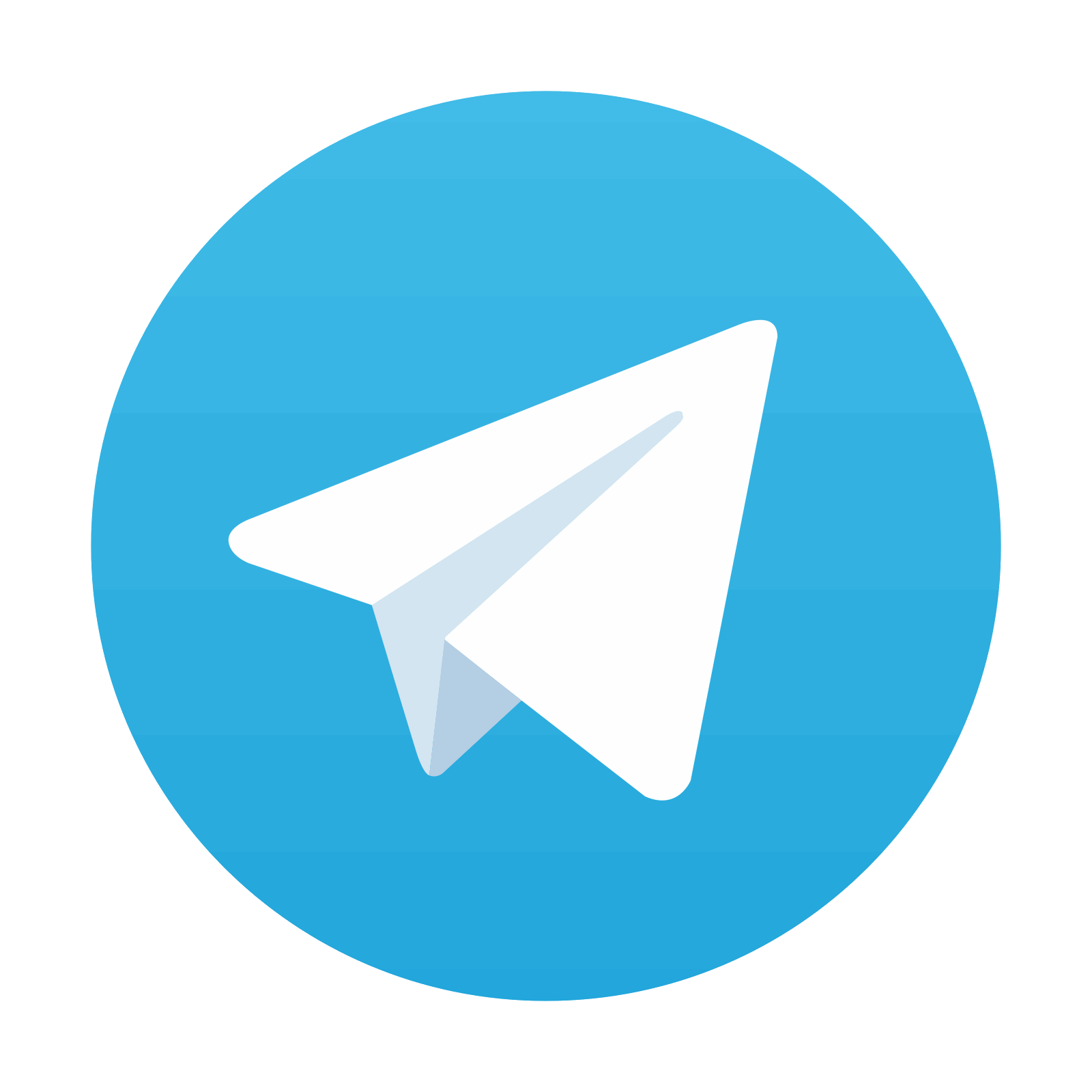
Stay updated, free articles. Join our Telegram channel

Full access? Get Clinical Tree
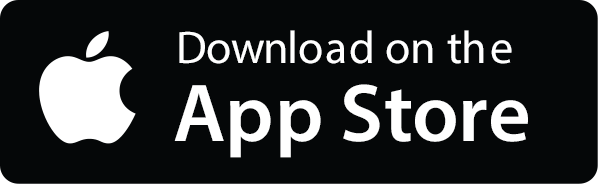
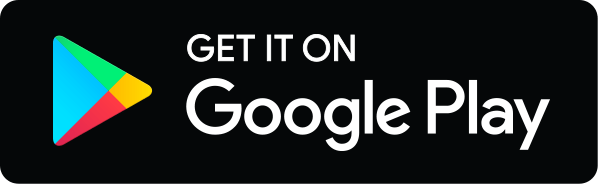