CHAPTER 15 Jayadeep S. Varanasi and George A. Stouffer Hypertrophic cardiomyopathy (HCM) is the name given to a heterogeneous family of disorders characterized by genetic defects involving myocyte sarcomeric proteins. More than 1400 mutations in 11 genes encoding proteins have been described, with the most common defect being a mutation in the gene that encodes beta‐myosin heavy chain, but defects in other genes encoding for sarcomeric proteins including troponin T, troponin I, myosin light chains, alpha tropomyosin, and myosin‐binding protein C have been implicated in causing HCM. Inheritance is usually autosomal dominant with variable penetrance. Classically, HCM was defined by excessive septal hypertrophy (usually the width of the septum on echocardiography exceeding 1.5 cm) with or without the presence of outflow tract obstruction, and even today most studies of HCM use echocardiographic, rather than genetic, criteria to determine eligibility. Various microscopic features of HCM distinguish it from other diseases that cause left ventricular hypertrophy (LVH). The principal aspect of HCM is myocyte disarray in which cells lose their normal parallel arrangement. Individual myocytes often show variability in size and may form circular patterns around areas of fibrous tissue. Myocyte disarray can be seen in other illnesses; however, it tends to affect less of the left ventricle when caused by hypertension or aortic stenosis. In HCM, intramyocardial arteries are often obliterated, creating small areas of fibrosis within the ventricular wall, especially within the interventricular septum. On gross examination in patients with HCM, there is increased myocardial mass with a normal or small left ventricular chamber size. The disease usually affects the left ventricle more than the right ventricle, with the interventricular septum and anterolateral free wall being the most common segments involved. The hypertrophy is often asymmetric, with differing wall thicknesses noted in contiguous segments. Left atrial enlargement is common because of both impaired ventricular relaxation and mitral regurgitation. Along with fibrosis in the ventricular wall, a fibrotic plaque is sometimes seen on the interventricular septum and is thought to be the result of repetitive contact between the anterior mitral leaflet and the septum. “Burned‐out” HCM, in which there is LV wall thinning, chamber enlargement, and systolic dysfunction resembling dilated cardiomyopathy, develops in 5–10% of patients. Several anatomic variants of HCM have been described. The most well‐known phenotype from a hemodynamic standpoint is hypertrophic obstructive cardiomyopathy (HOCM; also known as idiopathic hypertrophic subaortic stenosis). It was formally described as a distinct clinical entity in 1958, and consists of narrowing of the left ventricular outflow tract (LVOT) with ventricular contraction causing a dynamic pressure gradient (Figure 15.1). This anatomic pattern occurs in 25–50% of cases of HCM and will be the type of HCM that we concentrate on in this chapter. Other anatomic forms of HCM that have been described include apical HCM [1], concentric hypertrophy, localized hypertrophy (e.g., posterior portion of septum, posterobasal free wall of the LV, or at the mid‐ventricular level), and HCM of the elderly, characterized by severe concentric LVH, a small LV cavity, and hypertension [2]. The vast majority of intraventricular pressure gradients are localized to the LVOT, although mid‐cavity obstruction has also been reported. Note that in the rest of this chapter HCM will refer to all types of hypertrophic cardiomyopathy, while HOCM will refer only to hypertrophic cardiomyopathy in which there is a pressure gradient in the left ventricle at rest or with provocation. Figure 15.1 Eccentric hypertrophy and LV outflow tract gradient in HOCM. HOCM is characterized by asymmetric septal hypertrophy (a) which causes an obstruction to LV outflow and a pressure drop in the LV outflow tract (b). The most feared complication of HCM is sudden cardiac death. HCM is one of the most common causes of sudden death in individuals between the ages of 12 and 35 and is also a common cause of death in young athletes. Not all patients with HCM are symptomatic, but in those who are, dyspnea, angina, and palpitations are common. Signs of LVH, such as an S4 and laterally displaced precordial impulse, may be present. Most patients with intraventricular gradients have a double or triple apical impulse. Atrial contraction may be noted as a presystolic apical impulse, as well as a prominent A wave in the jugular venous pulsation. The carotid pulse may display a “spike and dome” configuration. In patients with an LVOT obstruction, there is a harsh mid‐systolic murmur that commences well after the first heart sound and becomes louder with maneuvers that decrease LV size and filling pressures (e.g., standing from squatting, Valsalva, or dehydration). The murmur often decreases with handgrip exercise. A murmur of mitral regurgitation may be present as well. An understanding of the hemodynamic manifestations of HOCM can be facilitated by considering causative factors separately, while realizing that in a given patient there will be significant interactions between these factors. The four factors that will be discussed here are diastolic dysfunction, LVOT obstruction, mitral regurgitation, and abnormal coronary flow reserve (Table 15.1). Table 15.1 Hemodynamic findings in HOCM. Diastolic dysfunction is present, to some degree, in almost all patients with HCM. Diastolic dysfunction progresses as the ventricle hypertrophies and becomes less compliant. Higher pressures are required to fill the ventricle, leading to increased left atrial, pulmonary artery (PA), right ventricular (RV), and right atrial pressures. Progressive diastolic dysfunction results in clinical manifestations of congestive heart failure in some patients with HOCM. Outflow tract obstruction in patients with HOCM is “dynamic,” which means that the resistance to flow changes depending on filling pressures, afterload (e.g., aortic pressure), and force of contractility. LVOT obstruction changes in severity depending on the force of systolic contraction and the dimensions of the LV (in contrast to aortic stenosis, which is a fixed obstruction since it is valvular and not muscular). Thus, patients with HOCM may not have any obstruction while at rest, but may develop significant pressure gradients during any activity that increases the force of cardiac contraction (e.g., exercise, emotional stress) or at times when their LV is smaller because of incomplete filling (e.g., dehydration). This obstruction can cause pressure gradients of greater than 100 mm Hg and is thought to be one etiology of exercise‐induced syncope in these patients (another potential etiology is arrhythmias). LVOT gradients can be provoked using methods that decrease filling pressures and stroke volume (e.g., Valsalva maneuver or nitroglycerin) or methods that increase the force of contraction (post‐PVC or isoproterenol infusion). Dobutamine should be avoided, as it can cause subaortic pressure gradients in normal hearts due to catecholamine stimulant effects. Mitral regurgitation is thought to be caused by systolic anterior motion (SAM) of the mitral valve during mid‐systole, which interferes with normal valve closure. Mitral regurgitation is usually mild, but can become significant, especially in association with large subaortic pressure gradients. The magnitude of pressure gradients measured at cardiac catheterization correlates well with Doppler velocities and with the duration of SAM. Prolonged mitral valve septal coaptation (>30% of systole) is invariably associated with high LVOT gradients and mild to severe mitral regurgitation. Although the role of SAM in producing the gradient is controversial, there is a close relationship between the degree of SAM and the size of the LVOT gradient in patients with subaortic obstruction (as opposed to mid‐cavity obstruction) [3,4]. Coronary flow reserve, the ratio of the maximal to the resting coronary blood flow and thus an index of the ability to increase coronary flow under physiologic stress, is abnormal in most patients with HOCM. A study of 20 patients with HCM found higher resting coronary blood flow and lower coronary resistance in comparison to 28 controls. Most patients with HCM achieved maximum coronary vasodilation and flow at modest increases in heart rate. Higher heart rates resulted in severe myocardial ischemia, elevation in LV end‐diastolic pressure (LVEDP), and a decline in coronary flow [5]. The same group of investigators, in a subsequent study of 50 patients with HCM, found that patients with LVOT obstruction at rest had higher coronary flow and regional myocardial oxygen consumption at rest and with pacing compared to patients with HCM but without obstruction. In patients with obstruction, transmural coronary flow reserve was exhausted at a heart rate of 130 bpm. Interestingly, in patients without obstruction, myocardial ischemia occurred at a lower coronary flow than in patients with obstruction [6]. A more recent study comparing coronary blood flow in eight patients with symptomatic HCM to eight matched controls found that patients with HCM had higher resting coronary blood flow, lower coronary resistance, and lower coronary flow reserve. Patients with HCM also had abnormal phasic coronary flow characteristics. These results are consistent with the reduction of coronary flow reserve in patients with HCM being caused by near maximal vasodilation of the microcirculation in the basal state [7]. Cardiac catheterization is usually not needed to confirm the diagnosis, but occasionally patients with HCM will undergo catheterization for evaluation of chest pain or congestive heart failure. Pulmonary capillary wedge pressure (PCWP) is generally elevated in patients with HCM, even more so when there is significant mitral regurgitation. The A wave may be accentuated due to a stiff ventricle and the V wave can be increased, either from mitral regurgitation or reduced LA compliance. RV systolic and PA pressures are generally only mildly elevated, and cardiac output is preserved, until LV failure occurs. As an example of filling pressures in patients with HCM, a study of 20 patients with HCM (9 with significant outflow tract gradients) compared to 28 controls found higher LVEDP (16 ± 6 versus 11 ± 3 mm Hg) and mean PCWP (13 ± 5 versus 7 ± 3 mm Hg) [5]. LVEDP is generally elevated, but there are no pathognomonic findings for HCM in the LV pressure tracing. The rapid filling phase of LV may be significantly prolonged and a prominent atrial contraction wave may be present (Figure 15.2). The upstroke of the LV pressure tracing may have a notch that corresponds with the anterior leaflet of the mitral valve coming into contact with the septum because of SAM. Figure 15.2 LV tracings in a patient with HOCM. In panel (a), pressures are obtained at baseline. Note the elevated LVEDP, prominent A wave in LV tracing, and lack of an outflow tract gradient. Panel (b) was obtained after inhalation of amyl nitrate. LVEDP is increased and an A wave is prominent. Aortic pressure is decreased compared to basal conditions and a small outflow tract gradient is present. A variable finding in HOCM is that the dynamic nature of the outflow tract obstruction causes a “spike and dome” configuration of the aortic or peripheral pulse (Figure 15.3). This is known as a bisferiens pulse, a name derived from Latin bis (= two) + feriere (= to beat). The bisferiens pulse is characterized by an initial rapid rise in aortic pressure (spike), followed by a slight drop in pressure (dip), and then a secondary peak (dome), and is most prominent in central aortic pressure, but can also be transmitted to the carotids. It is enhanced by maneuvers that increase intraventricular pressure gradients (e.g., following a PVC or by the Valsalva maneuver), and in patients with obstruction only during provocation, a normal‐appearing pressure tracing will often be replaced by the spike and dome during provocative maneuvers. A bisferiens pulse should be not confused with the dicrotic pulse, a pulse with an exaggerated dicrotic wave. A bisferiens pulse is most commonly associated with HOCM, but can also be seen in severe aortic regurgitation. Figure 15.3 Aortic pressure in a patient with HOCM demonstrating the “spike and dome” configuration. A gradient can be demonstrated by comparing pressure in the LV apex (using an end‐hole catheter) to aortic pressure or by a slow pullback of the catheter through the LV (Figure 15.4). In the cardiac catheterization laboratory, dynamic outflow tract obstruction can be provoked by a variety of maneuvers, including methods that decrease preload and/or afterload (e.g., Valsalva maneuver, administration of amyl nitrate; Figure 15.5) or methods that increase the force of contraction (e.g., isoproterenol infusion or PVC; Figure 15.6). LV outflow obstruction can be reduced or eliminated by maneuvers that increase chamber size (e.g., hydration). Figure 15.4 LV to aortic pullback using a dual lumen end‐hole catheter during amyl nitrate administration in a patient with HOCM. Aortic pressure is measured continuously through the second lumen. Figure 15.5 Demonstration of a provocable gradient with inhalation of amyl nitrate. There is no left ventricular outflow tract gradient under basal conditions (a). Following inhalation of amyl nitrate, aortic pressure falls, and a gradient is apparent (b). Over time, aortic pressure continues to fall and the gradient increases (c). All tracings are recorded on a 200 mm Hg scale. Figure 15.6 Demonstration of a provocable gradient with PVCs during administration of amyl nitrate. The Brockenbrough–Braunwald–Morrow sign was originally described in 1961. This sign is present if the aortic pulse pressure falls in the first normal beat post PVC in HOCM (Figure 15.7). While originally thought to be specific for HOCM, it has since been described in some cases of aortic stenosis. Figure 15.7 Brockenbrough–Braunwald–Morrow sign. Pulse pressure falls in the first normal beat post‐PVC (arrows) in HOCM (a) but not in aortic stenosis (b). RV intraventricular gradient is common in infants and children with HOCM, but is rarely seen in adults. Maron [8] reported that RV obstruction was observed in 60% of infants with HOCM and that RV outflow tract obstruction was frequently greater than LV outflow tract obstruction. Since the initial development of ultrasonic evaluation of the heart, echocardiography has been the gold standard for diagnosing HOCM. The cardinal echocardiographic features of HOCM are LVH, an intraventricular pressure gradient, and SAM of the mitral valve. There is considerable variability in the degree and pattern of hypertrophy and in some patients there is variation in the extent of LVH from region to region. The finding of a thickened septum that is at least 1.3–1.5 times the thickness of the posterior wall when measured in diastole just prior to atrial systole has been a commonly used criterion for the diagnosis of asymmetric septal hypertrophy. An intraventricular pressure gradient as measured by continuous‐wave Doppler interrogation in the aortic outflow tract is present in some patients. Provocative maneuvers (e.g., inhalation of amyl nitrate, Valsalva maneuver) can be performed in the echocardiography laboratory. Some investigators have criticized these maneuvers as being nonphysiologic and advocated using echocardiography in conjunction with treadmill or bicycle exercise testing to determine provocable gradients. The American College of Cardiology/European Society of Cardiology Clinical Expert Consensus [9] has recommended classifying patients with HCM into one of three groups based on the representative peak instantaneous gradient as assessed with continuous‐wave Doppler: (a) resting obstruction—gradient under basal (resting) conditions equal to or greater than 30 mm Hg (2.7 m/s by Doppler); (b) provocable obstruction—gradient less than 30 mm Hg under basal conditions and equal to or greater than 30 mm Hg with provocation; and (c) nonobstruction—gradient less than 30 mm Hg at rest and with provocation. A resting outflow tract gradient of ≥30 mm Hg (measured by continuous‐wave Doppler) is an independent determinant of symptoms of progressive heart failure and death. Other common echocardiographic findings in HCM include normal systolic function, diastolic dysfunction with reduced LV compliance, and a mitral valve E/A ratio less than 1.0 (usually <0.8) and an enlarged left atrium [10]. Invasive treatments are reserved for patients who have severe refractory symptoms (class III or IV) despite medical treatment and intraventricular gradients of more than 50 mm Hg. The classic Morrow myectomy is performed through an aortotomy and involves resection of a relatively small amount of tissue from the proximal septum. Surgical resection of the hypertrophied septum has an initial success rate of 90% in decreasing symptoms and LV outflow obstruction. In a study of 64 patients who underwent transaortic myectomy, there was a sustained improvement in symptoms and a decrease in LVOT resting gradient from 73.2 ± 14.8 mm Hg to 13.6 ± 2.7 mm Hg at an average follow‐up of 4.6 years (4 months to 12 years) [11]. Another study of 20 patients who underwent either myectomy or mitral valve replacement as treatment for an outflow tract gradient found a sustained reduction in gradient, a decrease in basal coronary blood flow, and a decrease in coronary blood flow and myocardial oxygen consumption during rapid atrial pacing [12]. Alcohol septal ablation has been developed as a treatment for LV outflow obstruction due to septal hypertrophy. In this procedure, alcohol is injected into a septal branch of the left anterior descending artery, causing localized infarction of the septum. Alcohol septal ablation appears to elicit a sustained reduction in outflow tract gradient. In a study of 50 patients, septal ablation (mean creatine kinase value 413 ± 193 U/L) reduced LVOT gradients from 80 ± 33 to 18 ± 17 mm Hg after 4–6 months and to 17 ± 15 mm Hg after 12–18 months [13]. There is considerable controversy about proper patient selection for alcohol septal ablation and surgical myectomy. Current guidelines emphasize several important points: (a) both ASA and myectomy are effective at reducing LVOT gradient; (b) myectomy produces more complete gradient relief, particularly in patients <65 years of age; (c) residual gradients are more common after alcohol septal ablation [9,14]. Dual‐chamber pacing has largely been abandoned as a treatment option in HOCM because data from randomised, double‐blind, crossover trials failed to show any benefit. RV pacing causes the interventricular septum to move paradoxically, and in theory this should increase LVOT dimensions and thus reduce LVOT blood velocities, which results in less SAM of the mitral valve and less mitral regurgitation. While initially proposed in 1975, interest in AV sequential pacing for HCM was revived by a study of DDD devices in 84 patients with obstructive HCM and severe symptoms refractory to medical therapy [15]. Symptoms were abolished in 33.3% of the patients, reduced in 56%, and unchanged in 8%. At a mean follow‐up of 2.3 ± 0.8 years only four patients had to undergo a surgical procedure. In this study there was significant improvement in NYHA class, in exercise capacity, and in resting LVOT gradient. Subsequent studies produced less enthusiastic results and dual‐chamber pacing is rarely utilized as a treatment for HOCM. In a multicenter, randomized, double‐blind, crossover study of permanent DDD pacing in 48 patients with drug‐refractory HOCM, Maron et al. found an average reduction of outflow gradient of 40%, but no change in exercise capacity, peak oxygen consumption, or LV wall thickness in the overall group [16].
Hypertrophic cardiomyopathy
Physical exam
Hemodynamics
Spike and dome configuration of arterial pulse
On aortic pressure tracing, there is a rapid rise during systole, which is followed by a mild drop in pressure and then a secondary peak
Systolic intraventricular pressure gradient
Simultaneous intraventricular and aortic pressure tracings will show a difference in maximum systolic pressure at rest and/or with provocation
Diastolic dysfunction
LV end‐diastolic pressure will be elevated, the rapid phase of LV filling will be prolonged, and atrial contribution to LV filling will be accentuated
Brockenbrough sign
Aortic pulse pressure fails to widen during a post‐extrasystolic beat
Findings at cardiac catheterization
Left atrium or pulmonary capillary wedge pressure
LV pressure
Aortic pressure
Outflow tract gradient
Echocardiography
Septal reduction for refractory symptoms
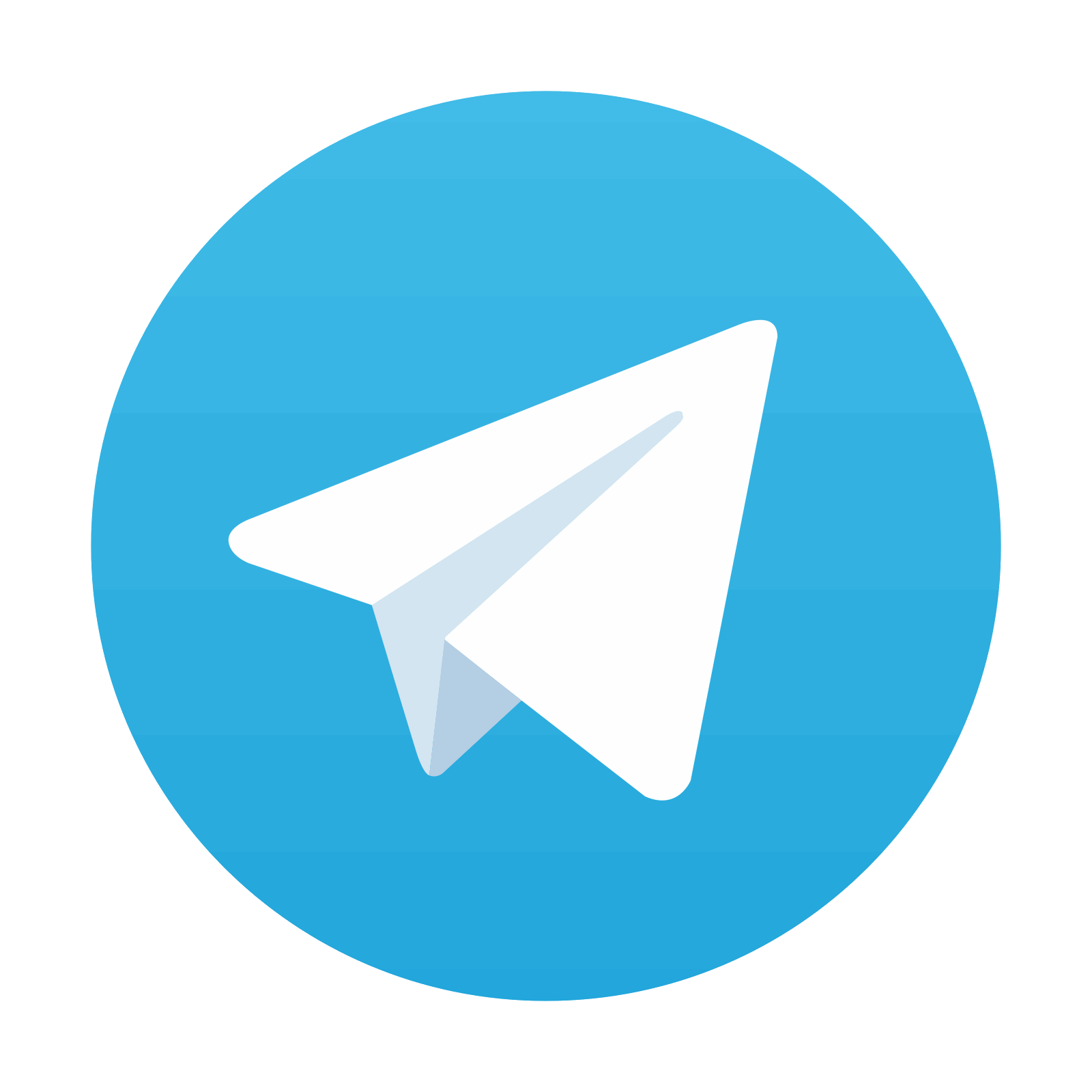
Stay updated, free articles. Join our Telegram channel

Full access? Get Clinical Tree
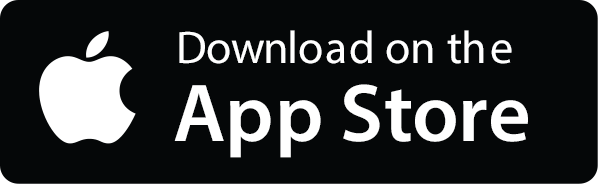
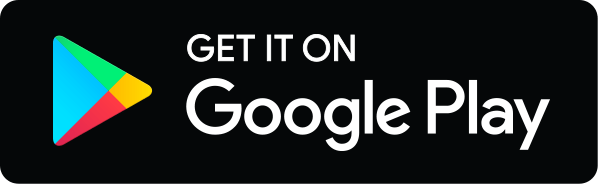