Chapter 157
Vascular Trauma
Thoracic
Ali Azizzadeh, Sharene Shalhub
Based on a chapter in the seventh edition by William T. Brinkman and Joseph E. Bavaria
Injury to the aorta and great vessels can occur following blunt and penetrating trauma. The majority of thoracic vascular injuries are caused by penetrating trauma secondary to gunshots, stab wounds, and iatrogenic injuries.1 Injury to the aorta, great vessels, pulmonary veins, and vena cava can also occur following blunt trauma, most commonly by motor vehicle accidents. Significant progress has been made over the last two decades in the diagnosis and treatment of thoracic vascular injuries. This paradigm shift includes the widespread use of computed tomography angiography (CTA) for diagnosis, aggressive blood pressure control, delayed repair for stable patients, adoption of endovascular repair as the treatment modality of choice for anatomically suitable candidates, and medical management of minimal aortic injuries. These advances have significantly improved the outcomes of patients with thoracic vascular trauma. This chapter summarizes the current diagnosis and management of thoracic vascular injuries.
Diagnostic Studies
Chest Radiograph
A supine anteroposterior chest radiograph should be obtained routinely in trauma patients. The treating physicians should be competent in interpreting supine portable chest radiographs because many patients are unable to have an “upright” posteroanterior study. Assessment of a supine chest radiograph can rapidly rule out significant pathology, such as pneumothorax, hemothorax, and fractures. If possible, an upright chest radiograph should be obtained. A normal upright chest radiograph has a negative predictive value of approximately 95%-98%.2
When presented with penetrating injuries, marking the entrance and exit sites with radiopaque markers is useful. Radiographic findings suggestive of penetrating great vessel injury include a large hemothorax; foreign bodies or their trajectory close to the great vessels; a confusing trajectory, which may indicate a migrating intravascular course; or a “missing” missile, suggesting embolization.5
Radiographic findings suggestive of blunt injury to the thoracic aorta include fractures of the sternum, scapula, clavicle, first rib, or multiple left-sided ribs. Indirect mediastinal clues include obliteration of the aortic knob, depression of the left main stem bronchus, loss of the paravertebral pleural stripe, an apical pleural cap, deviation of a nasogastric tube, and lateral displacement of the trachea at the T4 level.2 Pathologic widening of the mediastinum (Fig. 157-1), defined as more than 8 cm at the level of the aortic knob or a mediastinum width-to-chest width ratio exceeding 25%, has a reported sensitivity of 81%-100% and a specificity of 60%.3 As a result, a normal mediastinum on chest radiography does not exclude aortic injury. Moreover, determination of the presence of mediastinal widening has significant inter-reader variability, even among experienced radiologists. The radiologist’s overall impression of the mediastinum, rather than any one measurement, is a more sensitive predictor of traumatic aortic injury.4
Computed Tomographic Angiography
CTA has replaced angiography and transesophageal echocardiography (TEE) as the screening modality of choice for the diagnosis of traumatic aortic injury.5 Indications for chest CTA include an abnormal aortic contour on plain radiography, clinical signs, and injury mechanism suggestive of aortic injury. Compared with traditional angiography, CTA has a reported sensitivity of 86%-100%, specificity of 40%-100%, positive predictive value of 97%-100%, and negative predictive value of 94%-100%.6 This represents a dramatic change in the diagnosis of and screening for traumatic thoracic vascular injury. Traditional “step and shoot” CT scanners were not deemed acceptable screening tools due to slow acquisition, motion artifact, and poor spatial resolution.7 However, with the arrival of multidetector CT (MDCT), concerns about image acquisition time and spatial resolution have been minimized. At many trauma centers, CTA of the thorax is usually integrated into a whole-body CT “trauma pan-scan,” a technique that has been shown to improve survival and reduce imaging time.8,9 This allows for the diagnosis of vascular injuries and injuries elsewhere in the body. CT is widely available, cost effective, and can be used for operative planning (such as device sizing for endovascular repair) (Fig. 157-2).10
Figure 157-2 CTA of a patient with blunt thoracic aortic injury. A, Axial view demonstrating the injury and periaortic hematoma. B, Sagittal view demonstrating the typical location of the injury at the isthmus distal to the left subclavian artery. C, Three-dimensional CT reconstruction.
The protocol for multisystem evaluation usually includes nonionic contrast at 3-4 mL/s, for a total volume of 90-125 mL. Collimation of 3.2 mm with a pitch of 1.25 is used to reconstruct images at 1.6-mm intervals. Multiplanar reformulations are then used to evaluate vascular structures, including the aorta. Forty-eight cases of traumatic aortic injury were identified using this protocol at the Ryder Trauma Center over a 4-year period. All 48 cases were diagnosed using direct signs of injury, including active extravasation of contrast material, pseudoaneurysm formation, intimal flaps, and filling defects. Indirect signs of aortic injury included periaortic hematoma and mediastinal hematoma.11 Direct signs of aortic injuries were more accurate diagnostically than indirect signs.12 Traditionally, periaortic hematomas without direct signs on older generation helical CT were considered suspicious for aortic injury. Aortography was then recommended as a standard of care because there were reports of positive aortograms with no direct signs of aortic injury.13,14 Since the development of MDCT, the presence of periaortic hematomas in the absence of a direct sign of aortic injury does not necessarily require aortography. However, this depends on the confidence of the radiologist and the quality of the scanner and the study.15
Intravascular Ultrasound
Intravascular ultrasound (IVUS) provides real-time, 360-degree imaging of the aorta using a high-frequency (10 MHz) miniature ultrasound probe placed through a femoral arterial sheath (Fig. 157-3).16 IVUS is a useful tool for evaluation of traumatic aortic injury, especially in cases with equivocal CTA or in cases of periaortic hematomas in the absence of a direct sign of aortic injury.17 IVUS does not require contrast or radiation and can be performed concurrently using the same femoral puncture as angiography. In a recent study of 7,961 trauma admissions, we found that CTA was useful as a screening test in suspected thoracic aortic injury (TAI). However, when additional imaging was required after an equivocal CTA, IVUS was more sensitive than angiography. Equivocal results were more common with CTA images than with either IVUS or angiography (27% versus 2.5% or 5%, respectively; overall P = .0002). Compared with angiography, IVUS changed the diagnosis in 13% of cases—identifying injuries in 11% and ruling them out in 2%. Therefore, we advocate the use of IVUS in patients with suspected TAI in whom angiography is being considered.17 The disadvantages of IVUS are additional cost (capital equipment and disposable catheter), larger sheath, and operating room time.15 However, the information that it can provide can be invaluable in properly diagnosing TAI.
Angiography
Angiography, once considered the “gold standard” for diagnosis, remains a valuable imaging modality in the management of patients with thoracic vascular trauma. It has, however, taken on a therapeutic, rather than purely diagnostic, role given the wide utilization of CTA. Today, angiograms are more commonly performed in the operating room at the time of definitive repair. Disadvantages include invasive nature, cost, use of intravenous contrast, access site complications, and the need to transport the patient to an angiography suite or operating room (CTAs are commonly performed in the emergency center). There is a small incidence of false-positive angiograms from known anatomic abnormalities, such as ductus diverticulum and aberrant brachiocephalic arteries.18,19 Moreover, there is a significant interobserver variability in the interpretation in equivocal cases greater than that of IVUS.20,21
Transesophageal Echocardiography
TEE is a useful tool for the evaluation of traumatic aortic injury. TEE can be applied quickly at the bedside or in the emergency room while resuscitation is ongoing, and it allows diagnosis of associated cardiac injuries.22,23 In a meta-analysis of studies of TEE in the setting of traumatic aortic injury, the sensitivity and specificity of TEE was approximately 97%. In a direct comparison with aortography, TEE’s sensitivity and specificity were slightly lower (93% versus 95%).24 TEE performed better in patients with minimal aortic injuries because these aortic lesions can be missed by aortography due to a lack of aortic contour abnormality.25 Three trials followed patients with minor, nonsurgical aortic injuries. These studies found that TEE results were consistently positive, whereas in 10 of 12 patients, aortography or helical CT results were negative.17,19–22,24–26
The utility of TEE in the trauma setting is limited, because it is difficult to use in patients with neck injuries or when cervical spine “clearance” is pending. In addition, TEE does not visualize the ascending aorta or aortic branches well because of acoustic shadowing from the trachea and bronchi. Moreover, it requires specific training and expertise and may not be as readily available as CT or angiography. This can lead to significant time delays in a patient population in which rapid decisions are critical. Thus, TEE is now rarely used, as evidenced by a recent multicenter trial that showed only 1% of blunt TAI cases being diagnosed using this tool.5
Magnetic Resonance Imaging
Long examination times and limited access have hindered the application of magnetic resonance imaging (MRI) in patients with thoracic vascular trauma. However, with the development of fast MRI techniques (requiring only a few minutes), it can now be used, even in critically ill patients. The use of MRI to detect traumatic aortic rupture in comparison with angiography and CT (not MDCT) was reported in a series of 24 patients. The diagnostic accuracy was 100% for MRI, 84% for angiography (two false-negatives), and 69% for CT (two false-negatives and three false-positives).27 MRI is most useful for detecting the hemorrhagic component of a traumatic lesion. An aortic tear limited to the anterior or posterior wall can be visualized and followed if nonoperative management is chosen. Determination of the extent of aortic hematoma may be of prognostic significance. MRI is currently not commonly used to evaluate acute injury to the thoracic great vessels. However, when an aortic injury is being followed nonoperatively, MRI can be an excellent tool. This is especially true if there is a contraindication to CTA, such as renal insufficiency.28
Blunt Thoracic Aortic Injury
Incidence
Based on a recent analysis of the National Trauma Databank, the overall incidence of blunt thoracic aortic injury (BTAI) is 0.3%.29 After head injury, aortic injury is the second-most common cause of death, after blunt trauma.30 Contemporary autopsy studies of blunt trauma patients have reported aortic rupture in 21%-34%.31,32 Based on the National Vital Statistics Report, there were 37,661 motor vehicle deaths in 2010, of which an estimated 12,553 (33%) had BTAI.33 As a comparison, there were 10,397 deaths from aneurysms and dissections in the same year. In large part, traumatic BTAI is a condition of modern society. In 1958, Parmley et al37 first emphasized the lethality of BTAI and reported an 85% mortality rate at the accident scene. Of the patients who arrived at the hospital alive, most died from aortic rupture within a few days. In contemporary series, motor vehicle crashes remain the most common mechanism of injury (>70%) followed by motorcycle accidents, automobile-pedestrian accidents, and falls.30,34
Pathology
The entire aorta, from the aortic root to the aortic bifurcation, can be susceptible to BTAI. The most common site, however, is in the proximal descending aorta at the isthmus (Fig. 157-4). Most patients have a single injury, but there are examples of multiple BTAIs in a single patient.35 Autopsy series report that 36%-56% of BTAIs occur at the aortic isthmus, 8%-27% at the ascending aorta, 8%-18% at the arch, and 11%-21% at the distal descending aorta.32,36–39 However, most surgical series demonstrate a much higher percentage of aortic isthmic injuries—80%-100%—with only 3%-10% in the ascending aorta, arch, or distal descending aorta. This discrepancy may suggest that many patients with ascending or arch injuries die before intervention. Patients who arrive at the hospital alive have generally sustained incomplete, noncircumferential lesions to the media and intima. The tunica adventitia and mediastinal pleura then prevent free rupture. Remarkably, there are examples in the literature of patients with complete aortic transections who survived long enough to allow intervention.40,41
BTAIs represent a spectrum of lesions that range from intimal tears to free rupture. Classification is based on the extent of injury to the anatomic layers of the aortic wall. Based on imaging, BTAI is classified into four grades: intimal tears (grade I), intramural hematoma (grade II), pseudoaneurysm (grade III), and rupture (grade IV) (Fig. 157-5).20,42 Grade I injuries do not cause an external aortic contour abnormality and are best visualized on CTA or IVUS. Angiograms in grade I patients can be interpreted as normal. Injuries involving the media, such as intramural hematomas or dissections, are considered grade II. Grade II injuries cause an abnormality in the aortic contour and can be visualized on CTA, IVUS, or angiography. Pseudoaneurysms and ruptures (grade III and IV) are easily visualized with all imaging modalities. Additional classification systems based on contemporary imaging have also been proposed.20,43–47 Accurate diagnosis (grade) of BTAI is critical because it affects management decisions and is an important tool in evaluating outcomes.
Pathogenesis
Several different mechanical forces act in the pathogenesis of BTAI. The relative importance of these forces remains a subject of debate. The earliest proposed mechanism of BTAI was “stretching” of the aortic wall. This was supported by tensile test data, which demonstrated the inherent weakness of the isthmus and the relative immobility of the fixed distal descending aorta, relative to the ascending aorta and arch. The isthmus lies at the junction of these two segments. In contrast, some data show that the aortic wall is capable of sustaining strains up to 80% before rupturing. Therefore, the area stretched must be very localized to reach the required strain within the thoracic cage.48 Others have proposed a sudden rise in blood pressure as the cause of BTAI. A number of investigators have dismissed this, rationalizing that an isotropic cylinder under pressure ruptures longitudinally rather than transversely. Transverse tears (as seen with BTAI) occur only if the transverse strength is more than twice the longitudinal strength.40 Tensile data from Mohan et al48 on aortic wall samples demonstrated that the transverse-to-longitudinal strength was almost the required 2 : 1 ratio. In addition, their test samples consistently failed in a transverse manner. However, these bench tensile data had little clinical relevance. In 1956, Zehnder (as cited in the Kirklin et al text49) calculated that an intravascular pressure of 2,500 mm Hg was necessary to produce rupture of the aorta.
Osseous pinch is another hypothesis by Crass et al,50 who maintained that aortic rupture was due to entrapment of the aorta between the anterior thoracic bony structures and the vertebral column. A similar mechanism was reported by Symbas,51 who proposed that the aorta was forced onto and stretched over the spine in high-impact injuries. Another hypothesis is the “water-hammer” effect, which states that when the flow of a noncompressible fluid is suddenly obstructed, a high-pressure reflected wave is generated. If, during a vehicle impact, the aorta is suddenly occluded at the diaphragm, a reflected wave would be generated. The pulse pressure generated by this reflected wave would be greatest at the aortic arch because of the curvature reflection and further intensification. However, this phenomenon has only been studied in simple analytic models.52 Symbas53 concluded that torsion stress and stress from the water-hammer effect were most likely responsible for injuries to the ascending aorta, and bending and shearing stresses affected the aortic isthmus.
Finally, the Archimedes lever hypothesis suggests that lacerations of the aortic isthmus secondary to lateral impact motor vehicle crashes are due to the impacting vehicle’s force transmitted to the patient’s chest wall. This, in turn, initiates a rise in the intra-aortic pressure, which causes the entire anatomic unit of the proximal aorta to act as a single rigid Archimedes lever system. The fulcrum of this system is the insertion of the great vessels on the aortic arch, in particular, the left subclavian artery, which is least elastic because of its fascial binding to the first rib. In this system, the long arm of the Archimedes lever system is the ascending aortic arch, and the short arm is the aortic isthmus, which can then torque on the fixed junction of the descending aorta. This produces a transverse shearing stress and strain force on the free isthmus adjacent to the fixed portion of the proximal descending aorta, where the real-life aortic rupture occurs.54,55 The most likely explanation is that multiple forces, including shear, torsion, and stretch combined with hydrostatic forces cause BTAI (Fig. 157-6).40
Clinical Presentation
BTAI is caused primarily by motor vehicle accidents and falls. Motor vehicle crashes account for approximately 80% of BTAIs. Head-on collisions are the most common mechanism, but side- and rear-impacts can also cause BTAI. Patients with BTAI tend to be young (mean age, 39 years). Substance abuse is common and is a factor in more than 40% of the motor vehicle crashes that result in aortic disruption. Seat-belt use decreases risk by a factor of four. Ejection from a vehicle doubles the risk of aortic disruption.56 For drivers and front-seat passengers, steering wheel deformity is an independent predictor of serious thoracic injury.57 Even the deployment of air bags in automobile crashes has been associated with BTAI—in some instances, at speeds as low as 10 mph. Falls that result in aortic injury are generally from a height of 3 m or greater.
Table 157-1 lists the frequency of injuries associated with BTAI, based on data compiled from the 1970s to the late 1990s. The American Association for the Surgery of Trauma (AAST) compiled prospective data on 274 cases of BTAIs from 50 trauma centers throughout the United States and Canada. It reported that 50% of patients with BTAIs had associated brain injury. Other series reported that the occurrence of associated brain injuries was as low as 30%.58 Associated chest injuries, pelvic or long-bone fractures, and severe abdominal injuries were also frequently reported.
Table 157-1
Injuries Associated with Blunt Aortic Injury
Injury | No. of Patients (N = 274) (%) |
Closed head injury | 140* (51) |
Multiple rib fractures | 123 (46) |
Flail chest | 34 (12) |
Pulmonary contusion | 103 (38) |
Myocardial contusion | 10 (4) |
Diaphragm rupture | 20 (7) |
Splenic injury | 39 (14) |
Liver injury | 61 (22) |
Small bowel injury | 19 (7) |
Other abdominal injury | 38 (14) |
Spinal cord injury | 10 (4) |
Pelvic injury | 84 (31) |
Femoral injury | 67 (24) |
Tibial injury | 60 (22) |
Upper extremity injury | 54 (20) |
Maxillofacial injury | 36 (13) |
Cervical spine injury | 12 (4) |
Thoracic spine injury | 11 (4) |
Lumbar spine injury | 10 (4) |
None | 0 (0) |
* Thirty-four patients (24%) had intracranial hemorrhage.
From Fabian T, et al: Prospective study of blunt aortic injury: multicenter trial of the American Association for the Surgery of Trauma. J Trauma 42:374-380, 1997.
Diagnosis
All patients with thoracic trauma should have a chest radiograph. Surgeon-performed subxiphoid pericardial ultrasound to evaluate for pericardial fluid should also be routinely performed during the initial trauma evaluation. If there is suspicion of a vascular thoracic injury based on the mechanism of injury, physical examination, or any diagnostic finding, the next test is a CTA of the chest. Many times, CTA of the chest is combined with head and abdominal CTA or integrated into a whole-body CT trauma pan-scan. It is best to minimize the patient’s time in the radiology suite during the diagnostic evaluation in the emergency department.
Management
The first successful repair was accomplished by Klassen in 1957.59 Immediate repair was recommended once the diagnosis of a blunt or penetrating aortic injury was made. This practice began with the landmark paper by Parmley,37 who analyzed 296 BTAI cases among victims of the Korean War. Most important was the observation that of the 38 patients who made it to the hospital alive, 23 died of rupture within seven days of admission. In his conclusions, early diagnosis and immediate surgical intervention were required to save lives. However, in the 1990s, Pate et al60 pointed out that the observations by Parmley’s group had been made in the “presurgical era,” and that the injury pattern had since changed. Rupture at the aortic isthmus was reported in only 45% of Parmley’s cases, whereas the percentage in recent surgical series is generally greater than 80%.52–54 These findings seemed to indicate a change in cause. Motor vehicle crashes became the most common mechanism for BTAI. Moreover, injuries at the aortic isthmus seemed to be more stable than injuries at other locations of the thoracic aorta.7
Because most patients who reach the hospital alive have contained aortic injuries, the initial goal of therapy is centered around prevention of free rupture. The majority of ruptures occur within the first few hours after arrival. With aggressive blood pressure control, the risk of rupture can be reduced from 12% to 1.5%.30,61 Often, the associated injuries are the most common cause of excessive morbidity and mortality. As a result, immediate operative repair may not be possible. Therefore, delayed repair of BTAI has been advocated since the 1990s and early 2000s.62–65
Medical Management
Patients who may benefit from initial medical management and delayed surgical repair include those with (1) cardiac risk factors, such as segmental wall motion abnormality on echocardiography, ongoing angina, previous coronary artery bypass graft, and need for inotropic support; (2) head injury, such as that seen on an abnormal CT (hemorrhage or edema); (3) pulmonary injury, such as pulmonary contusion on imaging combined with any one of the following: PaO2/FiO2 less than 300 mm Hg, positive end-expiratory pressure requirement of 7.5 cm H2O to maintain adequate oxygenation, or inability to tolerate single-lung ventilation; (4) coagulopathy, including extensive nonsurgical bleeding, international normalized ratio greater than 1.5, or laboratory evidence of consumption, such as increased fibrin-split products or platelets less than 100,000; and (5) severe abdominal solid organ injury and pelvic fractures, when the use of heparin may need to be temporarily avoided.62,66
It is generally recognized that patients admitted with blunt traumatic aortic injuries fall into two distinct categories: stable and unstable. The hemodynamically unstable group has been associated with an all-cause mortality exceeding 90%. In the hemodynamically stable group, there is generally time for a workup and staging of any intervention; mortality in this group is as low as 25%.63 Pate et al64 and Fabian et al65 demonstrated that an aggressive antihypertensive regimen can significantly reduce the rate of rupture in BTAIs. They recommended maintenance of systolic blood pressure to less than 100 mm Hg or mean arterial pressure to less than 80 mm Hg and control of heart rate (<100 beats/min) using an intravenous beta blocker, such as esmolol or labetalol. A vasodilator can be added if a satisfactory blood pressure is not achieved with beta blockade alone.64,65 The combination of a beta blocker and a vasodilator reduces aortic wall shearing force by lowering the ventricular ejection dynamic (dP/dt). Factors that may cause the abandonment of a delayed or nonoperative strategy for BTAI include a rapid increase in the size of a mediastinal hematoma or pleural effusion, anuria persisting for more than six hours, limb ischemia, or the free leak of contrast medium within the thorax. Transient hypotension in association with these factors is an ominous sign and indicates impending rupture.67
The benefits of delayed repair were further demonstrated in a prospective trial of the AAST.68 In a study of 178 patients with BTAI, Demetriades et al68 analyzed outcomes according to the timing of repair (early <24 hours, or delayed >24 hours). Although the two cohorts were similar with regard to baseline characteristics and the mode of repair, the overall mortality in the delayed repair group was significantly lower than the early repair group (5.8% versus 16.5%; P = .034). The mean time from injury to repair was 10.2 hours in the early group and 126.2 hours in the delayed group. Similarly, in a 15-year single institution experience with 338 patients treated at a level 1 trauma center, we found that delayed repair was associated with a significant reduction in mortality compared with immediate open intervention.34 The benefits of delayed repair may be related to better resuscitation and optimization of the patient, as well as treatment of severe associated injuries.
Patients with concomitant traumatic brain injury (TBI) deserve special mention. To maintain adequate brain perfusion, these patients often require higher arterial pressures. As a result, they are often suboptimal candidates for medical therapy and delayed repair. Definitive repair can be considered after stabilization. Nevertheless, the timing of repair should be tailored to the individual patient based on the grade of aortic injury, the presence and severity of associated injuries, as well as the overall physiologic status of the patient. Delayed repair is not an option for patients with grade IV injuries.
In summary, our initial management for patients with BTAI includes resuscitation, blood pressure control, and treatment of associated injuries. Patients with grade I injuries can be managed with medical therapy (anti-impulse control). A repeat CTA study can be performed in six weeks. Stable patients with grade II and III injuries can undergo elective thoracic endovascular aortic repair (TEVAR) if they are anatomically suitable candidates. Patients with grade IV injuries should undergo emergent TEVAR if they are anatomically suitable candidates. Patients who are not anatomically suitable candidates for endovascular repair, such as those with ascending or transverse aortic arch injuries, can undergo open repair.
Thoracic Endovascular Aortic Repair
The first endovascular repair of BTAI was performed by Kato et al in 1997.69 Since then, endovascular repair has been rapidly and widely adopted as the treatment of choice for anatomically suitable candidates with BTAIs. This paradigm shift has taken place despite the fact that there have been no randomized clinical trials to support it.70,71 The most recent clinical practice guidelines published by the Society for Vascular Surgery (SVS) in 2012 suggest that endovascular repair of traumatic TAIs be preferably performed more than open surgical repair or nonoperative management.42 The practice guidelines recommend expectant management with serial imaging for grade I injuries and repair of grade II to IV injuries. For those requiring repair, the timing is urgent (<24 hours) in the absence of other serious concomitant injuries, or repair immediately after other injuries have been treated, but, at the latest, before hospital discharge. The committee suggests (1) endovascular repair, regardless of age, if the patient is anatomically suitable with selective revascularization of the left subclavian artery; (2) routine heparin administration, but at a lower dose than used in elective TEVAR; (3) general anesthesia; and (4) open femoral exposure technique. The committee did not suggest routine spinal drainage.42 It is important to note that at the time of publication of the SVS guidelines, there were no Food and Drug Administration (FDA) approved thoracic endografts labeled for the treatment of BTAI. The majority of the endovascular repairs at the time were being performed using thoracic devices approved for descending thoracic aneurysm repair in an off-label indication. The position of the SVS in suggesting endovascular repair before the availability of an on-label device illustrates the significant early benefits that single- and multi-institution series demonstrated in their initial experience.71,72 Since then, two devices have received FDA approval for treatment of BTAI: the Conformable GORE TAG Thoracic Endoprosthesis (W.L. Gore & Associates Inc., Flagstaff, Ariz), approved in January 2012, and the Valiant Captivia Stent Graft (Medtronic, Santa Rosa, Calif), approved in October 2012.
Endovascular procedures are performed in an operating room supplied with imaging equipment. The abdomen and bilateral groins are prepped and draped. Femoral access is achieved using an open or percutaneous technique. The more suitable femoral and/or iliac access side is selected based on preoperative CTA imaging. Arch aortography is usually performed from the contralateral percutaneous femoral access to identify the location of the injury. The cerebrovascular anatomy is also evaluated based on arch angiography, especially if left subclavian artery coverage is planned. IVUS is used selectively in cases where angiography is equivocal. The patient is then anticoagulated with heparin. A smaller dose than the standard weight-based protocol can be used in patients with severe multiorgan injury, especially those who have intracranial hemorrhage. The thoracic device is selected based on CT images, according to the manufacturer’s sizing recommendations. Measurements are made based on two-dimensional, thin-cut axial CT angiographic scans. The device is delivered over a stiff wire. The endograft is then deployed using standard technique, without any pharmacologic adjunct. The subclavian artery is covered as needed to obtain a proximal landing zone or to gain better apposition with the lesser curvature of the aortic arch. Postdeployment balloon angioplasty is used selectively in cases of incomplete apposition of the graft at the proximal landing zone or proximal type I endoleak. In most cases, a single device is sufficient to adequately exclude the traumatic aortic lesion. Heparin is reversed with protamine (Fig. 157-7).
Figure 157-7 (A) Diagnostic and (B) completion angiography of a patient with a grade III blunt thoracic aortic injury. (C) Follow-up CTA shows successful exclusion of the aneurysm.
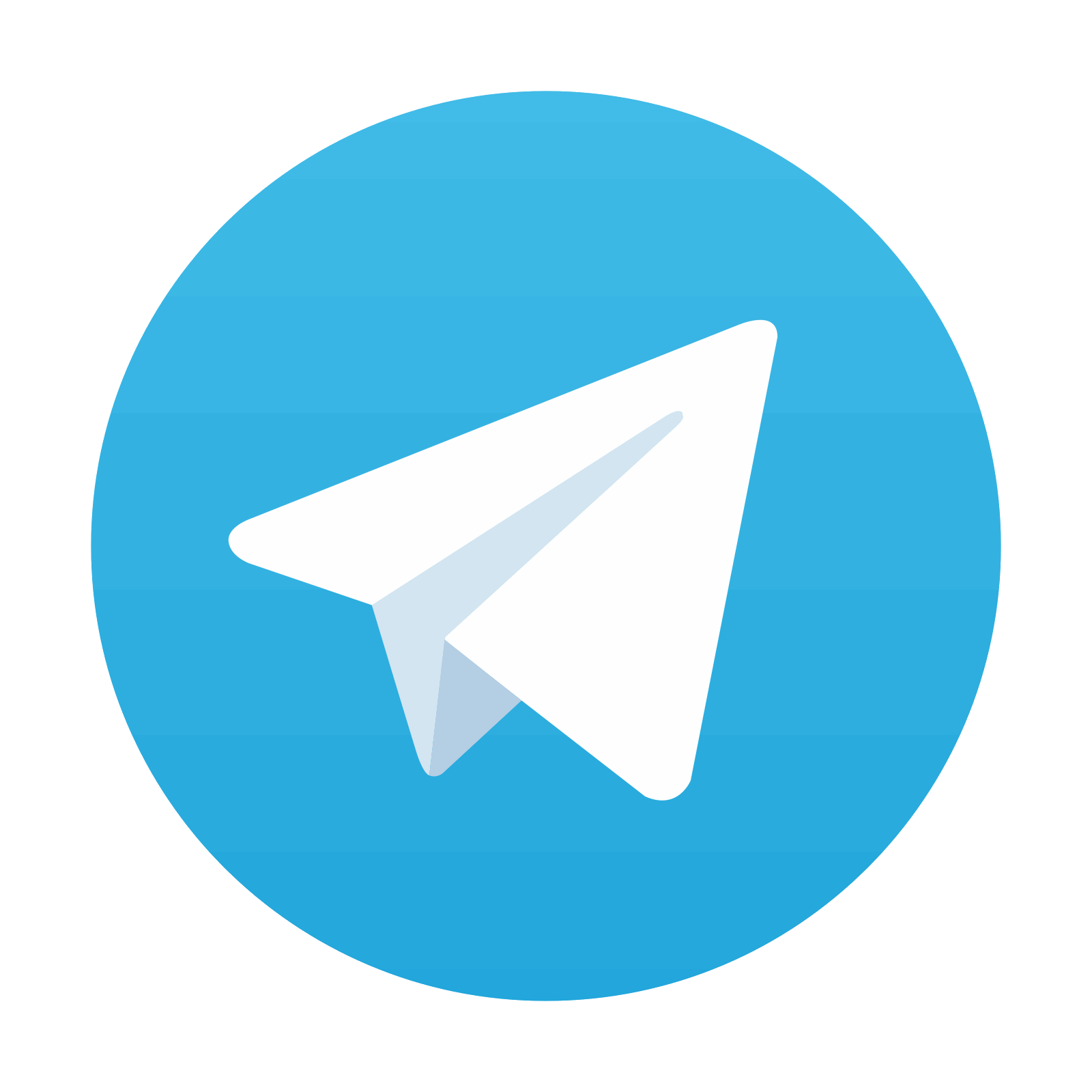
Stay updated, free articles. Join our Telegram channel

Full access? Get Clinical Tree
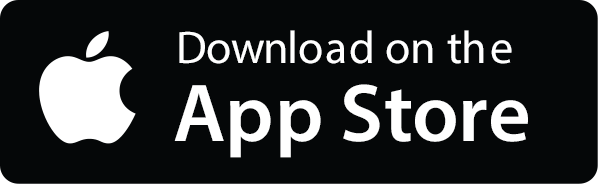
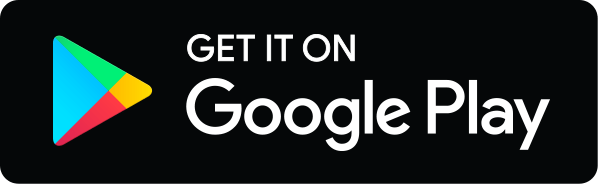