Chapter 4
Vascular Imaging
Introduction
The ability of vascular imaging to characterize atherosclerotic disease within the artery wall permits greater understanding of the atherosclerotic disease process and its influence by clinical and pharmacological factors. Diabetic patients demonstrate the same risk of cardiovascular disease (CVD) associated mortality as nondiabetic patients with a prior history of myocardial infarction [1]. Pathological studies have demonstrated diffuse atherosclerotic disease in patients with diabetes, in contrast to the more localized involvement often seen in nondiabetic individuals [2]. Symptoms of myocardial ischemia are often absent or atypical in diabetic patients, and CVD is frequently detected at an advanced stage, characterized by extensive atherosclerotic obstructive disease [3, 4]. Considering these findings, the role of vascular imaging in diabetic patients on risk stratification, early detection, and evaluation of current severity is potentially of great importance in clinical practice.
Noninvasive imaging techniques can be used as markers of atherosclerosis in individuals with atherogenic risk factors, including asymptomatic diabetic patients [5]. These imaging techniques are most likely to be useful for early detection and risk stratification during the early stage of atherosclerotic disease [6, 7]. Meanwhile, the widespread clinical use of invasive imaging techniques, including coronary angiography, permits the establishment of revascularization strategies [8]. The relative ease of its use in clinical trials also allows for a standardized approach for image acquisition and analysis. However, the requirement for an invasive procedure limits its use to patients who require cardiac catheterization for a clinical indication. Invasive techniques are normally justified in individuals with a strong clinical suspicion of advanced CVD. Furthermore, the current notion that inflammation and immune response may contribute to the development of plaque rupture has garnered increased interest in imaging vulnerable plaques. In both noninvasive and invasive, novel imaging techniques have shown the possibility of visually characterizing the components of plaque. According to patients’ clinical stages and requirements, clinicians need to choose the appropriate imaging modality.
Vascular imaging is also being utilized in the development of new treatment strategies to reduce cardiovascular risks [9]. Given that background therapy will include a greater number of established medial therapies (statins, aspirin, antihypertensive agents), it is likely that the placebo event rate in clinical trials will continue to decline. As a result, future clinical trials are likely to require larger cohorts of patients who are followed for longer periods of time to demonstrate efficacy. In order to evaluate the efficacy of medical therapies in clinical trials, there has been an increased interest in using surrogate markers for clinical events. These surrogate markers will be modified by experimental therapies and reflect stages in the pathological pathways leading to clinical events. Accordingly, vascular imaging has been increasingly employed in risk assessment and the evaluation of novel anti-atherosclerotic therapies.
This chapter summarizes the imaging modalities currently available, their findings in relation to diabetes, and their potential role in clinical settings. There is a summary in Table 4.1.
Table 4.1 Major artery wall-imaging modalities.
Modality | Images | Invasive | Radiation | Advantages | Disadvantages |
Carotid IMT |
| No | No |
|
|
CT coronary angiography |
| No | Yes |
|
|
Magnetic resonance |
| No | No |
|
|
Coronary angiography |
| Yes | Yes |
|
|
Intravascular ultrasound |
| Yes | No |
|
|
Optical coherence tomography |
| Yes | No |
|
|
Near-infrared spectroscopy |
| Yes | No |
|
|
CT, computed tomography. IMT, intima-media thickness.
Vascular Imaging Modalities and Findings in Relation to Diabetes
Noninvasive Imaging Techniques
Carotid Ultrasound
Measuring carotid intima-media thickness (CIMT) and identifying carotid plaque with B-mode ultrasound is a noninvasive, sensitive, and reproducible technique for identifying and quantifying subclinical vascular disease and for evaluating cardiovascular risk (Figure 4.1). Using this approach, it has been well established that increasing CIMT is associated with a greater prevalence of cardiovascular risk factors and the presence of atherosclerotic plaque within various vascular territories. Studies of large population cohorts have demonstrated that increasing CIMT provides additional prognostic information to that of conventional risk factors. The finding that therapies that slow the progression of CIMT also prevent cardiovascular events supports the use of carotid ultrasonic imaging in the evaluation of anti-atherosclerotic therapies [10].
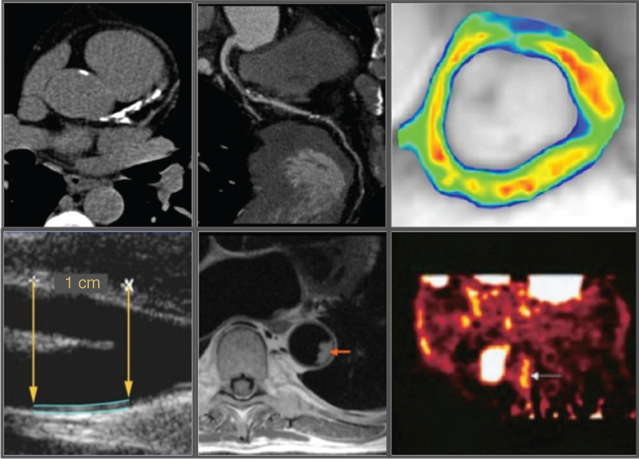
Figure 4.1 Noninvasive arterial wall imaging modalities. Clockwise from upper left: computed tomography calcium scoring, computed tomography coronary angiography, molecular imaging of vascular cell adhesion molecule-1 (VCAM-1) in plaque, fluorodeoxyglucose (FDG) positron emission tomography (PET) imaging of plaque inflammation, magnetic resonance imaging, and carotid intima-medial thickness evaluation on B-mode ultrasound.
Meanwhile, it is also important to recognize that CIMT is not a pre-atherosclerotic stage of the disease process. There is no evidence to suggest that plaque develops in the region where increasing CIMT is detected. Rather, it appears to be a systemic barometer of the presence of atherosclerosis throughout the arterial tree. The main predictors of medial hypertrophy or intimal thickening of common carotid arteries are age and hypertension, which do not necessarily reflect the atherosclerotic process. In contrast, carotid plaque, defined as the presence of focal wall thickening, is suggested as associated with the development of atherosclerotic disease [11]. Carotid plaque predominantly occurs at sites of nonlaminar turbulent flow such as in the bifurcation of carotid artery, but rarely in the common carotid artery except in advanced atherosclerotic disease.
The presence of CIMT greater than or equal to the 75th percentile for the patient’s age, sex, and ethnicity, or carotid plaque, is indicative of increased CVD risk and may signify the need for more aggressive risk-reduction interventions.
Carotid Ultrasound in Diabetes
Numerous cross-sectional studies have continuously reported increased CIMT in patients with diabetes or even a prediabetic state compared with those without [12, 13]. In a report from the Atherosclerotic Risk In Communities (ARIC) cohort, CIMT was 0.07 mm thicker in patients with diabetes compared to those without. Mean common CIMT in middle-aged individuals is reported to range from 0.71–0.98 mm in diabetic patients vs. 0.66–0.85 mm in nondiabetic populations. In diabetic individuals without a history of myocardial infarction, CIMT is similar to that in nondiabetic individuals with a history of myocardial infarction [14]. In a meta-analysis of 21 clinical studies from 1995 to 2004 including 4,019 diabetic and 1,110 impaired glucose tolerance (IGT) patients, both type 2 diabetes (T2DM) and IGT demonstrated thicker CIMT compared with control subjects at 0.13 (95% CI 0.12–0.14) mm and 0.04 (95% CI 0.01–0.07) mm, respectively. Additional risk factors including age, gender, obesity, hypertension, increase of LDL cholesterol, and decrease of HDL cholesterol have been reported to accelerate the increase in CIMT. A longer duration of diabetes and an increase of urinary albumin excretion are also indicated as determinants of an increase in CIMT [15].
The prediabetic state has not been consistently associated with increased CIMT. In the prediabetic population, postprandial glucose levels have been suggested to be more strongly associated with CIMT than levels of fasting glucose and HbA1c [16]. It is likely that postprandial glucose elevation is associated with a clustering of standard risk factors. Consequently, postprandial hypertriglyceridemia, which could be induced by postprandial hyperglycemia, was closely associated with increased CIMT despite normal levels of fasting triglycerides [17].
Accelerated progression of CIMT has also been reported in diabetic patients. In the ARIC study, mean annual CIMT increases were greater by 3–10 µm/y in the patients with diabetes compared with those without [18]. The Insulin Resistance Atherosclerosis Study (IRAS) compared CIMT in CCA and ICA by a range of glucose tolerance at a five-year interval. The rate of CIMT progression was 3.8 µm/y in CCA and 17.7 µm/y in ICA in the normal glucose tolerance group, and both progression rates were approximately two times greater than in the patients with diabetes. Furthermore, the patients who were diagnosed as diabetic at the time of enrollment showed a greater progression of CIMT in ICA than the patients with known diabetes (33.9 µm/y vs. 26.6 µm/y) [19]. This emphasizes the importance of early identification of diabetes and risk-factor control to reduce atherosclerotic change.
In patients with diabetes, CIMT was reported as an independent predictor of cardiovascular events [20]. In a prospective study with 229 T2DM patients free of any cardiovascular complication with at least one additional cardiovascular risk factor, the predictive value of increased CIMT, thicker than the median value of 0.835 mm, was similar to Framingham scores after a five-year follow-up. The addition of CIMT on Framingham scores showed further improvement of the risk-prediction value. CIMT was found to have a predictive value for future coronary events in combination with several other novel risk factors in the ARIC cohort, which included 1,500 diabetic participants [21].
A number of small studies have reported the effects of medical interventions on CIMT in diabetes, particularly with peroxisome proliferation activated receptor (PPAR-γ) agonists. The largest of these studies, the Carotid Intima-Media Thickness in Atherosclerosis Using Pioglitazone (CHICAGO) trial, directly compared the impact of two glucose-lowering strategies, the PPAR-γ agonist poiglitazone and the sulphonylurea glymepiride. Raising HDL-C and lowering both tryglycerides and CRP, in addition to improving glycemic control, were associated with halting of CIMT progression with pioglitazone [22]. Subsequent analysis revealed that raising HDL-C was the strongest independent predictor of the ability of pioglitazone to slow CIMT progression. A meta-analysis of five randomized controlled trials, which included four Japanese and 1 German study, examined the effect of alpha-glucosidase inhibitors on CIMT progression and suggested a beneficial impact. Significant increase of HDL-C was observed in the patients treated with alpha-glucosidase [23].
The Stop Atherosclerosis in Native Diabetics Study (SANDS) examined the effects of intensive lipid and blood pressure modifications on the progression of CIMT compared with the standard treatments in the patients with diabetes; target levels of LDL-C below 70 mg/dL and systolic blood pressure below 115 mmHg. After a three-year follow-up, CIMT showed regression in the intensive treatment group in comparison with progression in the standard treatment group (−0.012 mm vs. 0.038 mm; P<0.001). Adverse events related to blood pressure medications were observed more in the intensive treatment group, but clinical event rates did not differ significantly between groups [24]. Subsequently, several subanalyses of the SANDS data have been reported [25]. In the assessment of the additional effects of ezetimibe on statins within the aggressive treatment group, no difference was observed in the progression of CIMT between the statins plus ezetimibe and the statins alone groups. While a significant increase of arterial mass, calculated as the common carotid artery cross-sectional area, was observed in the whole cohort, it decreased in the association with achieved intensive target levels of LDL-C and systolic blood pressure [26]. In a recent analysis, HbA1 levels were negatively associated with the achievement of target levels, yet no relationship was observed between HbA1c and treatment-associated changes in CIMT [27].
The noninvasive CIMT measurement is a well-established technique for early detection of atherosclerosis or risk management in asymptomatic younger patients with type 1 diabetes (T1DM) [28]. Meanwhile, the evidence for increased CIMT seems to be weaker in T1DM patients compared to other conventional risk factors, including obesity, dyslipidemia, and hypertension [28]. A Japanese study showed a significant increase of CIMT related to T1DM in the older generation, 10–19 years old, and a not significant but greater increase in the younger generation, 4–9 years old. In a recent systematic review of CIMT measurements in children and adolescent patients, 6 out of 14 studies examining CIMT in association with T1DM did not show a significant increase of CIMT compared with the control group [29].
Similarly, in a larger study examining T1DM patients, the Epidemiology of Diabetes Interventions and Complications (EDIC) study, CIMT in T1DM did not show a difference between age- and sex-matched nondiabetic subjects, except in ICA among men [30, 31]. The EDIC study further extended its observations and measured CIMT at 1, 6, and 12 years after enrollment in combination with the long-term follow-up of the Diabetes Control and Complications Trial (DCCT) study, which compared the effects of intensive glycemic control on CIMT progression for 6 years [32]. The initial cohort was enrolled between 1983 and 1989, as 13 to 39 years old, had T1DM for 1 to 15 years, and was in generally good health at baseline. While there was no difference in the CIMT between the matched T1DM and nondiabetic population 1 year after enrollment ended, greater CIMT was observed after 6 years. During the DCCT study, CIMT progression was 0.019 mm less in the intensive glycemic control group with HbA1c target by 7.2% than in the standard glycemic control group with HbA1c target by 9%. This beneficial effect of intensive glycemic control was still evident 6 years after the DCCT study ended, but did not have an effect on the CIMT progression between 6 and 12 years [32]. This finding supports early and continued intensive glycemic control in T1DM to retard subclinical atherosclerotic changes.
Studies also showed a presence of more carotid plaques in patients with diabetes compared to those without [33]. In 738 Japanese subjects with normal fasting glucose and normal glucose tolerance, higher insulin resistance calculated as HOMA-IR showed a positive association with the presence of carotid plaque, with an odds ratio of 1.19 (95% CI 1.00-1.41). Carotid plaque is shown as a predictor of cardiovascular events, especially associated with stroke, which supports the identification of carotid plaques for risk stratification [34]. Meanwhile, the evaluations of carotid plaque are usually cross-sectional in middle-aged or older subjects. To detect early atherosclerotic change and evaluate disease progression, combined use with CIMT is recommended.
The assessment of plaque echogenicity has been suggested to be useful in evaluating plaque vulnerability. Echolucent plaques, indicating proneness to rupture, were detected more in the patients with diabetes [35]. By using integrated backscatter (IBS), a Japanese group suggested an increase of echogenicity in carotid plaque due to treatment with poiglitazone in patients with acute coronary syndrome [36]. However, these studies are performed in a relatively small number of patients. Plaque characterization with carotid ultrasound requires standardized measuring methods and needs further investigation.
Computed Tomography
Multiple population studies have reported that calculation of a coronary artery calcium (CAC) score, measured in computed tomography (CT), is an independent predictor of cardiovascular events, even after controlling for risk factors. Without a requirement for contrast administration, CT imaging of the coronary arteries can detect calcification, defined as a hyperattenuating lesion exceeding a threshold of 130 Hounsfield units (HU) with an area of at least three adjacent pixels [37]. Agatston et al. developed a CAC scoring algorithm, based on calcification volume and density, that is now widely used in clinical practice [38]. Statin therapy in patients with a CAC score above 400 showed a reduction in coronary events.
The application of multislice computed tomography (MSCT) scanners for noninvasive coronary angiography has developed rapidly in recent years. Employment of more than 16-row systems have demonstrated a sensitivity ranging from 88% to 95% and a specificity of between 90% and 96% compared with intravascular ultrasound (IVUS) [39]. Since CT angiography has a high negative predictive value of 99% on average, the technique is currently most suited to exclude coronary artery disease. Plaque can be classified as noncalcified, mixed, or calcified. Compared to IVUS, sensitivity and specificity in coronary artery plaque diagnosis were 93% (84–97%) and 98% (96–99%) for calcified plaque; 88% (81–93%) and 92% (89–95%) for noncalcified plaque. Initial comparisons have shown that calcification may represent the duration of atherosclerosis, whereas noncalcified and mixed lesions are more frequently observed in patients with an acute coronary syndrome. Meanwhile, MSCT is subject to a number of limitations, including exposure to a relatively high dose of radiation, currently in the range of 9–12 mSv, lower accuracy in the presence of severe calcification and movement artifacts, and limited application possibilities in cases with irregular heart rate. Taking the radiation exposure and the high negative predictive value of MSCT angiography into consideration, this technique is recommended for excluding CVD in patients of intermediate risk.
Computed Tomography in Diabetes
Studies with CT continuously show the presence of extensive calcification in diabetic coronary arteries compared with nondiabetic. Furthermore, the association between CAC and future cardiovascular events has been observed particularly in patients with diabetes [40]. Raggi et al. followed 10,377 patients (903 with diabetes) for five years after CAC evaluations by CT. There was a significant interaction of CAC scores with diabetes even after adjusting for other risk factors. Mortality increased with increasing baseline CAC scores for both diabetic and nondiabetic individuals, and diabetic patients had a greater increase in mortality than nondiabetic patients for every increase in CAC scores. However, the study examined CAC scores in 269 diabetic patients and did not find a relationship between CAC scores and coronary events during a six-year follow-up [41]. Meanwhile, a recent study, the Diabetes Heart Study (DHS), showed an increase of mortality with increasing levels of CAC in 1,051 diabetic patients. Overall, a greater CAC score may increase the risk of CVD events in the diabetic population [42].
Serial observation of T2DM without prior coronary disease showed the progression of CAC in 30% of subjects after a 2.5-year follow-up in association with a higher baseline CAC score and suboptimal glycemic control. In the EDIC/DCCT study with a type 1 diabetes cohort, prior intensive glycemic control correlated with a lower CAC score in the subjects without retinopathy or microalbuminuria in association with reduced levels of HbA1c. These studies implicate intensive glycemic control as having beneficial impacts on CAC progression. However, it is not known whether the reduction in the prevalence of CAC can be translated into a reduction in the incidence of coronary artery disease. Other than the effects of glycemic control, the effects of statin use on CAC progression have also been reported. However, their results are not consistent. The different severities of diabetes and atherosclerosis in each cohort may cause this inconsistency.
In an evaluation of the diagnostic accuracy of CT angiography, there were no statistically significant differences observed between the diabetic and nondiabetic individuals with 85% sensitivity and 98% specificity compared with coronary angiography. The evidence for the prognostic value of CT angiography in the diabetic population is currently emerging. A small study examining a cohort of 49 diabetics and 49 matched nondiabetics showed that event-free survival was lower in the diabetic patients in association with the presence of coronary artery disease identified on CT.
In the patients who underwent CT angiography for recurrent chest pain, diabetic patients showed more diseased coronary segments than nondiabetic patients, with more nonobstructive (<50% luminal narrowing) plaques. In addition, diabetic patients demonstrated relatively more noncalcified (28% vs. 19%) and calcified (49% vs. 43%), and less mixed (23% vs. 38%) plaques. These observations were confirmed in a smaller population undergoing an invasive evaluation using virtual histology intravascular ultrasound (VH-IVUS) in addition to CT angiography. Furthermore, in asymptomatic T2DM patients, Scholte et al. showed a high prevalence of coronary artery disease in asymptomatic diabetic patients. In a total of 70 patients, 54% had nonobstructive and 26% obstructive (at least one significant ≥50% stenosis) coronary artery disease, and 55% patients had a calcium score greater than 10. These studies indicate the usefulness of noninvasive CT angiography to screen for coronary artery disease in the diabetic population.
Magnetic Resonance Imaging
Magnetic resonance imaging (MRI) of the artery wall has been developed in parallel to its potential in characterizing cardiac structure and function [43]. Early validation studies in animal models and human subjects demonstrated the ability of MRI not only to quantify the extent of disease, but also to provide a characterization of its individual components. These findings, in addition to the potential to assess pathological pathways within the plaque in a noninvasive fashion, suggest that MRI has considerable potential as a tool to evaluate novel anti-atherosclerotic agents. This is also supported by observations that the composition of plaque within the carotid artery on MRI correlated with a likelihood of cerebrovascular events [44]. A number of reports have demonstrated that statin therapy has a beneficial impact on disease progression within the carotid arteries and aorta. It is important to note that in each of the studies, areas containing relatively bulky disease were selected for evaluation, potentially limiting the findings of these studies to these areas. Imaging resolution currently limits this technique to larger arteries.
MRI in Diabetes
An MRI plaque-imaging study in carotid arteries showed overexpression of high-risk lesions in the patients with diabetes, characterized by a lipid or necrotic core surrounded by fibrous tissue with possible calcification (Types IV–V) or a complex plaque with possible surface defect, hemorrhage, or thrombus (Type VI), compared with the patients without diabetes [45]. This suggests a higher risk of carotid plaque rupture in patients with diabetes.
In a study by Kwong et al., MRI showed myocardial scar and delayed gadolinium enhancement in 28% of DM patients who had no known history of myocardial infarction. In this study, the presence of delayed gadolinium enhancement was a strong independent predictor of future major adverse cardiovascular events and death [46].
Fluorodeoxyglucose (FDG) PET/CT
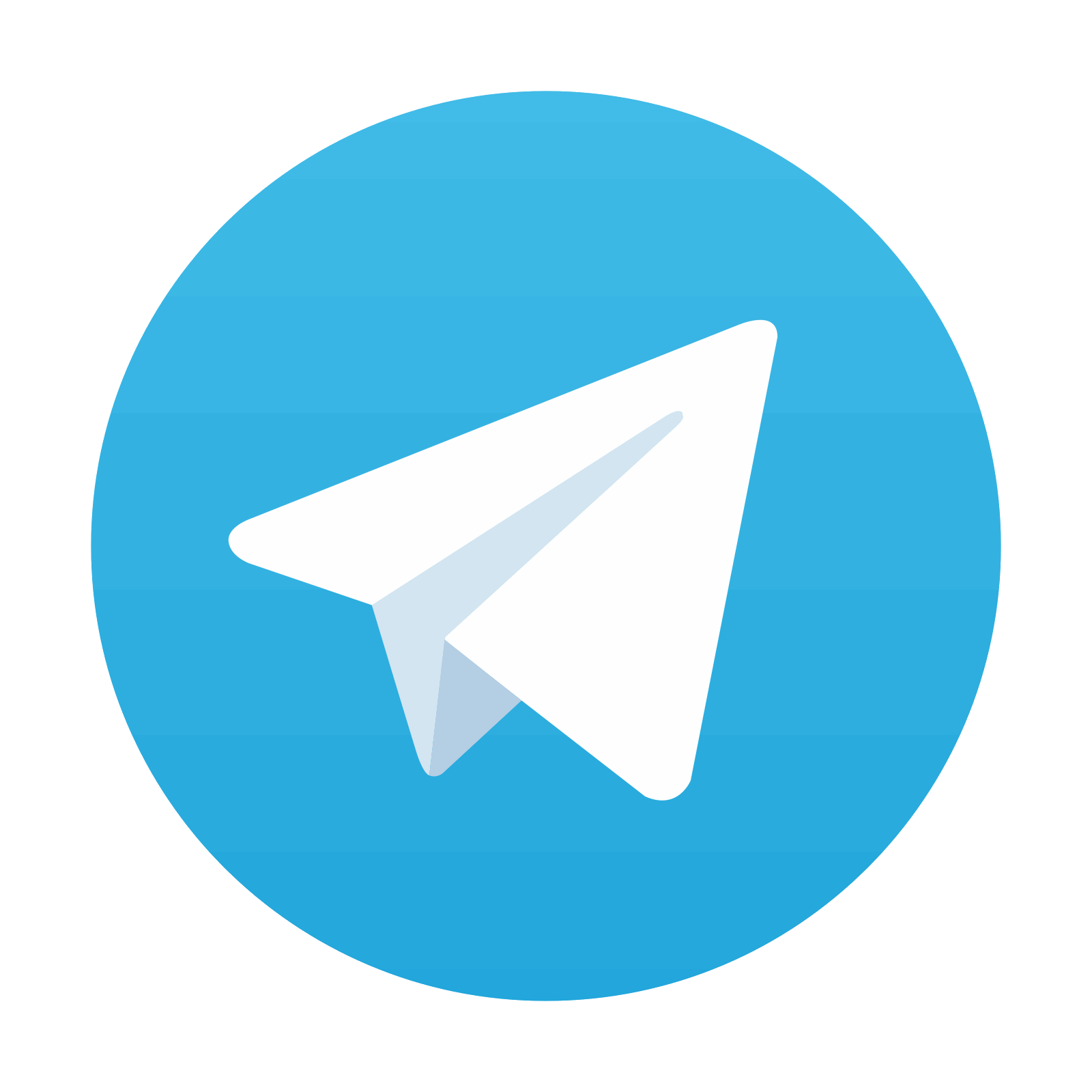
Stay updated, free articles. Join our Telegram channel

Full access? Get Clinical Tree
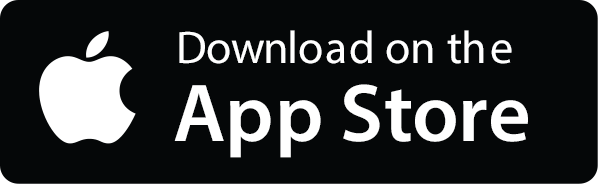
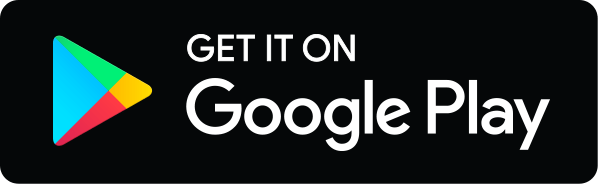