Bioprinting may offer a new solution to the age-old problem of the human body’s lack of “spare parts” when the failure of a small but critical piece threatens the survival of the whole. This report provides a general overview of the current state of bioprinting technology, examining its potential as a future source for tissue grafts and full organ transplants.
Each year, hundreds of thousands of “replacements” are needed: approximately 200,000 coronary artery bypass grafting operations and millions of procedures implanting interposition grafts to bypass obstructed arteries in peripheral vascular disease are performed in the United States alone. When possible, surgeons use autologous grafts, sacrificing saphenous veins, internal thoracic arteries, internal mammary arteries, epigastric arteries, and radial arteries to treat the consequences of vascular disease. The body’s supply of “expendable” vessels is not inexhaustible, a supply issue that is even more acute when taking into account the inequalities between these vessels as replacements (e.g., use of the internal mammary artery in coronary artery bypass grafting procedures is associated with better outcomes and longer term graft patency than use of the saphenous vein ). Current alternatives to autologous grafts include synthetic grafts (although these carry increased risk for stenosis and thrombosis ) and cryopreserved homografts (which are in short supply, have limited long-term durability, and are subject to rejection ). An emerging option is bioengineered vessels, which offer the prospect of structurally appropriate, living vessels constructed from the patient’s own cells. And unlike cloning, which has previously been suggested as a source of “spare” organs and tissue, tissue engineering does not carry the ethical issues associated using embryos to obtain useful stem cells; for example, the ability of bone marrow cells to form vascular tissues similar to those of vascular mixed cells has been demonstrated in the construction of vascular grafts in canine and ovine models. Other potential cell sources for vascular tissue engineering include fat tissue–derived progenitor cells and induced pluripotent stem cells (derived from somatic cells).
Traditional tissue engineering uses a biocompatible gel scaffold to accommodate the cells to be grown in number until they can be harvested for specific application. This is a slow process and is limited with respect to the shape of tissue that can be engineered. Additionally, the vascularization of tissue is challenging, limiting the production of thick tissue or solid organs. A recent innovation with the potential to address these limitations is bioprinting, the biomedical application of rapid prototyping.
Printing as developed by Johannes Gutenberg requires 5 components: written text, a printing press, movable type, paper, and ink. Analogously, “printing” human organs or tissues requires a computer-aided design or blueprint (the “text”); a “bioprinter” or robotic dispenser (the “printing press”); a container for dispensing biomaterials, living cells, or cell aggregates (the “movable type”); processable biomimetic hydrogel or “biopaper” (the “paper”); and self-assembling cell aggregates or cells in hydrogels (the “ink”). The fundamental principle underlying organ printing is tissue fusion driven by surface tension forces, that is, the capacity of closely placed tissue spheroids or cylinders to fuse into a single entity. Although many variants on bioprinting technology are emerging, they all share 3 principal characteristics: (1) they do not use solid scaffolds, (2) they are based on self-assembling tissue spheroids or cell aggregates with maximum cell density, and (3) they use direct processing technology in which cells and hydrogel are dispensed simultaneously without any intermediate steps, such as the creation of a supporting scaffold.
In contrast to scaffold-based tissue engineering, bioprinting is an automated approach with the potential for mass production of tissues from standardized building blocks. It allows a high level of control in the placement and positioning of several cell types in a single tissue through precise 3-dimensional control of the dispenser nozzle, allows the creation of tissues with high cell densities (important for solid organs), and has the potential to solve the vascularization problem in thick tissue constructs by simultaneously printing the vascular tree within the bioprinted tissue or organ. Finally, some tissue engineers believe that bioprinting will eventually evolve into a surgical tool for in vivo tissue building. But meaningful challenges remain to bring bioprinting into the mainstream of medical care: much research requiring multidisciplinary teams and cross-disciplinary research centers is still needed; access to raw materials such as a broad spectrum of progenitor cells, hydrogels, and biomaterials can be challenging; and expensive, sophisticated hardware and software are necessary to support bioprinting. In all, the effective development of bioprinting technology will require long-term sustainable financial support. Moreover, its application in medicine will also require safety, efficacy, and effectiveness research.
However, although we cannot yet print a spare artery or organ in a corner of the operating theater, such a scenario may not be too far off: scaffold-based tissue-engineered skin, blood vessels, and urinary bladders are already clinical realities, with the inability to rapidly and cost-effectively produce them for all patients in need the primary limitation to broader application. In 2009, the first production model 3-dimensional bioprinter was delivered to the developer of the proprietary bioprinting technology, which will in turn supply the units to research institutions investigating human tissue repair and organ replacement. Although initial work will be production of simple tissues such as skin, muscle, and short stretches of blood vessels, the company expects that within 5 years, after clinical trials, blood vessels will be available for use as grafts in bypass surgery. And ultimately, because this technology has the ability to create networks of blood vessels needed to sustain solid organs, printing these could be an alternative to donor organs. An important step in this direction was taken this year with Visconti et al’s report of bioprinting of a branched vascular tree such as would need to be built into a heart, liver, or kidney.
To conclude, “it’s not that wearing out is a good thing, it’s just that wearing out is a pretty good sign that whatever it is doesn’t suffer from one of [the] other reasons that things need to be replaced.” The potential bioprinting holds is for the replacement of the small critical pieces that fail and the opportunity to wear out the larger whole of the human body through a long, full life.
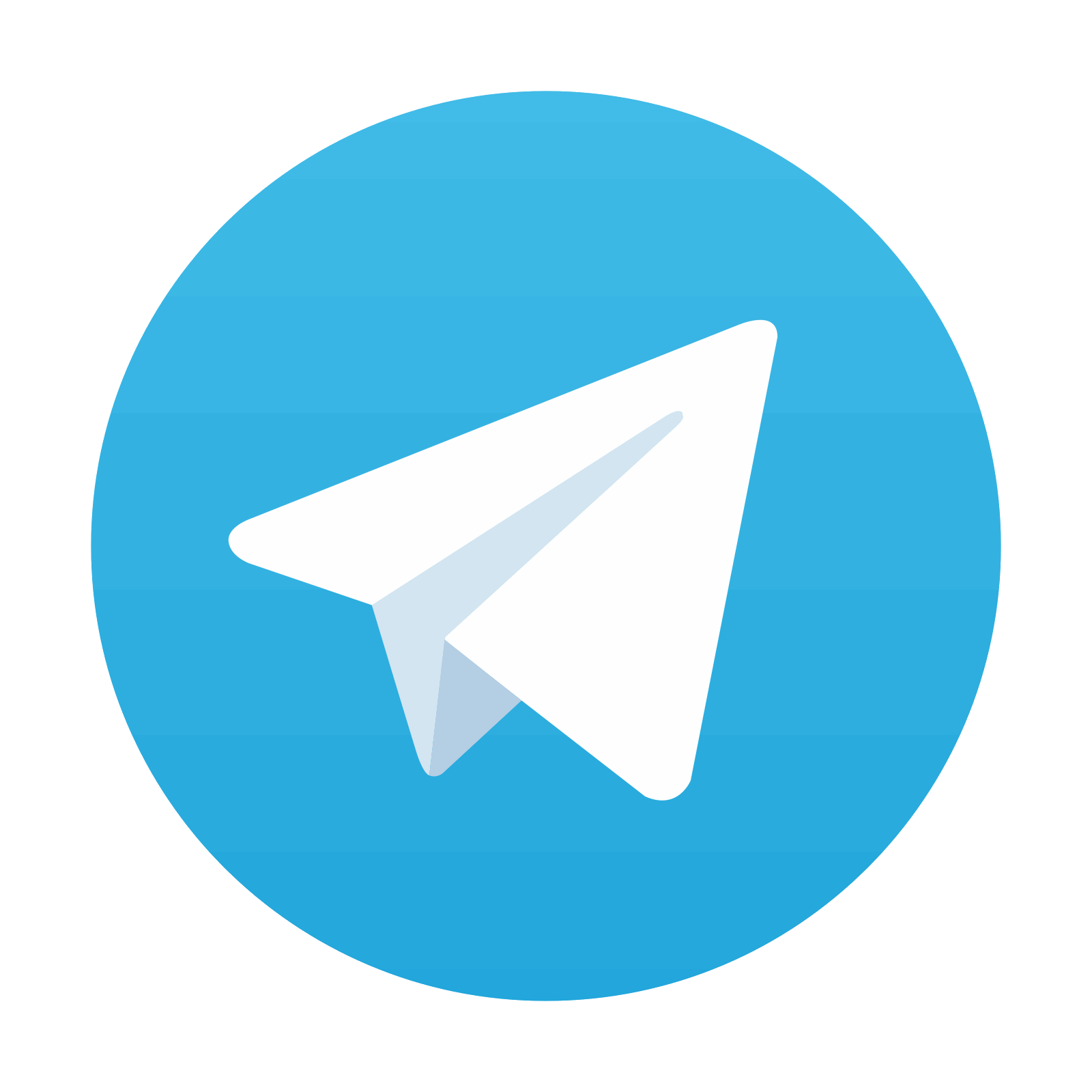
Stay updated, free articles. Join our Telegram channel

Full access? Get Clinical Tree
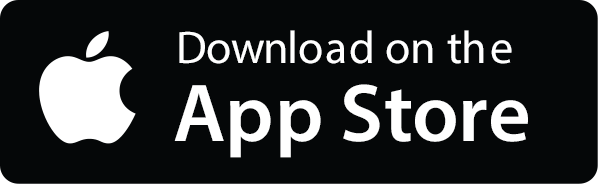
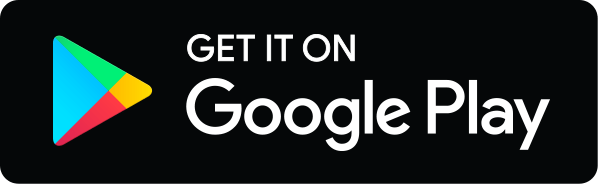