ARTERIOVENOUS MALFORMATIONS
Systemic Arteriovenous Malformations
Systemic arteriovenous malformations in children result from errors in the formation and development of the normal arterial–capillary–venous connections that occur very early in gestation. They can arise anywhere in the body but are most commonly seen in the brain. Most arteriovenous malformations are clinically silent, with symptoms developing in adulthood. There are rare examples of arteriovenous malformations presenting at birth with life-threatening high output heart failure. A large arteriovenous malformation should be included in the differential diagnosis of an infant presenting with severe congestive heart failure.
Vein of Galen malformations are the most common form of symptomatic cerebrovascular malformation in neonates and infants. The clinical manifestations demonstrated early in life reflect both the large size and low resistance of the vascular malformation. There typically is torrential blood flow into the malformation, leading to increased cardiac output, increased cardiac chamber volumes, and congestive heart failure. Blood flow to the body is reduced and may result in ischemic multisystem organ failure, lactic acidosis, and death. Along with signs of severe congestive heart failure, unique physical findings include the presence of a cranial bruit, often heard over the posterior cranium, as well as bounding carotid pulses. The neonate who presents with a vein of Galen malformation may also be cyanotic, related to patency of the ductus arteriosus and elevated pulmonary vascular resistance.
The clinical signs and symptoms of a neonate or infant with a vein of Galen malformation mimic those of a patient with critical congenital heart disease, leading to the request for echocardiography. Although structural anatomy is typically normal, there are distinct anatomic and hemodynamic echocardiographic features that should lead the echocardiographer to suspect the diagnosis.
Echocardiography
Cardiac output can be over twice normal in these patients, resulting in enlargement of all cardiac chambers. Increased blood flow into the vein of Galen malformation may lead to dilatation of the ascending aorta and carotid arteries (Fig. 26.1A). The left subclavian artery and descending aorta are typically of normal caliber but may appear relatively small, suggestive of aortic coarctation. Because coarctation of the aorta has been described with increased frequency in patients with vein of Galen malformations, careful two-dimensional imaging of the aortic arch is critical. Color Doppler interrogation of the descending thoracic aorta reveals marked holodiastolic retrograde flow into the cerebral circulation (Fig. 26.1B, Video 26.1); this is confirmed with pulsed Doppler examination of the descending aorta, which also reveals very limited antegrade systolic flow to the body (Fig. 26.1C). Increased systemic venous return from the malformation leads to dilatation of the superior vena cava (Fig. 26.2A, Video 26.2), with abnormal high-velocity continuous flow identified by pulsed and color Doppler (Fig. 26.2B). Increased frequency of sinus venosus atrial septal defects has also been reported, with some postulating the increased superior caval return in utero may interfere with absorption of the right horn of the sinus venosus into the right atrium.
The newborn with a vein of Galen malformation demonstrates unique hemodynamics secondary to elevation in pulmonary vascular resistance and the presence of a patent ductus arteriosus and patent foramen ovale. The elevated pulmonary resistance in combination with the low systemic resistance from the malformation promotes right-to-left ductal shunting. Decreased flow into the pulmonary arteries and left atrium combined with increased flow in the superior vena cava and right atrium promotes right-to-left atrial level shunting as well. The right heart structures are often markedly enlarged, with right ventricular dysfunction, tricuspid insufficiency, and Doppler evidence of systemic pulmonary artery hypertension.
Although much less common, the infant with a large hepatic arteriovenous malformation may present in a similar fashion. The echocardiographic findings are a bit different, as the site of the malformation in the lower body alters the distribution of blood flow. Increased blood flow into the liver may lead to dilatation of the descending aorta prior to the origin of the celiac axis. Doppler in the descending thoracic aorta will reveal augmented systolic antegrade flow, with persistence of antegrade flow throughout diastole. Increased systemic venous return from the malformation will lead to dilatation of the inferior vena cava. Again, because cardiac output is often twice normal, all of the cardiac chambers may appear dilated.
FIGURE 26.1. Suprasternal notch imaging in a newborn with a large vein of Galen malformation. A: Long-axis image reveals dilatation of the ascending aorta and brachiocephalic vessels, with mild distal transverse arch hypoplasia. There is no posterior shelf seen in the descending thoracic aorta. B: Color Doppler interrogation of the descending thoracic aorta demonstrates marked retrograde diastolic flow (red signal) into the cerebral circulation. C: Pulsed Doppler with the sample volume positioned in the descending aorta confirms dramatic retrograde flow from the aorta into the cerebral circulation, with little antegrade systolic flow to the body.
Treatment
Before the advent of sophisticated imaging techniques and endovascular therapies, the mortality associated with a large systemic arteriovenous malformation in the newborn was extremely high, with some series quoting only 50% survival at 1 month of age. As interventional therapy has progressed with catheter-based occlusion of the malformation, survival has improved. Initial medical management of the congestive heart failure often helps alleviate symptoms, so that direct therapy of the malformation can be delayed until an older age.
Pulmonary Arteriovenous Malformations
Pulmonary arteriovenous malformations are caused by abnormal communications between pulmonary arteries and pulmonary veins. They are commonly congenital in nature, are often multiple, and have a tendency to involve the lower lobes. It is estimated that about 70% of pulmonary arteriovenous malformations occur in patients with hereditary hemorrhagic telangiectasia, also known as Rendu-Osler-Weber disease. Hereditary hemorrhagic telangiectasia is an autosomal dominant disorder characterized by arteriovenous malformations in the skin, mucous membranes and visceral organs. Pulmonary arteriovenous malformations are rarely identified in infancy or childhood, with a peak incidence in the fourth and fifth decades.
FIGURE 26.2. Subcostal sagittal view in a newborn with a large vein of Galen malformation. A: Aneurysmal dilatation of the superior vena cava is seen as it enters the right atrium. B: Pulsed Doppler with the sample volume positioned in the superior vena cava reveals abnormally high velocities with a continuous flow pattern.
Pulmonary arteriovenous malformations create a right-to-left shunt from the pulmonary arteries to the pulmonary veins, leading to systemic arterial desaturation. Because pulmonary vascular resistance is also low, flow into the malformation is not torrential; therefore cardiac output is typically normal or only mildly elevated. Most patients develop symptoms of dyspnea and cyanosis later in life, although life-threatening presentations including severe hemoptysis have been described. The intrapulmonary right-to-left shunt also may facilitate passage of emboli into the cerebral circulation, placing patients at risk for stroke and brain abscess. Presentation early in life is rare, but the classic triad of cyanosis, tachypnea, and an audible bruit over the lung fields in an infant should raise the question of a pulmonary arteriovenous malformation.
Pulmonary arteriovenous malformations can also be acquired. In the pediatric age group, acquired pulmonary arteriovenous malformations have been well described in the patient with single-ventricle physiology palliated with a cavopulmonary anastomosis. The development of pulmonary malformations appears to be related to exclusion of hepatic venous blood from the pulmonary circulation, supported by evidence that these malformations regress after completion of the Fontan operation when hepatic venous blood is again directed into the pulmonary circulation. Acquired pulmonary arteriovenous malformations also occur in the setting of mitral stenosis, chronic liver disease, schistosomiasis, trauma, and metastatic thyroid carcinoma.
Echocardiography
Although the diagnosis of pulmonary arteriovenous malformations can be made with use of many different imaging modalities, contrast echocardiography has become the preferred tool for screening and diagnosis, as it is readily available and noninvasive. The technique involves injection of 5 to 10 mL of agitated saline into a peripheral vein, while simultaneously imaging the heart with two-dimensional echocardiography. The agitated saline contains microbubbles, which are easily visualized by echocardiography because of their echo-reflectivity. In the patient with normal intracardiac anatomy, no intracardiac or intrapulmonary shunt, and normal systemic venous drainage, the microbubbles quickly appear in the right heart, with gradual dissipation as the bubbles are cleared by the pulmonary capillary circulation, never appearing in the left heart (Video 26.3). In the patient with a patent foramen ovale or atrial level shunt, contrast can be visualized in the left atrium within one cardiac cycle of its appearance in the right atrium; the sensitivity of this procedure can be enhanced with the patient performing a Valsalva maneuver, which raises right atrial pressure to facilitate right-to-left atrial shunting. In the patient with a pulmonary arteriovenous malformation, contrast is also visualized in the left atrium, as the microbubbles bypass the pulmonary capillaries through the abnormal arteriovenous channels. However, as opposed to the atrial level shunt, there is a delay of about three to eight cardiac cycles before the contrast appears in the left atrium, because it takes time for the microbubbles to traverse the pulmonary vascular bed.
Contrast echocardiography in the patient with a cavopulmonary anastomosis is also helpful in the diagnosis of pulmonary arteriovenous malformations but is not diagnostic (Video 26.4). In this patient group, the superior vena cava is excluded from the atria and is directly connected to the pulmonary arteries. Therefore, injection of agitated saline into an upper extremity peripheral vein should not produce contrast echoes within the right or left atria, as they should be cleared by the pulmonary capillary bed. If contrast echoes are visualized within the heart, pulmonary arteriovenous malformations need to be considered; however, venovenous collaterals will also bypass the pulmonary vasculature, leading to return of microbubbles to the atria via the abnormal venous channels. It is critical to differentiate pulmonary arteriovenous malformations from venovenous collaterals in these patients, because both can result in marked cyanosis, yet treatment is completely different. Angiography is a better way to evaluate the venous collaterals and, if demonstrated, permits catheter-based interventional closure of the collaterals.
Treatment
Pulmonary arteriovenous malformations can be treated with catheter embolization or surgical resection. Close observation may be reasonable in the asymptomatic patient with only mild desaturation. In the patient with acquired pulmonary arteriovenous malformations, treatment of the underlying disease process will often cause regression of the lesions. Incorporation of the hepatic circulation into the pulmonary vascular bed is the treatment of choice in the patient with pulmonary arteriovenous malformations after cavopulmonary anastomosis.
CORONARY ARTERY ABNORMALITIES
Isolated coronary artery anomalies have been described in approximately 1% to 5% of patients undergoing coronary angiography and approximately 0.3% of patients at autopsy. Many patients are asymptomatic, but signs of myocardial ischemia may present in childhood. Although visualization of coronary artery anatomy has traditionally been obtained using invasive procedures such as coronary angiography, transthoracic echocardiography has become the most important screening procedure for detection of these abnormalities in the pediatric population.
It is worthwhile considering coronary anomalies in children under three classifications: (a) anomalies involving obligatory ischemia, such as anomalous origin of the left coronary artery from the pulmonary artery, where clinical symptoms are frequent and presentation during childhood with evidence of myocardial dysfunction and injury is common; (b) anomalies involving exceptional ischemia, such as anomalous origin of a coronary artery from the opposite sinus of Valsalva, where ischemia can occur under severe clinical stress but there is no evidence of myocardial dysfunction at rest; and (c) anomalies involving absent ischemia, such as coronary artery fistulas, with minimal risk of myocardial dysfunction in childhood.
Echocardiography
Transthoracic echocardiographic imaging of coronary artery origins is critical and should be a part of the routine complete examination in every child. This is usually best accomplished from parasternal short-axis imaging, where both origins can be visualized by scanning superior to the aortic valve with careful interrogation of the aortic sinuses. The left coronary generally rises at approximately 4 o’clock, if you consider the aortic root as a clock face, and the right coronary artery arises at approximately 12 o’clock (Fig. 26.3). Rotation of the transducer clockwise from the standard short-axis position frequently improves imaging of the length of the main coronary artery and its early branches, the left anterior descending coronary artery and the left circumflex coronary artery. In contrast, counterclockwise rotation from the standard short-axis view can facilitate imaging of the origin of the right coronary artery. Color Doppler flow interrogation of the left and right main coronaries is also important, as documentation of direction and timing of flow is also helpful in diagnosing anomalies (as described later). Normal coronary artery flow is predominantly diastolic, because that is the time that aortic pressure (and thus coronary pressure) exceeds ventricular pressure. To see these coronary flow signals, which are usually low-velocity, the Nyquist limit of the color Doppler map must be decreased to 20 to 40 cm/s. Many machines allow presets for coronary imaging to facilitate altering the setup to better identify coronary flow signals. Using the highest frequency transducer that allows adequate penetration is also important in coronary imaging, because fine-detail resolution is required to optimally image these small structures.
Anomalous Origin of a Coronary Artery from the Pulmonary Artery
Anomalous origin of the left coronary artery from the pulmonary artery (ALCAPA) is a rare congenital abnormality, occurring in approximately 1 in 300,000 children. The anomalous left coronary usually arises from the main pulmonary artery, although anomalous origin from the right pulmonary artery has also been described. The timing of presentation during childhood is quite variable and is related to adequacy of collateralization from the right coronary artery. In fetal life, the pressure in the pulmonary artery is high, allowing antegrade perfusion of the left coronary artery. However, shortly after birth, when pulmonary artery pressures fall, the left coronary artery system becomes dependent on the development of right coronary artery collaterals to supply the left ventricular myocardium. When few collaterals to supply left coronary flow are present, there is significant myocardial ischemia in infancy, leading to symptoms such as irritability, tachypnea, diaphoresis, and congestive heart failure. The clinical picture mimics the presentation of a patient with idiopathic dilated cardiomyopathy. In the patient with adequate right coronary collaterals, presentation is often delayed until adolescence or young adulthood, because myocardial function can be well preserved. Sudden cardiac death can occur in this age group, particularly with exercise, and is likely related to limited coronary reserve with development of pathologic ventricular arrhythmias during times of increased myocardial demands.
FIGURE 26.3. Short-axis images of the normal coronary origins from the aortic root (Ao). A: Normal origins of the left main coronary artery (LCA) from the left sinus of Valsalva and right coronary artery (RCA) from the right sinus of Valsalva are well visualized. With clockwise rotation of the probe from this position in B, a longer length of the left main coronary and its bifurcation into the left anterior descending and circumflex branches are visualized. Finally, color Doppler assessment of flow direction in the coronaries can obtained (C) by lowering the color Nyquist limit (in this case to 23 cm/s) to visualize the low-velocity diastolic antegrade flow in the left main coronary seen as a red flow signal coursing appropriately toward the transducer and away from the Ao.
Echocardiography
Prospective identification of ALCAPA using echocardiography has been well described, and this technique should be diagnostic in most patients. Two-dimensional imaging may provide direct visualization of the anomalous coronary insertion into the pulmonary artery from parasternal long- and short-axis imaging, with the coronary usually inserting into the posterior and leftward aspect of the main pulmonary artery (Fig. 26.4, Video 26.5). The anomalous left coronary artery frequently courses close to its normal site of origin near the left sinus of Valsalva, so recognition of associated echo findings is often critical to the diagnosis (Table 26.1). The right coronary artery is dilated because of the obligate collateral circulation needed to perfuse the left ventricular myocardium, but this may not be striking in the infant with limited collateralization who presents early with a severe dilated cardiomyopathy (Video 26.6). Dramatic right coronary dilatation and tortuosity are characteristic of the older asymptomatic child (Fig. 26.5). In addition, the older patient is likely to have prominent coronary collaterals that can be identified using color Doppler flow mapping as abnormal diastolic flow signals within the myocardium of the ventricular septum (Fig. 26.6, Video 26.7A,B).
FIGURE 26.4. Two-dimensional image of anomalous insertion of the left main coronary artery into the posterior aspect of the proximal main PA from a parasternal short-axis view in a four-month-old infant with anomalous left coronary artery from the pulmonary artery (PA). The left anterior descending (LAD) and circumflex (Cx) arteries are both seen entering the PA through a short left main coronary segment, clearly a long distance from the normal entrance into the aortic root (Ao).
FIGURE 26.5. Short-axis image of the origin of the right coronary artery (RCA) in a 10-year-old child with anomalous origin of the left coronary from the pulmonary artery. The right coronary arises appropriately from the anterior aspect of the aorta (Ao) and is markedly dilated (arrows) because of the increased flow into that coronary, which measured 5.6 mm in diameter proximally.
Because the anomalous left coronary artery is perfused retrograde from the right coronary artery collaterals, identification of retrograde filling of the left coronary is critical for diagnosis (Fig. 26.7, Video 26.8). This can be accomplished by documenting direction of flow in the left main coronary artery using color or spectral Doppler, with the expectation that appropriate flow will progress away from the aortic root (usually seen as a red Doppler signal in the normal patient because the left coronary arises from the more posteriorly positioned left sinus and courses anteriorly toward the transducer from parasternal windows). Careful screening for diastolic flow signals in the main pulmonary artery by color Doppler is also important (Video 26.9). Mitral valve abnormalities are variable, but fibrotic changes of the chordae and papillary muscles secondary to chronic ischemia are common and can lead to the development of mitral valve prolapse and mitral insufficiency (Fig. 26.8, Video 26.10). Left ventricular dysfunction should always increase suspicion of ALCAPA, especially when endocardial fibrotic changes are also seen, but left ventricular function can be well preserved, particularly in older children with well-developed collaterals (Video 26.11).
FIGURE 26.6. Short-axis image of color Doppler interrogation of the ventricular septum shows septal coronary collateral flow in the same 10-year-old child as Figure 26.5. The child was initially referred for evaluation of a heart murmur and was found to have anomalous origin of the left coronary from the pulmonary artery. A low-velocity linear diastolic flow signal is seen in the ventricular septum (arrows). The color Doppler and spectral Doppler timing of flow in diastole help differentiate this septal collateral from a ventricular septal defect, which is generally characterized by a high-velocity systolic flow signal. The identification of this septal flow signal was the initial echo finding that led to the diagnosis of ALCAPA in this boy.
FIGURE 26.7. Short-axis image using color Doppler to identify retrograde flow in the left anterior descending coronary artery (LAD) in the same four-month-old infant with anomalous left coronary artery from the pulmonary artery shown in Figure 26.4. Flow in the LAD is blue as it moves away from the transducer towards the pulmonary artery (PA), which is abnormal because it should be flowing away from the aortic root (red Doppler signal) rather than towards it. A turbulent flow signal (arrows) is also seen in the pulmonary artery as the anomalous left coronary artery empties into the low-pressure pulmonary artery.
Surgical Therapy
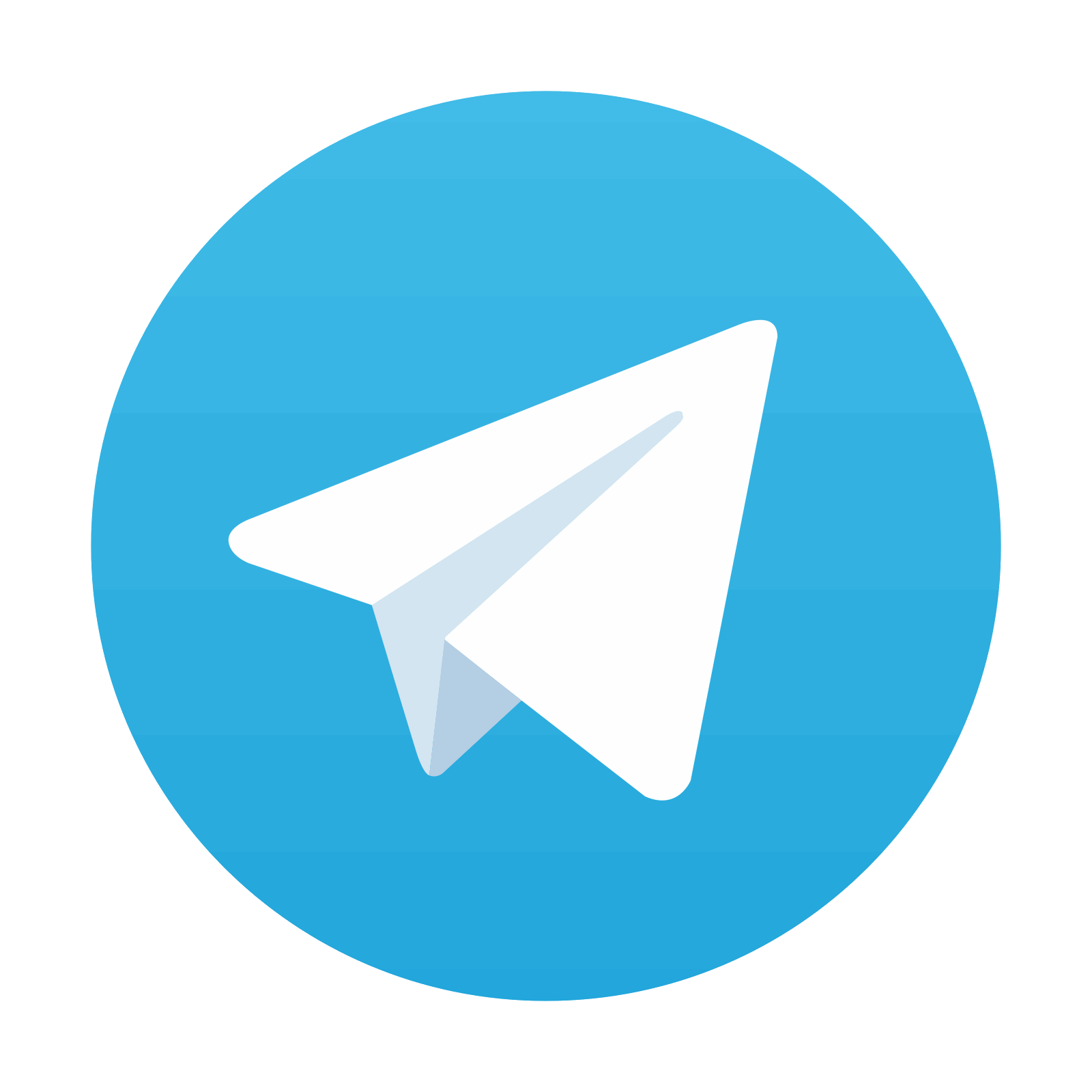
Stay updated, free articles. Join our Telegram channel

Full access? Get Clinical Tree
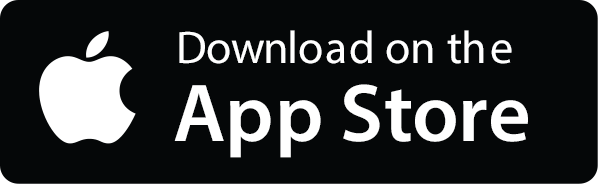
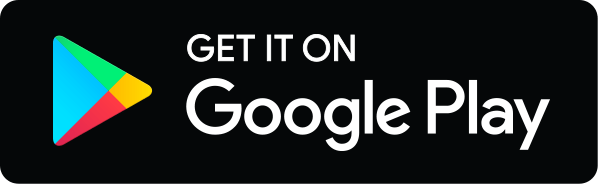