Distinguishing the pathologic hypertrophy of hypertrophic cardiomyopathy (HC) from the physiologic hypertrophy of professional football players (PFP) can be challenging when septal wall thickness falls within a “gray zone” between 12 and 16 mm. It was hypothesized that 2-dimensional and speckle-tracking strain (ε) echocardiography could differentiate the hearts of PFPs from those of patients with HC with similar wall thicknesses. Sixty-six subjects, including 28 professional American football players and 21 patients with HC, with septal wall thicknesses of 12 to 16 mm, along with 17 normal controls, were studied using 2-dimensional echocardiography. Echocardiographic parameters, including modified relative wall thickness (RWT; septal wall thickness + posterior wall thickness/left ventricular end-diastolic diameter) and early diastolic annular tissue velocity (e′), were measured. Two-dimensional ε was analyzed by speckle tracking to measure endocardial and epicardial longitudinal ε and circumferential ε and radial cardiac ε. Septal wall thickness was higher in patients with HC than in PFPs (14.7 ± 1.1 vs 12.9 ± 0.9 mm, respectively, p <0.001), while posterior wall thickness showed no difference. RWT was larger in patients with HC than in PFPs (0.68 ± 0.10 vs 0.48 ± 0.06, p <0.001). Longitudinal endocardial ε and radial cardiac ε were significantly higher in PFPs than in patients with HC, while circumferential endocardial ε was no different. RWT was the parameter that most accurately differentiated PFPs from patients with HC. An RWT cut point of 0.6 differentiated PFPs from patients with HC, with an area under the curve of 0.97. In conclusion, a 2-dimensional echocardiographic measure of RWT (septal wall + posterior wall thickness/left ventricular end-diastolic dimension) accurately differentiated PFPs’ hearts from those of patients with HC when septal wall thickness was in the gray zone of 12 to 16 mm. Two-dimensional strain analysis identifies variations in myocardial deformation between PFPs and patients with HC with gray-zone hypertrophy.
Two-dimensional speckle-tracking echocardiography to derive myocardial strain (ε) is an angle-independent technology that objectively quantifies global and regional myocardial systolic and diastolic function in various cardiac diseases. Pulsed-wave Doppler tissue velocity imaging studies using angle-dependent and less comprehensive techniques have suggested that the quantification of systolic and diastolic function helps in differentiating athlete’s heart from pathologic cardiomyopathy. However, in these studies, the groups have had markedly different average septal wall thicknesses, a criterion in itself that may differentiate these 2 populations. The aim of this study was to selectively examine professional football players (PFPs) in training and patients with hypertrophic cardiomyopathy (HC) to determine the dimensional echocardiographic and functional ε parameters that could differentiate PFPs from patients with HC with “gray zone” wall thicknesses, as well as the diagnostic accuracy of these parameters.
Methods
A total of 66 participants were enrolled in the study: 21 patients with HC, 28 competitive PFPs, and 17 normal control subjects. Inclusion criteria for HC were septal wall thickness of 12 to 16 mm and a clinical diagnosis of HC on the basis of the presence of systolic anterior motion of the mitral valve with a left ventricular (LV) outflow gradient at rest, echocardiographic and/or magnetic resonance imaging findings demonstrating asymmetric septal hypertrophy, and/or a family history of HC, along with the absence of known hypertension or systemic disease. Exclusion criteria for HC were septal hypertrophy with wall thickness >16 mm, an LV end-diastolic dimension <35 mm, or previous septal myectomy. The PFP group was recruited from the United Football League, and these athletes were all active, competitive professional players. Inclusion criteria were increased LV septal wall thickness ≥12 mm without a history of hypertension. Subjects with extreme chamber dilatation (LV end-diastolic dimension >70 mm) or septal hypertrophy (>16 mm) were excluded from analysis. All subjects, including controls, were men aged 18 to 40 years and in sinus rhythm, without known coronary artery disease, diabetes mellitus, conduction disturbances, or decreased ejection fractions (<50%). The original description of gray-zone septal hypertrophy encompassed wall thicknesses of 13 to 15 mm. We used a slightly expanded definition of 12 to 16 mm because some elite athletes can at times demonstrate physiologic hypertrophy >15 mm, while patients with HC will at times demonstrate hypertrophy <13 mm. The study was approved by the Mayo Clinic institutional review board, and subjects provided informed consent.
Standard 4-chamber, 5-chamber (apical long-axis), and 2-chamber apical views and parasternal short-axis views of the base, middle, and apex of the left ventricle were obtained using a commercially available ultrasound system and stored in cine loop format for subsequent off-line analysis. Standard echocardiographic data were recorded, including LV dimensions and ejection fractions, LV mass index, septal and posterior wall thickness, and left atrial volume according to published guidelines, while we used a modified calculation for relative wall thickness (RWT; septal plus posterior wall divided by end-diastolic cavity diameter). Additionally, pulsed-wave Doppler mitral inflow peak E- and A-wave velocities, E-wave deceleration time, and pulsed-wave tissue Doppler of the septal mitral annular velocities (e′ and a′) in the apical 4-chamber view were recorded. Speckle tracking was performed using vendor-customized 2-D Cardiac Performance Analysis software (TomTec Imaging, Munich, Germany). The 2-D Cardiac Performance Analysis software is similar to Velocity Vector Imaging software (Siemens Medical Systems, Erlangen, Germany), for which technical details have been described previously. Briefly, the software detects motion along a user-defined endocardial tracing and an automatically placed subepicardial border by applying successive tracking steps. From this tracking, longitudinal and circumferential ε are determined from the subendocardial and subepicardial trace and radial ε from the full thickness of the myocardium. Parameters defining LV mechanics were averaged from 18 segments in the 3 short-axis views (basal, mid, and apical ventricle) and 18 segments in the 3 apical long-axis views of the left ventricle. Assessment of LV ε was regarded as suboptimal when speckle tracking could not be obtained in ≥4 of the 6 myocardial segments in each apical or short-axis view. A venous blood sample was obtained in standard fashion. Laboratory data included B-type natriuretic peptide levels, lipid panel, and serum creatinine.
Statistical analysis was performed using MedCalc for Windows version 9.5.0.0 (MedCalc Software, Mariakerke, Belgium). All continuous data are reported as mean ± SD and categorical data as percentages. Wilcoxon’s rank-sum tests were used for unpaired comparisons between patient and control groups. Kruskal-Wallis tests were used for comparison of continuous variables among the patient groups. Receiver-operating characteristic (ROC) analysis was performed to determine the accuracy of clinical and echocardiographic parameters in predicting a PFP or a patient with HC. Interobserver and intraobserver variability of endocardial and epicardial layer-based measurements was previously performed using the same software platform in control and myopathic hearts. In that study, variability was calculated as the absolute difference of the corresponding pair of repeated measurements in percentage of their mean in each patient and then averaged for 18 randomly selected patients. Statistical significance for each analysis was defined as p <0.05.
Results
The baseline clinical and laboratory characteristics in the 3 groups are listed in Table 1 . In addition to anthropometric differences (height, weight, body mass index, and body surface area) in the PFP versus HC and control groups, the athletes also demonstrated higher systolic blood pressures. The patients with HC had significantly higher B-type natriuretic peptide levels but with a wide SD.
Variable | PFPs (n = 28) | Patients With HC (n = 21) | Normal Controls (n = 17) | p Value | ||
---|---|---|---|---|---|---|
PFPs vs Patients With HC | PFPs vs Normal Controls | Patients With HC vs Normal Controls | ||||
Age (years) | 27 ± 3 | 30 ± 8 | 28 ± 5 | NS | NS | NS |
Height (m) | 1.9 ± 0.1 | 1.8 ± 0.1 | 1.8 ± 0.1 | 0.003 | 0.019 | NS |
Weight (kg) | 125 ± 23 | 98 ± 25 | 75 ± 17 | 0.0003 | <0.0001 | NS |
Body mass index (kg/m 2 ) | 35 ± 5 | 30 ± 6 | 23 ± 4 | 0.008 | <0.0001 | 0.026 |
Heart rate (beats/min) | 65 ± 8 | 68 ± 10 | 72 ± 7 | NS | NS | NS |
Systolic blood pressure (mm Hg) | 133 ± 12 | 117 ± 13 | 115 ± 14 | <0.0001 | 0.003 | NS |
Diastolic blood pressure (mm Hg) | 78 ± 8 | 72 ± 13 | 75 ± 10 | NS | NS | NS |
Total cholesterol (mg/dl) | 200 ± 48 | 172 ± 31 | 192 ± 26 | 0.036 | NS | NS |
Triglycerides (mg/dl) | 159 ± 91 | 139 ± 55 | 133 ± 24 | NS | NS | NS |
High-density lipoprotein (mg/dl) | 52 ± 14 | 42 ± 9 | 48 ± 7 | 0.017 | NS | NS |
Low-density lipoprotein (mg/dl) | 117 ± 42 | 101 ± 32 | 117 ± 24 | NS | NS | NS |
B-type natriuretic peptide (pg/ml) | 13 ± 8 | 137 ± 210 | 7 ± 2 | 0.007 | NS | 0.045 |
Creatinine (mg/dl) | 1.2 ± 0.1 | 1.5 ± 1.8 | 1.0 ± 0.1 | NS | 0.02 | NS |
Table 2 lists the 2-dimensional and Doppler echocardiographic characteristics of each group. There were larger LV dimensions and mass in the PFP group, while left atrial volume index was no different between the PFP and HC groups. Average septal wall thicknesses in the PFP and HC groups were both in the predefined gray zone but were significantly different, while average posterior wall thickness was similar. Doppler echocardiographic differences were limited to a higher septal e′ in the PFP group than the HC group and in normal patients compared to the HC group and shorter isovolumic relaxation times in the athletes.
Variable | PFPs (n = 28) | Patients With HC (n = 21) | Normal Controls (n = 17) | p Value | ||
---|---|---|---|---|---|---|
PFPs vs Patients With HC | PFPs vs Normal Controls | Patients With HC vs Normal Controls | ||||
Septal wall thickness (mm) | 12.9 ± 0.9 | 14.7 ± 1.1 | 9.2 ± 1 | <0.0001 | <0.0001 | <0.0001 |
Posterior wall thickness (mm) | 12.5 ± 1.1 | 13.2 ± 1.7 | 9.0 ± 1 | 0.1 | <0.0001 | <0.0001 |
LV end-diastolic diameter (mm) | 53 ± 5 | 42 ± 5 | 44 ± 6 | <0.0001 | <0.0001 | 0.2 |
LV end-systolic diameter (mm) | 35 ± 4 | 24 ± 5 | 30 ± 4 | <0.0001 | <0.0001 | <0.0001 |
LV mass index (mm/m 2 ) | 112 ± 15 | 104 ± 23 | 76 ± 29 | 0.2 | 0.05 | 0.03 |
LV RWT | 0.48 ± 0.06 | 0.68 ± 0.10 | 0.42 ± 0.04 | <0.0001 | 0.0002 | <0.0001 |
Left atrial volume index | 38 ± 10 | 39 ± 14 | 21 ± 8 | NS | 0.002 | 0.012 |
LV ejection fraction (%) | 60 ± 7 | 59 ± 10 | 62 ± 4 | NS | NS | NS |
E wave (cm/s) | 81 ± 17 | 90 ± 19 | 81 ± 13 | NS | NS | NS |
A wave (cm/s) | 44 ± 10 | 50 ± 16 | 37 ± 9 | NS | NS | 0.02 |
E/A ratio | 1.9 ± 0.6 | 1.9 ± 0.6 | 2.2 ± 0.6 | NS | NS | NS |
Deceleration time (ms) | 221 ± 43 | 201 ± 29 | 231 ± 33 | NS | NS | 0.02 |
LV isovolumic relaxation time (ms) | 77 ± 16 | 93 ± 24 | 93 ± 16 | 0.006 | 0.009 | NS |
Septal e′ (cm/s) | 10.5 ± 1.7 | 8.8 ± 2.5 | 11.5 ± 1.9 | 0.007 | NS | 0.006 |
Septal a′ (cm/s) | 7.2 ± 1.6 | 7.7 ± 2 | 7.5 ± 1.4 | NS | NS | NS |
Septal e′/a′ ratio | 1.5 ± 0.4 | 1.3 ± 0.9 | 1.6 ± 0.5 | NS | NS | NS |
Table 3 lists the longitudinal endocardial ε, longitudinal epicardial ε, circumferential endocardial ε, circumferential epicardial ε, and radial ε values in the HC, PFP, and normal groups. Strain in the longitudinal endocardial and epicardial layers was attenuated in the HC group compared to the PFP and normal groups. For circumferential ε, only circumferential endocardial ε in PFPs and patients with HC compared to controls were significantly attenuated, while no significant differences were seen between the PFP and HC groups. Radial ε was significantly higher in PFPs than in patients with HC.
Variable | PFPs (n = 28) | Patients With HC (n = 21) | Normal Controls (n = 17) | p Value | ||
---|---|---|---|---|---|---|
PFPs vs Patients With HC | PFPs vs Normal Controls | Patients With HC vs Normal Controls | ||||
Longitudinal endocardial ε (%) | −17 ± 2 | −15 ± 3 | −18 ± 2 | 0.01 | 0.18 | 0.002 |
Longitudinal epicardial ε (%) | −14 ± 2 | −13 ± 3 | −18 ± 2 | 0.09 | <0.0001 | <0.0001 |
Transverse ε (%) | 27 ± 7 | 19 ± 7 | 26 ± 10 | 0.0003 | 0.79 | 0.015 |
Longitudinal endocardial ε/RWT (%) | −36 ± 7 | −22 ± 5 | −44 ± 7 | <0.0001 | 0.001 | <0.0001 |
Circumferential endocardial ε (%) | −23 ± 4 | −26 ± 6 | −16 ± 5 | 0.065 | <0.0001 | <0.0001 |
Circumferential epicardial ε (%) | −8.7 ± 2.1 | −8.9 ± 1.8 | −8.4 ± 3.1 | 0.84 | 0.62 | 0.57 |
Radial ε (%) | 24 ± 8 | 19 ± 6 | 25 ± 10 | 0.022 | 0.76 | 0.05 |
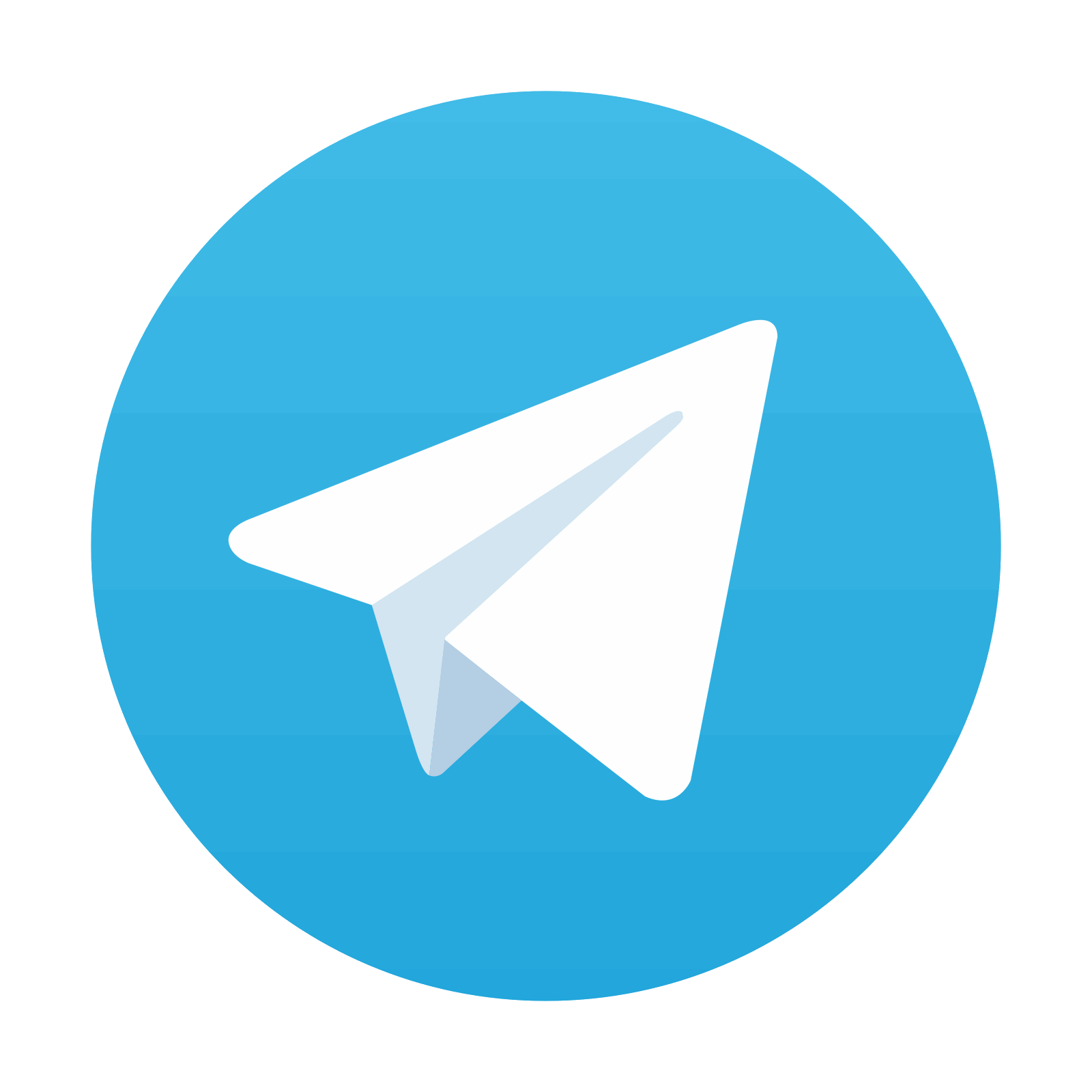
Stay updated, free articles. Join our Telegram channel

Full access? Get Clinical Tree
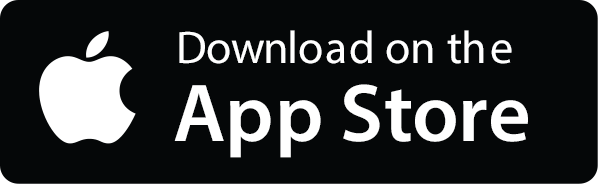
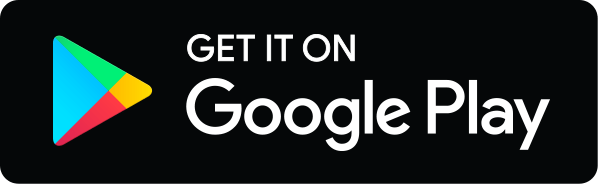
