Fig. 14.1
(a) This is a picture of the Spencer technologies headgear that is utilized for securing the transcranial Doppler probe for peri-procedural imaging. The torque device in the front is for tightening the harness depending on the patient’s cranial circumference. The lateral devices allow for positioning of the transcranial Doppler in a position appropriate for ideal insonation of the middle cerebral system. (b) This is a CT angiogram of a patient who subsequently underwent carotid artery stenting. The “TCD” represents the positioning of the probe, and the square is the insonation window or area being monitored during carotid stenting
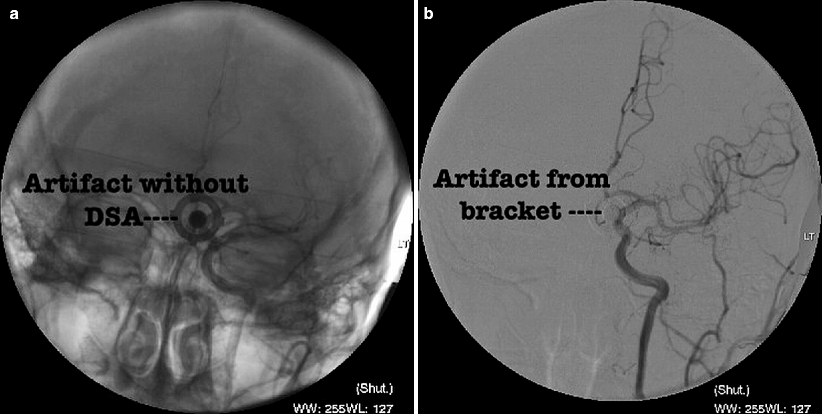
Fig. 14.2
(a) A non-subtracted image illustrating an artifact in the midline related to the ratchet device used to harness the transcranial Doppler. (b) During digital subtraction angiography, the artifact silhouette persists, but one can still define the intracranial cerebral anatomy in the AP view without removing the transcranial Doppler harness
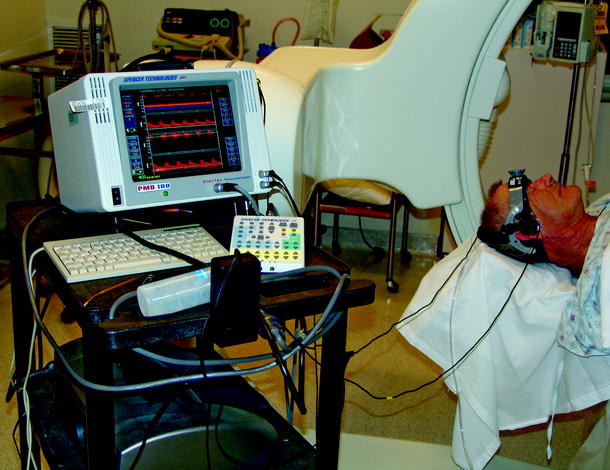
Fig. 14.3
This illustrates the typical setup during transcranial Doppler monitoring in the angiographic suite. The patient is placed in a supine position, and the transcranial Doppler bracket is fixed to the head prior to baseline angiography
Pre-procedural “Baseline” TCD Observations
The baseline TCD pattern may show blunting of the peak systolic wave in patients with severe extracranial disease [29]. More importantly, the TCD may give some insight into the collateral support via the circle of Willis [30–32]. This information will help the operator better understand the patient’s ability to tolerate cerebral protection systems that may arrest or even reverse ipsilateral internal carotid artery flow. Niesen et al. reported the utilization of baseline ratio between the peak systolic velocities in the ipsilateral middle cerebral vessel compared to the contralateral middle cerebral system as a reference for collateral support [33]. Also, the baseline intracranial flow characteristics could be important in predicting patients who are at risk for post-procedural reperfusion syndrome and even intracranial hemorrhage. Mori et al. suggested the possibility of utilizing further hemodynamic testing in patients who are at increased risk for intracranial hemorrhage prior to placing them on the table [34]. This may include utilization of carbon dioxide reactivity or VMR testing with Diamox prior to interaction with the lesion. More recently, a group out of Greece showed that pre-procedure exhausted cerebrovascular reactivity on ipsilateral TCD was an excellent predictor of reperfusion syndrome [35].
Peri-procedural TCD Observations
Transcranial Doppler allows us to continuously monitor two important parameters during carotid artery stenting. The first is related to insuring preserved middle cerebral flow velocity and pulse volume. The utilization of a proximal or distal occlusive device for cerebral protection does result in interruption or reversal of flow in the ipsilateral internal carotid artery [36]. During internal carotid artery flow arrest or reversal, a dramatic drop in middle cerebral flow velocity on TCD may precede the clinical hemispheric symptoms in patients with severe contralateral disease or inadequate collateral support due to incomplete circle of Willis. This provides the operator with additional insight as to how balloon occlusion will be tolerated and whether the procedure will need to be staged. Currently, the most widely tested distal occlusion balloon protection system is the GuardWire (previously known as PercuSurge). This device is a low-pressure balloon and on a. 014″ wire that can be navigated across the lesion and then inflated to occlude the internal carotid artery during transcatheter intervention [20]. Utilization of this type of distal balloon occlusion system has proven to be effective in reducing the risk of embolization. However, Nadim Al-Mubarak et al. have shown with TCD the release of particles during balloon deflation that is likely related to inadequate particle retrieval from the cul-de-sac around the balloon [37].
Parodi has shown with TCD the ability to completely reverse flow in the middle cerebral artery by transcatheter occlusion of the ipsilateral common and external carotid artery while at the same time creating negative pressure at the tip of the guiding sheath, as noted in Fig. 14.4 [38]. This may have significant implications for optimizing cerebral protection during carotid stenting and ultimately could facilitate clot retrieval during transcatheter treatment of acute stroke.
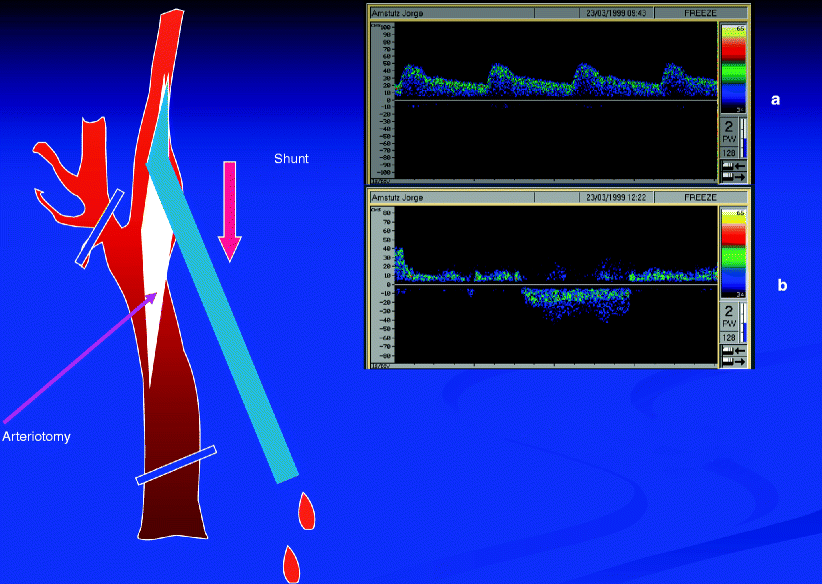
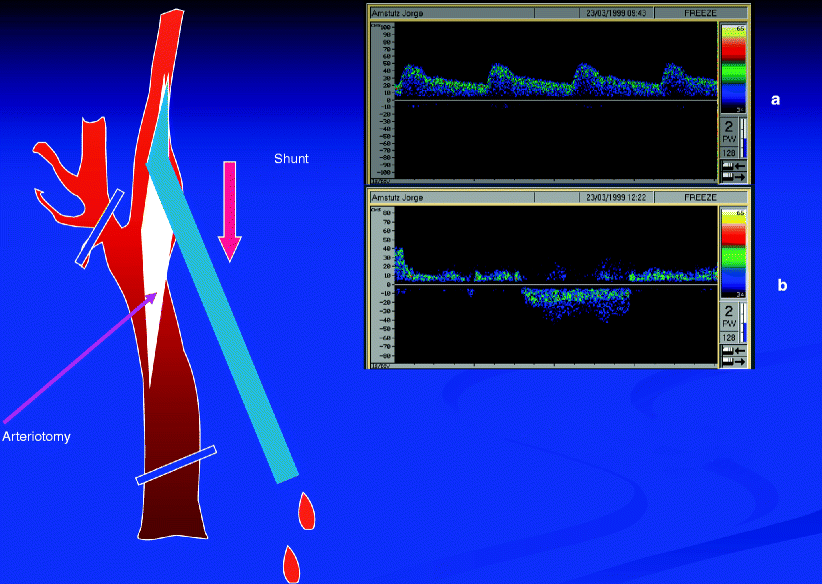
Fig. 14.4
This was provided compliments of Dr. Juan Parodi from his original work evaluating transcranial Doppler evidence of intentional middle cerebral artery flow reversal during carotid endarterectomy. TCD before (a) and after (b) arteriotomy and shunt insertion (Courtesy of Dr. Juan Parodi)
It should be noted that TCD suggests that middle cerebral artery flow is reduced by 10–30% when a distal filter is opened due to filter-induced flow resistance [39]. In patients with an intact circle of Willis undergoing carotid stenting with a protection system that preserves flow (i.e., filter), the sudden interruption of flow to the ipsilateral middle cerebral artery could be an ominous finding suggesting a large embolic event or spasm in the internal carotid artery proper. Also, filter systems do have a threshold of particulate debris based on the filtered design and size [40]. If the volume of embolic debris exceeds the filter threshold, then occlusion can occur, and this may be heralded by changes on TCD. Similar changes can occur if there is spasm in the ipsilateral internal carotid artery system related to the distal protection filter [27].
The second variable that is monitored by TCD during carotid artery stenting is the occurrence of microemboli signals (MESs). The reflective properties of microembolic material as it passes through the middle cerebral artery are translated into sudden signal shifts that are depicted as high-velocity transient spikes on the continuous Doppler recording [41–43]. These high-intensity signals may represent the egress of small particles or microembolic debris into the ipsilateral hemisphere [44]. It is difficult to differentiate artifact from small air bubbles versus true embolic debris, and that is one of the limitations of this technology [45–47]. Ex vitro studies by Coggia et al. and Ohki et al. have provided significant insight into when particles are released during different stages of the procedure [48, 49]. The peak embolic risk during angioplasty in the ex vitro model appears to be during balloon deflation [48]. Similar findings are seen in vivo with continuous TCD during carotid stenting at the time of balloon deflation [50, 51]. In our experience, MESs are seen during all stages of carotid stenting with or without protection even with navigation of a. 014″ wire across the lesion (Fig. 14.5). Paradoxically, in a study of over 500 patients who underwent CAS with TCD, there was actually a higher number of MESs in the patients with filters in comparison to those without protection, and the clinical significance of these findings have not been clearly defined [52].
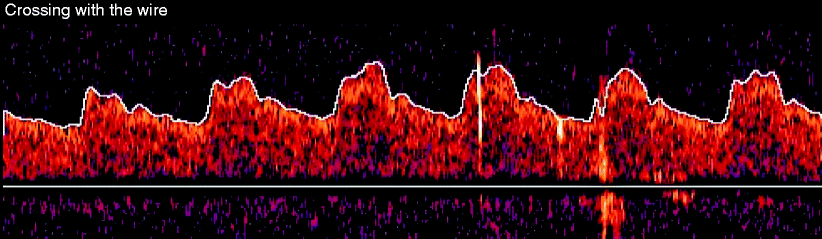
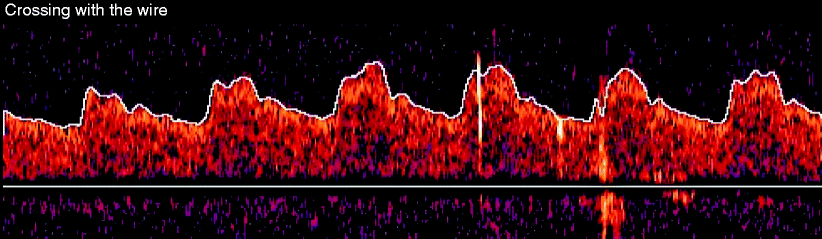
Fig. 14.5
This shows small subtle microembolic signals (MESs) coincident with advancing the wire through the lesion. These MESs are shown as bright signals, the most prominent seen in the fourth complete Doppler pulse sequence
In the context of academic applications, a new approach to TCD has been advocated by Garami et al. whereby dual measurements are recorded simultaneously during CAS to evaluate proximal versus distal protection devices [53]. One transducer is positioned in the traditional transcranial window, while another is used to sample flow in the internal carotid via a submandibular window.
Post-procedural TCD Observations
Some authors have followed TCD with serial interval studies during the first 12 h following carotid stenting [54, 55]. Our center feels that this may be particularly important in patients who are at increased risk for intracranial hemorrhage. The pre-procedural risk factors for reperfusion syndrome and/or intracranial hemorrhage include contralateral severe carotid disease or occlusion, baseline high-grade stenosis or “string sign” with slow-flow, hypertension during the carotid stenting procedure, and baseline decrease of vasoreactivity [56]. Figure 14.6 details the TCD hemodynamic sequence of a patient who developed typical reperfusion syndrome complicated by intracranial hemorrhage during our early experience with unprotected carotid stenting 15 years ago.
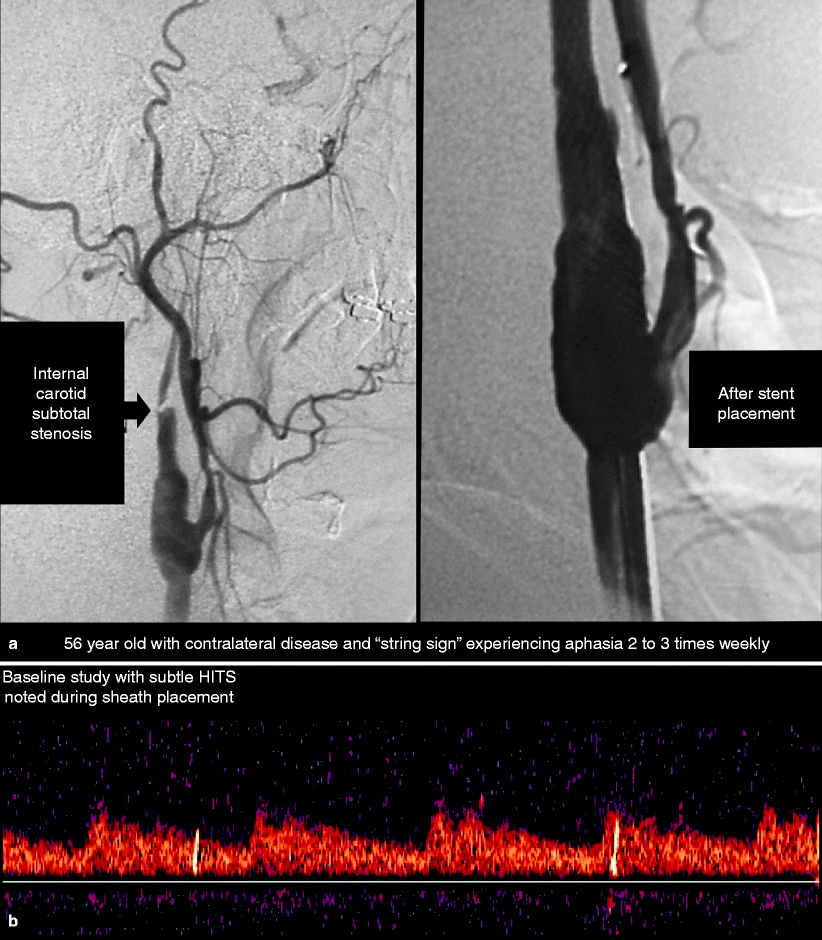
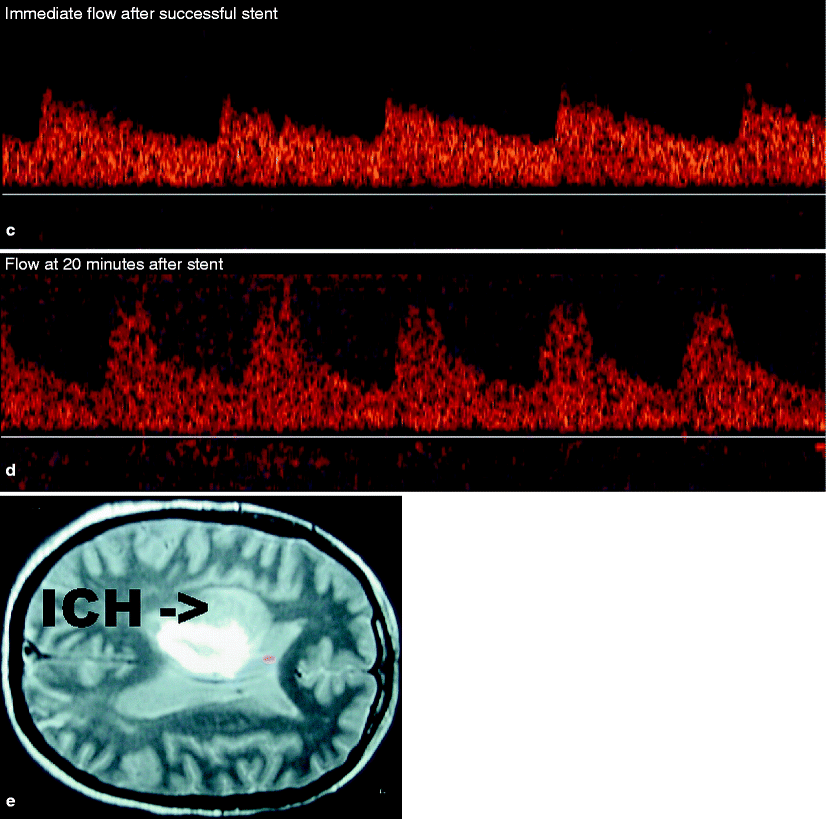
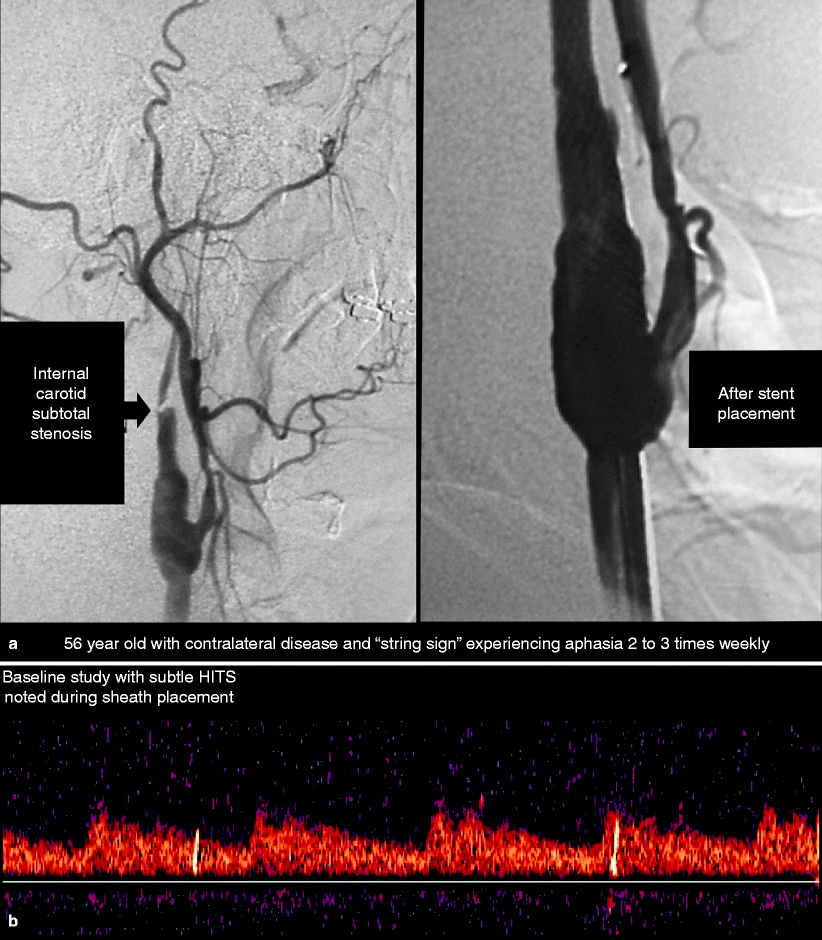
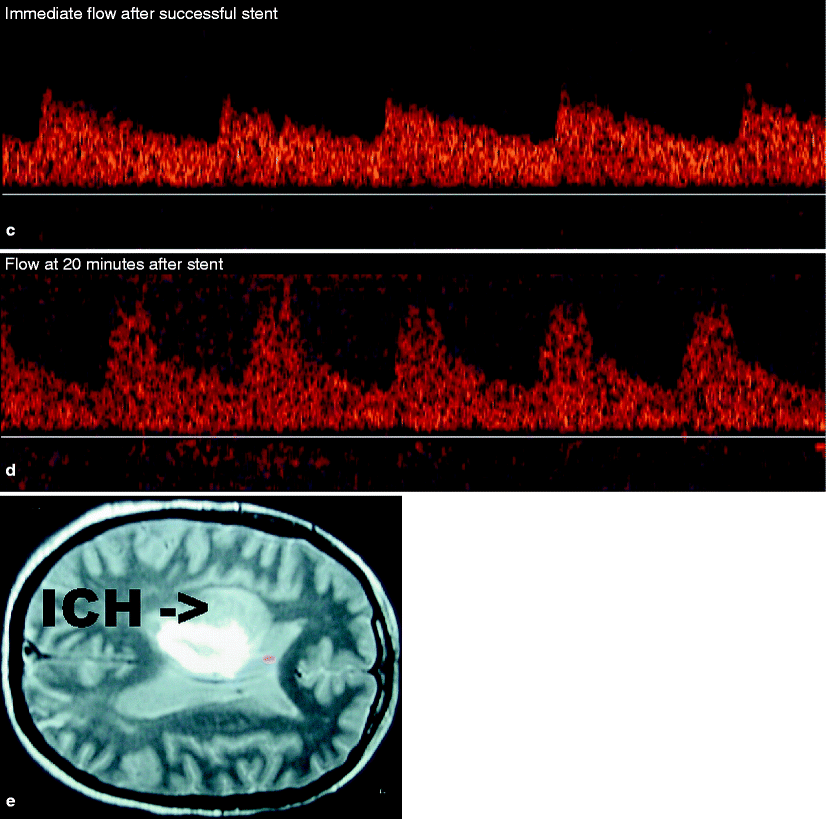
Fig. 14.6
(a) Baseline angiography and follow-up angiography after carotid stenting in a patient with multiple risk factors for postprocedural intracranial hemorrhage. (b) Baseline low pulse volume middle cerebral artery Doppler on the patient illustrated in (a). (c) Immediate flow after carotid stent placement in the patient presented by (a). Note that the flow velocity has increased by approximately 30%. (d) This shows the flow pattern in the same patient depicted in (a). This is 20 min following stent placement, and note that the flow velocity is now three times greater than the baseline velocity. (e) This illustrates the CT scan confirming a large intracranial hemorrhage depicted as ICH 12 h after stent placement
Few studies have actually performed continuous TCD in the early postoperative after carotid stenting. However, it seems that MESs are common during the recovery period after carotid stenting as well [57].
Significance of Microemboli During Carotid Artery Stenting
Microembolic signals detected during carotid endarterectomy have been associated with decrease in cognitive function [58]. Similarly, MESs with so-called embolic storms during different stages of carotid stent procedures are associated with ipsilateral defects on early follow-up diffusion-weighted MRI scan [59–63]. Currently, there is very little data with regard to cognitive function in patients following protected carotid artery stenting, but this is a concern. Interestingly, in a small subgroup analysis of patients during the carotid and vertebral artery transluminal angioplasty study (CAVATAS), there were similar outcomes on neurologic testing in both the carotid endarterectomy and carotid stenting groups in spite of a higher number of MESs in the latter [64].
The threshold or “safe” size for microemboli has not been clearly defined. Early work suggests that any particles more than 50 μm in size will not circulate to the venous cerebral system and thus by definition will cause some arteriolar occlusions [65]. Significant work on this front has been done to better understand the decrease in cognitive function that one sees after coronary artery bypass surgery. It appears that based on a postmortem study by Moody et al., the particles causing decreased cognitive function after a coronary artery bypass surgery are less than 70 μm in size [66]. This is particularly important to understand since most distal protection filters have a pore size of 100–120 μm.
Differentiating Microemboli from Air Bubbles
The differentiation of air bubbles from true atheroemboli has been very difficult. Several techniques have been described, including a defined threshold of greater than 10 hits in a sequence [28]. Different mathematical sequences have also been defined through the years; however, there currently is no ideal way to differentiate these in vivo [46–49].
There are certain stages of the procedure where contamination from air artifact would be less likely. For example, crossing the lesion with the wire as noted in Fig. 14.5 should not be associated with air embolization and is likely true microemboli. Also, many centers have reported the highest numbers of emboli occur with pre-dilatation or post-dilatation as the balloon is deflated, and it is also unlikely there is significant trapped air or artifact during this time, as depicted in Fig. 14.7. On the other hand, one typically sees scattered MESs during contrast injections, which is related to microbubbles in the contrast (Fig. 14.8).
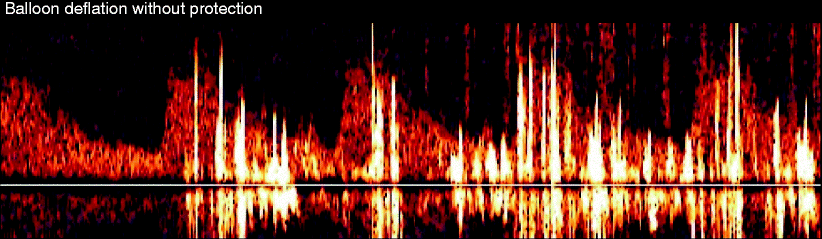
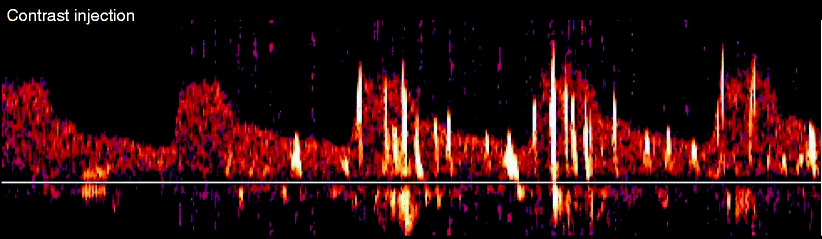
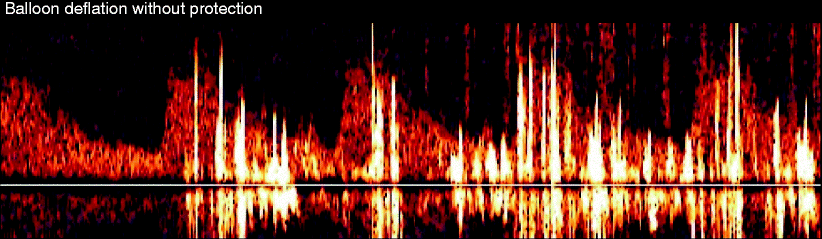
Fig. 14.7
Multiple MESs in a patient after balloon deflation in our early experience before cerebral protection was available
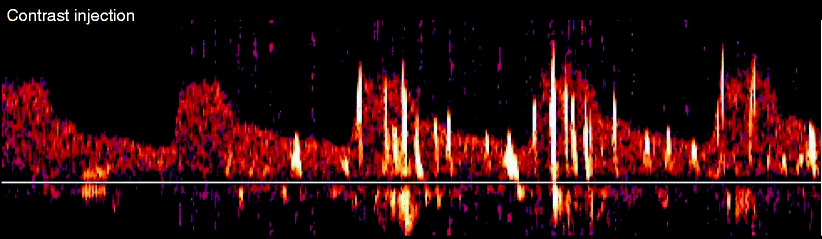
Fig. 14.8
Multiple air bubble MESs during contrast injection
Most of the carotid stents used today are self-expanding stents made of nickel titanium with an outer constraining sheath. There is always a high volume of MES involved with retracting the sheath housing of the self-expanding stent, and some authors have suggested that this is related to the shear force of a stent against the plaque [28]. However, we feel this is related to trapped air within the fabric of the stent and thus less likely of pathologic concern (Fig. 14.9).
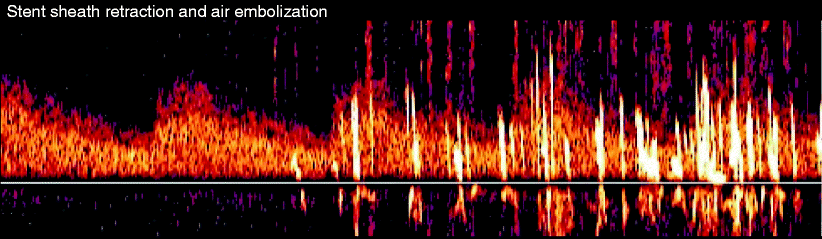
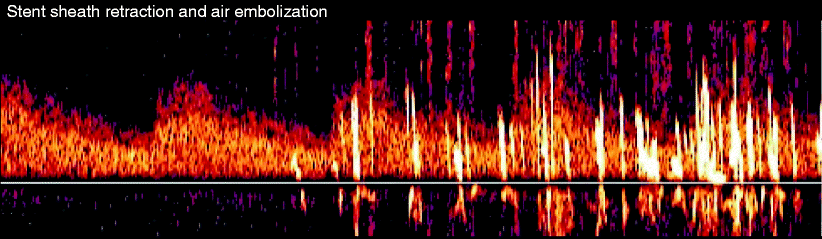
Fig. 14.9
This shows fairly impressive air bubble microemboli during sheath retraction at the time of stent deployment
Conclusions
Currently, TCD has not been declared mandatory for peri-procedural adjuvant monitoring in patients undergoing carotid artery stenting based on consensus documents [67]. Many centers subscribe to the bias that the TCD is more of an academic tool best used to help understand the benefits and failure modes of current protection systems. Peri-procedural TCD may be beneficial in patients who are at high risk for reperfusion syndrome as aggressive hemodynamic monitoring would follow concerning postprocedure middle cerebral flow pattern changes. However, the number of patients experiencing reperfusion syndrome is small, and, thus, level 1 data confirming benefit of TCD will likely never be available.
The burden of embolic load estimated by MESs may give additional insight into the long-term issues of decreased cerebral flow reserve and/or worsening cognitive function after carotid stenting, but linear relationships between embolic load and outcomes have not been confirmed. In our center, TCD has significantly contributed to our understanding of carotid stent procedures, and this feedback has allowed us to improve techniques and participate in the development of newer-generation protection systems. However, the addition of TCD to a carotid stent procedure adds significant time to the case and requires significant consumption of lab technician resources.
In summary, it seems today that TCD should be considered in patients at high risk for reperfusion syndrome or those undergoing carotid stenting with internal carotid artery flow arrest or flow reversal. Only future studies will help us understand the importance of MESs in predicting future issues related to cognitive function. From an academic perspective, ongoing studies evaluating MES frequency with different stent designs and antiplatelet regimens will also advance the art and optimize patient outcomes.