The purpose of this study was to investigate left ventricular (LV) mechanics in hypertensive patients by 2- and 3-dimensional (3D) speckle tracking imaging, and determine the relation between the LV mechanics and functional capacity in this population. This cross-sectional study included 51 recently diagnosed, untreated, hypertensive patients, 49 treated subjects with well-controlled arterial hypertension, 52 treated participants with uncontrolled hypertension, and 50 controls adjusted by gender and age. All the subjects underwent 24-hour blood pressure monitoring, complete 2-dimensional and 3D examination, and cardiopulmonary exercise testing. 3D global longitudinal, circumferential, radial, and area strains were similar between the control group and well-controlled hypertensive patients but significantly decreased in comparison with patients with untreated or inadequately controlled hypertension. Similar findings were obtained for LV torsion and twist rate, whereas LV untwisting rate significantly deteriorated from the controls, across the well-controlled group, to the patients with untreated or uncontrolled hypertension. Peak oxygen uptake was significantly lower in the patients with untreated and uncontrolled hypertension than in the controls and the well-treated hypertensive patients. Peak oxygen uptake was independently associated with LV untwisting rate (β = 0.28, p = 0.03), 3D LV ejection fraction (β = 0.31, p = 0.024), and 3D global longitudinal strain (β = 0.26, p = 0.037) in the whole hypertensive population in our study. In conclusion, LV mechanics and functional capacity are significantly impaired in the patients with uncontrolled and untreated hypertension in comparison with the controls and the well-controlled hypertensive patients. Functional capacity is independently associated with 3D global longitudinal strain, LV untwisting rate, and 3D LV ejection fraction.
A recent study showed that increased exercise capacity in hypertensive population was associated with lower mortality risk regardless of body mass index, whereas other investigations revealed the relation between exercise capacity, left ventricular (LV) diastolic function, and LV hypertrophy in the hypertensive patients. However, the relationship between LV mechanics assessed by 3-dimensional (3D) speckle tracking and functional capacity has not been investigated. The purpose of the present study is to investigate LV mechanics in hypertensive patients (recently diagnosed, well-treated, and uncontrolled hypertension) by 2-dimensional (2D) and 3D speckle tracking imaging and determine the relation between the LV mechanics and functional capacity obtained by cardiopulmonary exercise testing in the hypertensive population.
Methods
Our cross-sectional study included 101 consecutive untreated subjects who were referred to our outpatient clinic due to hypertension screening, and 101 treated hypertensive patients who were referred to our clinic because of treatment evaluation. The study was conducted at Cardiology Department, University Hospital “Dr Dragisa Misovic” in Belgrade, Serbia from October 2011 to March 2013. All the participants underwent 24-hour blood pressure (BP) monitoring, and according to 24-hour systolic BP values, the study population was divided into 3 groups: 50 untreated subjects with normal BP who were included in the control group, 51 recently diagnosed untreated hypertensive patients, 49 treated hypertensive subjects with well-controlled arterial hypertension, and 52 treated participants with uncontrolled hypertension. All the participants were aged <65 years. The patients with white coat hypertension were excluded from the study. The subjects with heart failure, coronary artery disease, previous cerebrovascular events, atrial fibrillation, congenital heart disease, valvular heart disease, body mass index ≥30 kg/m 2 , neoplastic disease, cirrhosis of the liver, kidney failure, sleeping disorders, or endocrinological diseases including type 2 diabetes mellitus were also excluded from the study. The subjects with unsatisfactory 3D acquisitions (13 participants) were also excluded from any further analyses.
Anthropometric measures (height and weight) and laboratory analyses, as well as antihypertensive drugs usage, were taken from all the subjects included in the study. Body mass index was calculated for each patient. The duration of hypertension was defined by the length of antihypertensive drugs usage. The study was approved by the local ethics committee, and informed consent was obtained from all the participants.
Noninvasive 24-hour ambulatory BP monitoring was performed on the nondominant arm using Schiller BR-102 plus system (Schiller AG, Baar, Switzerland). The recording was then analyzed to obtain 24-hour daytime and nighttime average systolic BP, diastolic BP, mean arterial BP, and heart rates. Arterial hypertension was defined by a 24-hour systolic BP of ≥130 mm Hg and/or 24-hour diastolic BP ≥80 mm Hg or usage of antihypertensive medications. All hypertensive patients were first divided into 2 groups: (1) the untreated participants who had never used antihypertensive therapy and (2) the treated hypertensive patients. The latter group was again divided into 2 subgroups: the well-controlled hypertensive patients who had a 24-hour BP of <130/80 mm Hg under antihypertensive therapy and the remaining treated hypertensive patients who were classified as the uncontrolled hypertensive subjects. The normal BP group consisted of the subjects who had never used antihypertensive therapy with a 24-hour BP of <130/80 mm Hg. White coat hypertension was defined as an increased BP in clinical settings but normal 24-hour BP. These patients were excluded from the study.
Echocardiographic examination was performed using a 2.5-MHz transducer with harmonic capability and 3D data set acquisition of the LV obtained using the 3V matrix probe and a Vivid 7 ultrasound machine (GE Healthcare, Horten, Norway).
Reported values of all 2D echocardiographic parameters were obtained as the average value of 3 consecutive cardiac cycles. The LV end-systolic and end-diastolic diameters and the LV posterior wall and ventricular septal thickness were determined according to the current recommendations. Relative wall thickness was calculated as (2 × LV posterior wall thickness)/LV end-diastolic diameter. LV end-systolic and end-diastolic volumes and ejection fraction (EF) were calculated using the biplane disc summation method. LV mass was calculated using the Devereux formula and indexed for height powered to 2.7.
Transmitral Doppler inflow and tissue pulsed Doppler were obtained in the apical 4-chamber view. Pulsed Doppler measurements included the transmitral early diastolic peak flow velocity (E), late diastolic flow velocity (A) and their ratio (E/A). Tissue Doppler imaging was used to obtain LV myocardial velocities in the apical 4-chamber view with a sample volume placed at the septal and lateral segment of the mitral annulus during early and late diastole (e′ and a′). The average of the peak early diastolic relaxation velocity (e′) of the septal and lateral mitral annulus obtained by the tissue Doppler was calculated, and the E/e′ ratio was computed.
2D strain imaging was performed using apical LV views (4- and 2-chamber view) and parasternal short-axis views (at the base, just below the mitral level; at the papillary muscle level; and at the apex). The frame rate ranged from 50 to 70 Hz. A commercially available software (EchoPAC 110.1.2; GE Healthcare, Horten, Norway) was used for the 2D strain analysis.
2D longitudinal strain was calculated by averaging all the values of regional peak longitudinal strain and strain rate obtained in 2-chamber and 4-chamber apical views, before aortic valve closure. 2D circumferential and radial strains were assessed as the average of the LV 6 regional values measured in the parasternal short-axis view, at the basal level of the LV.
LV twist values, which represent the difference between average peak degree of rotation at 6 basal segments and 4 apical segments, as well as untwisting rate, were provided by the software. Furthermore, LV torsion was calculated when LV twist was divided by end-diastolic LV longitudinal length between the LV apex and the mitral plane.
A full-volume acquisition of the LV required for further analyses was obtained by harmonic imaging from an apical approach. Six electrocardiogram-gated consecutive beats were acquired during end-expiratory breath-hold to generate full volume. The frame rate was >30 frames/s.
All data sets were analyzed by a commercially available software, 4D Auto LVQ software (EchoPAC 110.1.2; GE Healthcare), which was used for the off-line analysis. 3D-derived LV mass was also indexed for height powered to 2.7. The software automatically identified the LV cavity endocardial border in 3D and provided the measured LV volumes, cardiac output, stroke volume, EF, and LV sphericity index. After that, an automatic trace of the epicardial border was displayed to detect the region of interest required for LV mass and 3D myocardial deformation parameters (speckle tracking). The 3D deformation parameters, global longitudinal strain, global circumferential strain, global radial strain, and global area strain, were calculated as weighted averages of the regional values from the 17 myocardial segments at end-systole. If ≥3 segments were rejected, global strain values were not calculated, and these patients were excluded.
All the study participants underwent a maximum symptom-limited (fatigue and/or dyspnea) treadmill exercise test according to a modified Bruce ramp protocol (adding to the standard Bruce protocol stage 3 minutes; 1.7 km/h, at 5% grading). Beta blockers and calcium channel antagonists were excluded from therapy 3 days before the testing. Patients were encouraged to continue with the test for as long as their respiratory exchange ratio exceeded 1. The peak oxygen uptake, carbon dioxide production, and minute ventilation were assessed with the breath-by-breath gas analysis (CARDIOVIT CS-200 Ergo-Spiro system; Schiller AG, Baar, Switzerland). Spirometry was done in all the subjects before the cardiopulmonary exercise testing, including forced expiratory volume in the first second and the measurement of forced vital capacity, which was computed as a percentage of predicted values, considering age and gender. Peak oxygen uptake was defined as an average value within the last 20 seconds of exercise and expressed as ml/kg/min and METs (1 MET equals 3.5 ml of oxygen uptake per kilogram of body weight per minute). Ventilatory anaerobic threshold and oxygen uptake at this level, expressed as the percentage of peak oxygen uptake, was determined in all the participants. The ventilation/carbon dioxide slope, which showed the linear increase of ventilation relative to carbon dioxide production, was computed automatically by the Schiller computer system.
Clinic arterial BP values were obtained by conventional sphygmomanometer before cardiopulmonary exercise testing by measuring the average value of the 3 consecutive measurements in the sitting position, taken within an interval of 5 minutes, after the subject had rested for at least 5 minutes in that position. During the exercise test, BP was obtained at the last minute of each exercise stage, at peak exercise, and during the recovery period (first, third, and fifth minutes). All BP assessments were performed on the nondominant arm by conventional sphygmomanometer. Heart rate was noted before the exercise testing, at peak exercise, and during the recovery period (first, second, and third minutes).
Continuous variables are presented as mean ± SD, and the analysis of equal variance with Bonferroni post hoc analysis was used to detect differences between the 4 groups as they showed normal distribution. Differences in proportions were compared using the chi-square test. Pearson’s correlation coefficient was used for determining the correlation between different 2D and 3D echocardiographic parameters and peak oxygen uptake. The variables that showed p value ≤0.10 were included into the stepwise multiple regression analyses. Inter- and intraobserver variabilities were examined using Pearson’s bivariate two-tailed correlations. The p value <0.05 was considered statistically significant.
Results
Demographic and clinical parameters of the study population are presented in Table 1 . The participants were of similar age and gender distribution. The prevalence of different antihypertensive drug groups was similar between the well-controlled and uncontrolled groups.
Variable | Controls (n = 50) | Untreated HTA (n = 51) | Well-Controlled HTA (n = 49) | Uncontrolled HTA (n = 52) | p Value |
---|---|---|---|---|---|
Age (yrs) | 46 ± 9 | 47 ± 10 | 48 ± 10 | 50 ± 9 | 0.179 |
Women | 24 (48) | 23 (45) | 23 (47) | 22 (42) | 0.384 |
HTA duration (yrs) | — | — | 6 ± 3 | 7 ± 3 | 0.097 |
BMI (kg/m 2 ) | 24.9 ± 2.9 ∗, † | 25.6 ± 2.8 | 26.3 ± 3.2 ∗ | 26.7 ± 3.4 † | 0.02 |
24-h systolic BP (mm Hg) | 119 ± 8 | 142 ± 9 | 121 ± 8 | 137 ± 8 | <0.001 ‡ |
24-h diastolic BP (mm Hg) | 71 ± 7 | 86 ± 6 | 73 ± 6 | 83 ± 8 | <0.001 ‡ |
24-h mean BP (mm Hg) | 87 ± 8 | 105 ± 9 | 89 ± 8 | 101 ± 9 | <0.001 ‡ |
Plasma glucose (mmol/L) | 4.8 ± 0.67 | 5.05 ± 0.63 | 4.93 ± 0.58 | 5.11 ± 0.65 | 0.082 |
Serum creatinine (μmol/L) | 64 ± 8 | 65 ± 7 | 64 ± 8 | 67 ± 7 | 0.146 |
Hemoglobin (g/L) | 143 ± 11 | 147 ± 12 | 144 ± 11 | 146 ± 12 | 0.287 |
Angiotensin-converting enzyme inhibitor/angiotensin receptor blocker | — | — | 32 (65) | 43 (82) | 0.068 |
Diuretics | — | — | 26 (53) | 22 (42) | 0.322 |
Calcium channel blocker | — | — | 20 (41) | 18 (35) | 0.544 |
β Blockers | — | — | 28 (58) | 20 (38) | 0.074 |
∗ Controls versus controlled HTA (p <0.05).
† Controls versus uncontrolled HTA (p <0.01).
‡ p Value <0.01 for comparison between any 2 groups except for controls versus controlled HTA.
All participants had similar LV diameters, whereas LV relative wall thickness, left atrial diameter, 2D LV mass index, and E/e′ ratio progressively and significantly increased from the control group, across the well-controlled and the untreated patients, to the uncontrolled hypertensive subjects ( Table 2 ). However, there was no statistically important significance between the untreated and the uncontrolled subjects.
Parameter | Controls (n = 49) | Untreated HTA (n = 51) | Well-Controlled HTA (n = 49) | Uncontrolled HTA (n = 52) | p Value |
---|---|---|---|---|---|
2D echocardiographic parameters | |||||
LV end-diastolic diameter (mm) | 48.3 ± 4.3 | 49.7 ± 4.6 | 48.5 ± 4.1 | 49.4 ± 4.5 | 0.305 |
LV end-systolic diameter (mm) | 31.3 ± 3.4 | 31.8 ± 3.6 | 32.1 ± 3.7 | 31.6 ± 3.8 | 0.734 |
Relative wall thickness | 0.36 ± 0.04 | 0.42 ± 0.06 | 0.39 ± 0.05 | 0.43 ± 0.06 | <0.001 ∗ |
Left atrial diameter (mm) | 36.1 ± 3.5 | 40.8 ± 4.4 | 38.5 ± 3.9 | 41.9 ± 4.8 | <0.001 ∗ |
LV mass index (g/m 2.7 ) | 38.5 ± 5.6 | 48.6 ± 6.3 | 39.2 ± 5.8 | 48.4 ± 6 | <0.001 † |
LVEF (%) | 65 ± 5 | 63 ± 4 | 64 ± 5 | 63 ± 5 | 0.11 |
E/A ratio | 1.29 ± 0.34 | 0.96 ± 0.31 | 1.1 ± 0.25 | 0.85 ± 0.22 | <0.001 ‡ |
E/e′ ratio | 5.56 ± 2.3 | 10.6 ± 3.8 | 7.5 ± 2.4 | 11.4 ± 3.9 | <0.001 ∗ |
Peak systolic 2D strain (%) | |||||
Longitudinal | −21.1 ± 2.4 | −18.9 ± 2.1 | −19.9 ± 2 | −18.6 ± 1.7 | <0.001 ‡ |
Circumferential | −23.4 ± 3.1 §, ‖ | −21.6 ± 3 ‖ | −22 ± 3.1 | −21.1 ± 2.9 § | 0.001 |
Radial | 51 ± 14.8 | 46.3 ± 13.8 | 48.8 ± 14.3 | 46.8 ± 14 | 0.332 |
Peak LV twist (°) | 10.5 ± 2.6 §, ¶ | 13.4 ± 2.9 ¶ | 11.9 ± 2.8 # | 13.5 ± 3 §, # | <0.001 |
Torsion (°/cm) | 1.5 ± 0.4 §, ¶ | 1.9 ± 0.5 ¶ | 1.7 ± 0.4 | 1.9 ± 0.6 § | 0.009 |
Untwisting rate (°/s) | −136 ± 39 §, ‖ | −119 ± 33 ‖ | −129 ± 37 # | −108 ± 38 §, # | 0.001 |
3D echocardiographic parameters | |||||
LV end-diastolic volume (ml) | 118 ± 21 | 126 ± 23 | 122 ± 20 | 127 ± 24 | 0.157 |
LV end-systolic volume (ml) | 48 ± 15 § | 55 ± 16 | 51 ± 15 | 59 ± 16 § | 0.003 |
Stroke volume (ml) | 70 ± 16 | 71 ± 15 | 71 ± 18 | 68 ± 17 | 0.771 |
Cardiac output (L/min) | 4.8 ± 0.9 | 5 ± 1 | 4.7 ± 1.1 | 4.9 ± 1.2 | 0.525 |
LVEF (%) | 60 ± 5 | 56 ± 4 | 59 ± 5 | 54 ± 5 | <0.001 † |
LV mass index (g/m 2.7 ) | 36.3 ± 3.5 | 45.4 ± 4.6 | 37.1 ± 3.9 | 45 ± 4.2 | <0.001 † |
Sphericity index | 0.41 ± 0.07 | 0.42 ± 0.08 | 0.42 ± 0.07 | 0.42 ± 0.07 | 0.87 |
3D strain (%) | |||||
Global longitudinal strain | −20.8 ± 3.5 | −17.5 ± 3.1 | −19.2 ± 3.2 | −17.2 ± 2.9 | <0.001 ∗∗ |
Global circumferential strain | −18.9 ± 3.2 §, ¶ | −16.3 ± 2.7 ¶ | −17.8 ± 2.9 # | −16 ± 2.6 §, # | <0.001 |
Global radial strain | 50.1 ± 9.8 §, ¶ | 44.2 ± 8.3 ¶ | 48.4 ± 8.8 # | 43.1 ± 7.8 §, # | <0.001 |
Global area strain | −32.1 ± 5 | −26 ± 4.4 | −30.6 ± 4.8 | −25.4 ± 4.5 | <0.001 † |
∗ p <0.05 for all comparisons except for untreated versus uncontrolled HTA.
† p <0.01 for all comparisons except for controls versus controlled HTA and untreated versus uncontrolled HTA.
‡ p <0.05 for all comparisons except for untreated versus controlled HTA and untreated versus uncontrolled HTA.
§ Controls versus uncontrolled HTA (p <0.01).
‖ Controls versus untreated HTA (p <0.05).
¶ Controls versus untreated HTA (p <0.01).
# Controlled versus uncontrolled (p <0.05).
∗∗ p <0.05 for all comparisons except for controls versus controlled HTA and untreated versus uncontrolled HTA.
The 2D strain analysis showed that longitudinal LV strain was not only significantly better in the controls than in the untreated, the uncontrolled group, or even the well-treated participants but also significantly higher in the well-controlled group than in the untreated or the uncontrolled hypertensive patients ( Table 2 ). Circumferential strain was decreased in the untreated and uncontrolled hypertensive patients in comparison with the controls, whereas radial strain was similar across all 4 groups. The LV twist was not only increased in the untreated and uncontrolled groups compared with the controls but it was also higher in the uncontrolled group than in the well-controlled hypertensive patients. The untwisting rate significantly decreased from the controls, across the well-controlled group, to the uncontrolled and untreated hypertensive subjects who had the lowest untwisting rate ( Table 2 ).
3D echocardiography showed that the subjects with normal BP and the well-controlled hypertensive patients had significantly better LVEF than the untreated and the uncontrolled hypertensive participants. 3D LV mass index was similar between the controls and the well-controlled group, but significantly lower than in the other 2 groups ( Table 2 ). 3D LVEF was significantly lower, whereas LV mass index was significantly increased, in the uncontrolled hypertensive patients compared with the well-controlled subjects.
3D speckle tracking analysis revealed that global longitudinal, circumferential, radial, and area strains were similar in the controls and the well-controlled hypertensive patients, but significantly better than in the untreated and the uncontrolled groups who also had similar strain values ( Table 2 ).
Peak oxygen uptake and achieved METs, obtained by cardiopulmonary exercise testing, were similar between the normal-BP group and the well-controlled hypertensive patients, but still higher than in both groups of patients with high BP ( Table 3 ). The ventilation/carbon dioxide ratio was lower in the uncontrolled hypertensive patients than in the controls, whereas corresponding slope was similar among all 4 groups ( Table 3 ).
Variable | Controls (n = 50) | Untreated HTA (n = 51) | Well-Controlled HTA (n = 49) | Uncontrolled HTA (n = 52) | p Value |
---|---|---|---|---|---|
Forced expiratory volume in first second (%) | 101 ± 14 | 96 ± 11 | 102 ± 13 | 98 ± 12 | 0.067 |
Forced vital capacity (%) | 98 ± 12 | 94 ± 11 | 95 ± 10 | 93 ± 9 | 0.099 |
Rest | |||||
Heart rate at rest (beats/min) | 70 ± 8 | 71 ± 7 | 68 ± 7 | 72 ± 8 | 0.055 |
Systolic BP (mm Hg) | 128 ± 11 | 151 ± 12 | 130 ± 9 | 145 ± 11 | <0.001 ∗ |
Diastolic BP (mm Hg) | 78 ± 7 | 91 ± 8 | 81 ± 7 | 89 ± 7 | <0.001 ∗ |
Maximum exercise | |||||
Heart rate (beats/min) | 168 ± 15 | 165 ± 14 | 163 ± 17 | 162 ± 18 | 0.254 |
Systolic BP (mm Hg) | 180 ± 21 †, ‡ | 194 ± 24 ‡ | 187 ± 18 | 196 ± 17 † | <0.001 |
Diastolic BP (mm Hg) | 100 ± 9 § | 103 ± 8 | 102 ± 10 | 105 ± 9 § | 0.046 |
Maximal load (km/h) | 5.6 ± 1 § | 5.1 ± 1.3 | 5.3 ± 0.9 | 5 ± 1.2 § | 0.038 |
Maximal load (%) | 14.3 ± 2.3 | 13.4 ± 2.7 | 13.7 ± 2.5 | 13.2 ± 2.8 | 0.159 |
Recovery | |||||
Heart rate recovery in 1 minute (beats/min) | 141 ± 15 | 139 ± 16 | 137 ± 17 | 140 ± 19 | 0.676 |
Heart rate recovery in 2 minutes (beats/min) | 117 ± 12 | 119 ± 15 | 114 ± 14 | 117 ± 16 | 0.381 |
Heart rate recovery in 3 minutes (beats/min) | 104 ± 10 | 105 ± 11 | 105 ± 10 | 106 ± 12 | 0.832 |
Peak METs | 8.7 ± 2.2 †, ‖ | 7.5 ± 1.9 ‖ | 8.4 ± 2 ¶ | 7.1 ± 1.6 †, ¶ | 0.001 |
Respiratory exchange ratio | 1.11 ± 0.08 | 1.08 ± 0.06 | 1.09 ± 0.08 | 1.08 ± 0.07 | 0.13 |
Exercise time (minutes) | 12 ± 3 | 11.5 ± 3.5 | 11 ± 2.5 | 10.5 ± 2.5 | 0.06 |
Oxygen uptake at ventilatory threshold (ml/kg/min) | 20.8 ± 4.1 † | 18.7 ± 3.9 | 20.5 ± 4 ¶ | 18 ± 3.7 †, ¶ | 0.001 |
Peak oxygen uptake (ml/min) | 2,353 ± 593 | 2,208 ± 427 | 2,305 ± 516 | 2,154 ± 489 | 0.19 |
Peak oxygen uptake (ml/kg/min) | 30.5 ± 4.6 | 26.2 ± 4.2 | 29.5 ± 4.8 | 24.9 ± 4 | <0.001 # |
Oxygen pulse (ml/beat) | 14.3 ± 3.6 | 13.5 ± 3.2 | 14 ± 3.7 | 13.2 ± 3.1 | 0.36 |
Anaerobic threshold (% of peak oxygen uptake) | 68 ± 8 | 71 ± 9 | 70 ± 9 | 72 ± 8 | 0.109 |
Ventilation/carbon dioxide ratio | 29 ± 7.6 § | 28 ± 6.5 | 27 ± 7 | 25 ± 6 § | 0.023 |
Ventilation/carbon dioxide slope | 23.7 ± 3.2 | 23.5 ± 3.1 | 23.6 ± 3 | 23.4 ± 2.9 | 0.965 |
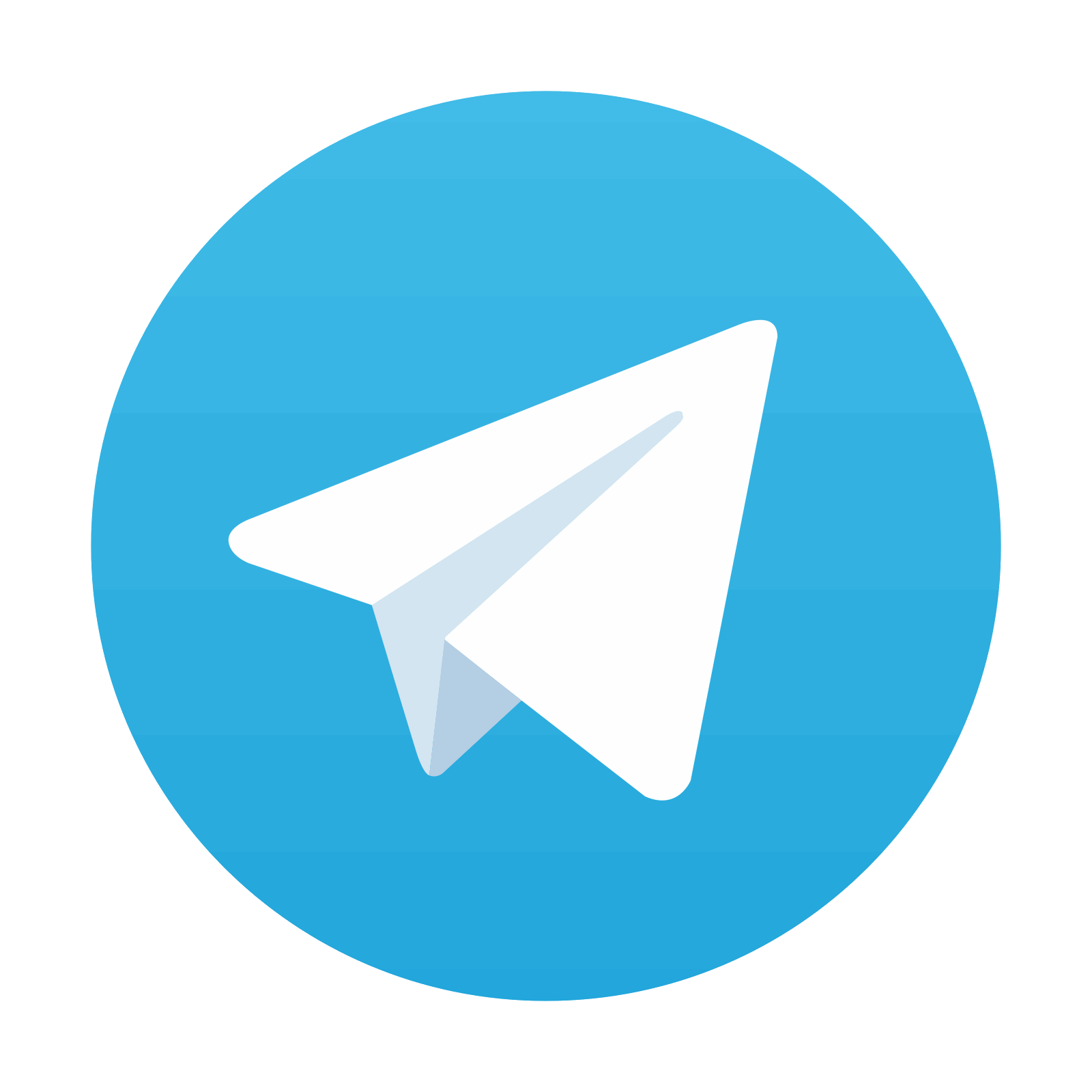
Stay updated, free articles. Join our Telegram channel

Full access? Get Clinical Tree
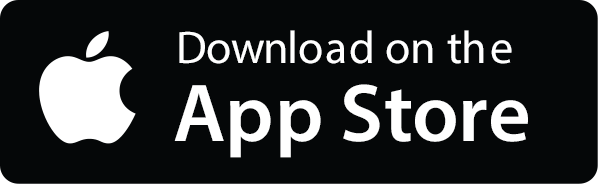
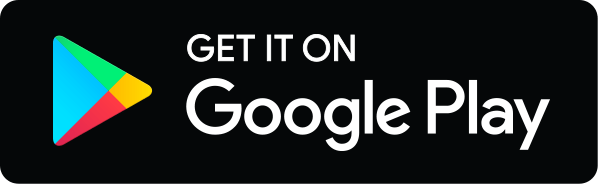
