Introduction to Echocardiographic Assessment of Cardiac Tumors and Masses
- Teerapat Yingchoncharoen, MD
- Allan L. Klein, MD
Cardiac tumors, both benign and malignant, are rare, and the diagnosis is usually challenging. Early recognition and diagnosis of a cardiac tumor by integration of clinical symptomatology and imaging findings could lead to appropriate management in a timely manner. Echocardiography is therefore a highly valuable tool in the assessment of cardiac masses and regarded as the first-line imaging modality. With the advent of three-dimensional echocardiography, the anatomy and spatial relation of cardiac masses can be appreciated without difficulty, enabling more accurate assessment of mass pathology. It is crucial to always differentiate normal cardiac structures or anatomic variants as well as ultrasound artifacts from true pathologies to avoid inappropriate testing and unnecessary surgical procedures.
Cardiac masses can be classified as intra- and extracardiac masses, including primary and metastatic cardiac tumors, pericardial and mediastinal tumors and cysts, nontumor masses, and disorders that mimic tumors including intracardiac thrombi, vegetation, infiltrative disorders, and iatrogenic materials ( Fig. 146.1 ).

Patients with cardiac tumors may present with cardiovascular-related symptoms (obstruction, interference with valvular structures resulting in regurgitation, direct invasion of the myocardium with associated impaired contractility, arrhythmias and conduction disorders, pericardial effusion, or embolization) or constitutional symptoms. However, cardiac tumors are most commonly discovered incidentally during echocardiography performed for other indications.
Primary cardiac tumors are rare across all age groups, occurring with an incidence of less than 0.1% in one series of more than 12,000 autopsies. Approximately 75% of primary cardiac tumors are benign and mostly mesenchymal in origin; cardiac myxoma accounts for more than 50% of them. Of the remaining 25% of primary cardiac tumors that are malignant neoplasms, sarcomas are the most common, followed by lymphomas. The clinical signs and symptoms of primary cardiac malignant neoplasms are typically subtle, given their slow progression. Thus, findings of locally infiltrative or systemically widespread disease can be common at the time of the initial presentation. Primary cardiac tumors may be intracavitary or intramural. Most atrial tumors are intracavitary (clusters of echoes partially filling the cardiac chamber), whereas ventricular tumors are more commonly intramural (localized thickening or nodularity of the myocardium).
Secondary cardiac tumors are 20 to 40 times more common than primary cardiac tumors. The majority of cardiac metastases occur in the pericardium and are usually carcinoma, as opposed to sarcomas. Malignant tumors spread to the heart by (1) direct extension, (2) hematogenous spread, (3) venous spread, and (4) lymphatic spread. Because of increased prevalence, the most common tumor to metastasize , to the heart is lung cancer; however, the tumor with the greatest propensity to metastasize to the heart is melanoma, followed by germ cell tumors and leukemia. Such metastases usually appear on echocardiography as regional wall thickening with, at times, an associated pericardial effusion. A tumor mass may protrude into a cardiac chamber.
Transthoracic echocardiography (TTE) is the primary diagnostic tool for patients with a suspected cardiac mass and can provide information on the appearance, size, shape, extent, and spatial relationship of the mass to adjacent structures, as well as hemodynamic consequences and ventricular function. The reported sensitivity of TTE to detect cardiac mass ranges from 55% to 93% , depending on the size, acoustic properties, and location of the mass. Transesophageal echocardiography (TEE) provides better visualization of cardiac structures than does TTE, especially those at a considerable depth from the chest wall and lesions that involve the left atrial appendage. Of note, TEE and TTE detect atrial myxomas and left-sided cardiac tumors at similar rates. However, TEE is superior to TTE for detecting masses in and around the right cardiac chambers. TEE also provides structural details, such as data on cysts, points of attachment, and the compression or infiltration of adjacent cardiovascular structures, that may be helpful in differentiating malignant from benign masses and facilitating treatment. Biplane TEE affords a clear view into the superior vena cava to demonstrate thrombi attached to pacemaker wires or intravenous lines, as well as other sources of cardiac embolus such as left atrial appendage thrombus and aortic atheromatous plaque. Newer echocardiography techniques using ultrasonic contrast agents may help to differentiate vascularized tumor from a thrombus by demonstrating tissue perfusion of the mass. In addition, contrast echocardiography can help to differentiate benign and malignant tumors, given the fact that malignant and vascular tumors are hyperenhanced whereas stromal tumors and thrombi are hypoenhanced after ultrasonic contrast administration. Three-dimensional echocardiography (3D echo) contributes mainly to an improved assessment of the size, shape, and mobility of a tumor and its relationship to adjacent structures. Cardiac computed tomography (CT) and magnetic resonance imaging (MRI) are complementary to echocardiography for the evaluation of cardiac masses. The larger field of view with CT and MRI allows better visualization of cardiac masses without limitations due to acoustic windows. However, echocardiography is better than CT for detecting small (< 1 mm) and highly mobile cardiac masses because of its higher spatial and temporal resolution. Cardiac CT provides less information regarding tissue characterization than does cardiac MRI; however, CT provides better soft-tissue contrast than echocardiography, may depict calcification and fat, and may allow tissue diagnosis by measuring attenuation value (lipoma). Cardiac MRI offers a unique ability to enable tissue characterization of the cardiac mass based on a particular pattern on T1- and T2-weighted techniques, as well as tissue vascularity and fibrosis on contrast-enhanced imaging and delayed contrast enhancement imaging.
In summary, echocardiography remains the imaging modality of choice to detect cardiac masses given its superior spatial and temporal resolution and accessibility. TEE should be considered in all cases where TTE cannot provide complete assessment of the mass. In selected cases, cardiac CT or MRI may be required to confirm or exclude the diagnosis and further evaluate the extension or severity of the mass. Integration of history, clinical findings, and multimodality imaging findings is the key to the diagnosis and appropriate management.
Primary Benign, Malignant, and Metastatic Tumors in the Heart
- Zoe Yu, MD
- Gillian Murtagh, MD
- Jeanne M. DeCara, MD
- Gillian Murtagh, MD
Cardiac masses are often the subject of case reports because of their rarity. However, because they are so infrequent, they often challenge the diagnostic skills of even the most experienced physician. Improvements in echocardiography, including real-time three-dimensional (3D) echocardiography, as well as cardiac computed tomography and cardiac magnetic resonance imaging, have enabled cardiologists to better refine the differential diagnosis of a cardiac mass.
Cardiac masses are generally first noted antemortem with echocardiography. The sensitivity and specificity of echocardiography for the detection of cardiac masses is difficult to precisely discern because the incidence of cardiac tumors is low, especially for primary tumors. Data from several small case series, however, offer some insight about the diagnostic yield from two-dimensional (2D) echocardiography. For instance, the sensitivity of 2D transthoracic echocardiography for the detection of a pathologically confirmed tumor was 93.3% with a minimal detectable tumor size of 0.5 to 1.0 cm 2 in one series of 149 patients. In the same series, 2D transesophageal echocardiography had a sensitivity of 96.8%.
Three-dimensional echocardiography appears to have incremental yield when used as an adjunct to 2D echocardiography. Its value lies in its ability to provide additional information about the location of a mass, its size, means and point of attachment, and potential approach for surgical resection. In one series, 3D transesophageal echocardiography provided incremental information over 2D echocardiography for the preoperative assessment of 37% of the patients studied and was estimated to be able to do so in approximately 18% of all intracardiac masses. Another way in which 3D echocardiography may be helpful is in determining the size of a cardiac mass. Compared with real-time 3D echocardiography, 2D transthoracic echocardiography has been shown to underestimate the diameter of cardiac masses by as much 24.6%. Similarly, 2D transesophageal echocardiography can underestimate mass diameter by 19.8% compared with real-time 3D echocardiography. This has clinical importance because the diameter of a mass, be it a vegetation, thrombus, or tumor, has important implications for patient prognosis and embolic potential.
Tumor classification and frequency
Cardiac masses can be classified as primary or secondary, benign or malignant, or by their location: atrial, ventricular, or valvular. Tables 147.1 and 147.2 show the relative frequencies of primary benign, primary malignant, and metastatic neoplasms. Figure 147.1 illustrates how the location of a mass may provide a helpful clue to its etiology.
Tumor | Total | Surgical | Autopsy | Age ≤ 15 Years at Diagnosis |
---|---|---|---|---|
Primary Benign Neoplasms of the Heart | ||||
Myxoma | 114 | 102 | 12 | 4 |
Rhabdomyoma | 20 | 6 | 14 | 20 |
Fibroma | 20 | 18 | 2 | 13 |
Hemangioma | 17 | 10 | 7 | 2 |
Atrioventricular nodal | 10 | 0 | 10 | 2 |
Granular cell | 4 | 0 | 4 | 0 |
Lipoma | 2 | 2 | 0 | 0 |
Paraganglioma | 2 | 2 | 0 | 0 |
Myocytic hamartoma | 2 | 2 | 0 | 0 |
Histiocytoid cardiomyopathy | 2 | 0 | 2 | 2 |
Inflammatory pseudotumor | 2 | 2 | 0 | 1 |
Fibrous histiocytoma | 1 | 0 | 1 | 0 |
Epithelioid hemangioendothelioma | 1 | 1 | 0 | 0 |
Bronchogenic cyst | 1 | 1 | 0 | 0 |
Teratoma | 1 | 0 | 1 | 1 |
Totals | 199 | 146 (73%) | 53 (27%) | 45 (23%) |
Primary Malignant Tumors of the Heart | ||||
Sarcoma | 137 (95%) | 116 | 21 | 11 (8%) |
Angio | 33 | 22 | 11 | 1 |
Unclassified | 33 | 30 | 3 | 3 |
Fibrous histiocytoma | 16 | 16 | 0 | 1 |
Osteo | 13 | 13 | 0 | 0 |
Leiomyo | 12 | 11 | 1 | 1 |
Fibro | 9 | 9 | 0 | 1 |
Myxo | 8 | 8 | 0 | 1 |
Rhabdomyo | 6 | 2 | 4 | 3 |
Synovial | 4 | 4 | 0 | 0 |
Lipo | 2 | 0 | 2 | 0 |
Schwannoma | 1 | 1 | 0 | 0 |
Lymphoma | 7 (5%) | 1 | 6 | 0 |
Totals | 144 | 117 (81%) | 27 (19%) | 11 (8%) |
Primary Tumor | Total Autopsies | Metastases to Heart |
---|---|---|
Lung | 1037 | 180 (17%) |
Breast | 685 | 70 (10%) |
Lymphoma | 392 | 67 (17%) |
Leukemia | 202 | 66 (33%) |
Esophagus | 294 | 37 (13%) |
Uterus | 451 | 36 (8%) |
Melanoma | 69 | 32 (46%) |
Stomach | 603 | 28 (5%) |
Sarcoma | 159 | 24 (15%) |
Oral cavity and tongue | 235 | 22 (9%) |
Colon and rectum | 440 | 22 (5%) |
Kidney | 114 | 12 (11%) |
Thyroid gland | 97 | 9 (9%) |
Larynx | 100 | 9 (9%) |
Germ cell | 21 | 8 (38%) |
Urinary bladder | 128 | 8 (6%) |
Liver and biliary tract | 325 | 7 (2%) |
Prostate gland | 171 | 6 (4%) |
Pancreas | 185 | 6 (3%) |
Ovary | 188 | 2 (1%) |
Nose (interior) | 32 | 1 (3%) |
Pharynx | 67 | 1 (1%) |
Miscellaneous | 245 | 0 |
Total | 6240 | 653 (10%) |
* In this series including some of the more commonly encountered malignancies, melanoma is the primary tumor for which metastatic tumors were most commonly found in the heart on autopsy.

Primary benign tumors
Myxomas are the most common adult primary cardiac benign tumors. Symptom presentation can be variable and ranges from subtle to overt. For instance, some patients present only with vague constitutional symptoms, whereas others may experience overt heart failure or syncope secondary to obstruction to ventricular filling in cases where the tumor is sufficiently large. Patients may also present with embolic events, including stroke, thought to arise from the friable surface of the tumor and possibly adherent thrombus. Myxomas are classically described as mobile lobular masses located in the left atrium attached via a pedicle to the interatrial septum, often in the region of the fossa ovalis ( Fig. 147.2 ). Less frequently, they can be located in the right atrium or in the ventricles. Differentiating this tumor from thrombus becomes challenging when the myxoma is sessile and in an atypical location within the atrium. In such cases, it is important to elicit clinical context favoring thrombus formation, such as the presence of atrial dysrhythmias, recent ablation for atrial dysrhythmias, or mitral stenosis. Myxomas are mainly solitary masses, although familial syndromes may be marked by the presence of multiple lesions. Once the diagnosis of myxoma is confirmed, prompt surgical resection yields good results, although there is a 2% to 5% recurrence rate. , Recurrence is often either at the site of the initial myxoma, indicating incomplete resection, or from an additional undiscovered focus. , This underscores the importance of a thorough intraoperative transesophageal echocardiographic examination.

Papillary fibroelastomas are the second most common primary benign neoplasm of the heart. These round and solitary lesions are most commonly located on the aortic valve (44%), followed closely by the mitral valve (35%), and less commonly on the tricuspid valve (15%) and pulmonic valves (8%).The valvular location of papillary fibroelastomas—along with their frondlike surface, the latter giving them a shimmering appearance on echo—distinguishes these tumors from myxomas ( Fig. 147.3 ). Although these masses may be discovered incidentally on echocardiography, embolic events associated with these lesions are known to occur and are more common when the fibroelastoma is mobile and attached to a stalk. Cropping and manipulation of 3D echocardiographic images may aid in the detection of a discrete stalk if not readily apparent on 2D images. The identification of a stalk can be the first clue to help to distinguish a fibroelastoma from other entities in the differential diagnosis such as Libman-Sacks endocarditis, in which the lesion is sessile and along the coaptation point of the valve leaflets. Because of its valvular location, a papillary fibroelastoma may initially be mistaken for vegetation until conditions predisposing to endovascular infection have been excluded. Unlike vegetations, which may be irregularly shaped and disrupt valve function, papillary fibroelastomas appear more discrete and do not tend to cause valve regurgitation or stenosis. A Lambl excrescence may also be in the differential diagnosis of a papillary fibroelastoma; however, the former have a wispy, fibrolinear nature, while the latter are round and well formed.

In children, rhabdomyomas and fibromas are the most common benign tumors; yet, despite their benign nature, they can be associated with conduction abnormalities, arrhythmias, and even sudden death. Unlike myxomas, these neoplasms are commonly located in the ventricular myocardium. Rhabdomyomas tend to be small and may be multiple or solitary in nature. Fibromas are larger tumors, and as a result, many patients are symptomatic. Fibromas ( Fig. 147.4 ) tend to be hyper-reflective on echocardiography, whereas other rare benign masses such as hemangiomas ( Fig. 147.5 ) are highly vascular tumors and may show evidence of perfusion on contrast echocardiography.


Primary malignant tumors
Cardiac sarcomas are the most common primary malignancies of the heart. These include (in order of frequency) angiosarcoma, rhabdomyosarcoma, fibrosarcoma, and leiomyosarcoma. It is their large size and predilection for the right atrium that point toward this diagnosis ( Fig. 147.6 ). These tumors often take the form of broad-based, lobular masses; however, they may alternatively be polypoid or intramyocardial and can extend into the inferior vena cava. Calcification may be noted in the case of osteosarcomas. Cardiac sarcomas are aggressive tumors that spread rapidly from an intramural location into the cardiac chambers and/or pericardium. They tend to manifest with obstructive symptoms associated with impaired right heart filling, pericardial effusion, or tamponade. Arrhythmias or embolization may also occur. By the time of diagnosis, metastases are often present. Rhabdomyosarcomas are more common in children and are found in both ventricular and atrial locations. Though optimal management of sarcomas includes chemotherapy and radiotherapy and complete surgical resection, this is frequently not possible owing to their dimensions and intramyocardial location. Overall, the prognosis remains very poor; median survival is 6 to 12 months.

Sarcomas must be differentiated from primary cardiac lymphomas. Although metastatic lymphoma is much more common than primary cardiac lymphoma, the latter, like sarcomas, has a predilection for the right heart, particularly the right atrium. Morphologically, they do not have a classic pattern. However, an associated pericardial effusion is not uncommon. Surprisingly, constitutional symptoms typical of lymphoma are not usually present. Patients instead may complain of precordial pain or dyspnea. The majority of reported primary cardiac lymphomas have been of the diffuse large B cell variety. , In contrast to sarcomas, a favorable response to surgery and/or anthracycline-based therapy has been reported for primary cardiac lymphomas when detected early with cardiac imaging. Therefore, obtaining a definitive pathologic diagnosis can be important in determining prognosis and treatment options when a primary malignant cardiac tumor is suspected.
Metastatic tumors
Metastatic disease has been found in 10% to 25% of patients in autopsy series of patients with cancer. , In the majority of clinical cases, metastatic disease is known before cardiac involvement is discovered on echocardiogram. Symptoms may include embolic events, congestive heart failure in cases where intramyocardial extension has compromised systolic function, pericardial tamponade, intracavitary obstruction, or arrhythmia. Cardiac metastatic foci may present as multiple or solitary lesions. There are a variety of mechanisms for cardiac metastasis, including direct invasion from the mediastinum, hematologic spread, lymphatic spread, or venous extension into the heart. Metastatic foci in the right heart commonly originate from extension of the tumor from the inferior vena cava. This can be observed with renal cell carcinoma, leiomyosarcoma, and some gynecologic malignancies. In the left heart, the pulmonary veins may serve as a conduit for lung cancer extension into the heart ( Fig. 147.7 ). Not only do solid tumors metastasize to the heart, but hematologic malignancies may also become manifest in the heart, particularly in the pericardium or myocardium. For lymphoma, this may involve actual tumor foci on the pericardium or myocardium with clinical manifestations, whereas leukemic infiltration of the heart may be subtler, even though cardiac metastasis is reported to be more common. Generally, metastatic disease involving the heart, regardless of the primary malignancy, is a sign of widely disseminated disease and associated with a poor prognosis. In most cases treatment is largely palliative.

Summary
Cardiac tumors are rare clinical entities that challenge our diagnostic acumen. Tumor features such as location, size, and means of attachment as well as clinical context are important clues to refining the differential diagnosis. Although most masses are first discovered with 2D echocardiography, real-time 3D echocardiography may have an additive role in determining the potential etiology of a cardiac mass. By nature of its volumetric data acquisition, 3D echocardiography can yield potential prognostic information by yielding information on the size of a mass as it relates to embolic potential. In addition, 2D and 3D transesophageal echocardiography is of value in defining potential surgical approaches whenever resection is planned.
Left Ventricular Thrombus
- Amr E. Abbas, MD
- Steven J. Lester, MD
The presence of a thrombus in the left ventricular (LV) cavity would at first glance seem to defy the strongly held triad of Virchow. As such, thrombus in the LV usually occurs in the presence of a markedly reduced ejection fraction (EF) and regions of intracavitary flow stasis. There are a number of clinical predispositions that increase the likelihood for LV thrombus formation ( Box 148.1 ). Transthoracic echocardiography (TTE) is the principal clinical tool used to assess for LV thrombus. All at-risk patients should be evaluated, as identification reveals risk for thromboembolic complications.
Left ventricular thrombus with impaired left ventricular function (more common)
LV apical aneurysm
Following myocardial infarction with apical akinesis
Following myocardial infarction in segments other than LAD territory
LV pseudoaneurysm
Dilated cardiomyopathy
Apical ballooning (takotsubo) syndrome
Left ventricular thrombus with preserved left ventricular function (extremely rare)
Hypercoagulable states
Antiphospholipid antibody
Protein C deficiency
Myeloproliferative disorders
Essential thrombocythemia
Myelofibrosis
Salmonella septicemia
Cardiac trauma
Eosinophilic endocarditis
Connective tissue disease
Systemic lupus erythematosus
Behçet disease
On the surface of LV tumors
On device leads that inadvertently migrate to the LV
Administration of large doses of erythropoietin
Left ventricular thrombus with impaired left ventricular function
A thrombus within the LV can be noted within 24 hours after acute myocardial infarction (AMI) and 90% of the time is formed at a maximum of 2 weeks following the event, with the risk highest within the first 3 months ( Fig. 148.1 ). Traditionally the antemortem incidence of LV thrombus following an AMI has been reported to be between 7% and 46%, depending on the diagnostic modality used for detection. However, the contemporary literature reports a declining incidence of LV thrombus post AMI, primarily attributed to the use of primary percutaneous coronary intervention (PCI); incidence may vary between 5% and 15% in patients receiving such treatment. LV thrombus usually occurs following AMI in the left anterior descending artery (LAD) territory and is much less common following infarction in non-LAD territories. About 11% of clots may occur in the septal wall and 3% may occur in the inferior wall, particularly when the infarct extends to the lateral wall.

A left ventricular ejection fraction (LVEF) less than 40%, multivessel coronary artery disease, high creatine kinase levels, and a decreased mitral E-wave deceleration time are associated with increased evidence of clot. , In addition, Doppler analysis of apical flow patterns has been suggested to help identify which of these patients are at highest risk of thrombus formation. Evidence of apical flow stasis or of continuous swirling of flow around the apex is thought to identify patients at particular risk for apical thrombus. In the presence of a pseudoaneurysm, the clot occurs lining the LV wall at the site of rupture contained by the pericardium.
The use of angiotensin-converting enzyme inhibitors following AMI has not been associated with a decreased incidence despite improved remodeling, and the effect of beta-blocker is controversial, from harmful to neutral. It has been demonstrated that levels of soluble tissue factor, D-dimer, and anticardiolipin are increased in patients with LV clot following AMI. However, the usefulness of these levels in diagnosis is not known.
On the other hand, in patients with cardiomyopathy ( Fig. 148.2 ), an EF less than 20% provides the highest risk with a reported incidence of almost 50%. The presence of mitral regurgitation appears to be protective in these patients against developing clot. However, a similar association has not been demonstrated following AMI.

Left ventricular thrombus with preserved left ventricular function
Thrombus within the LV cavity has been reported in patients with preserved LV function. This occurs rarely in the setting of endothelial damage as with trauma, eosinophilic endocarditis ( Fig. 148.3 ), tumors, device leads ( Fig. 148.4 ), or in the setting of hypercoagulable states. The treatment is targeted to the underlying pathology, along with the use of anticoagulation.


Diagnostic tests and the role of echocardiography
A space-occupying mass in the LV can be classified as either neoplastic or non-neoplastic. An LV thrombus is an example of a non-neoplastic mass, and although echocardiography does not provide a histological diagnosis, certain features, when present, suggest the mass to be a thrombus. A mass noted in a region of the LV where there is a wall motion abnormality, that is avascular (based on either color Doppler or use of contrast), and in an apical location suggests thrombus as its etiology. A fresh thrombus often protrudes into the LV cavity and is highly mobile, whereas more chronic thrombi are often sessile in appearance.
The predictive accuracy of TTE for the detection of LV thrombus is dependent on clear delineation of all endocardial borders. Therefore, meticulous imaging is imperative, and one should have a low threshold for the use of echocardiographic contrast if endocardial border delineation is suboptimal. The sensitivity and specificity to detect an LV thrombus have been reported as 92% to 95% and 86% to 88%, respectively. The diagnostic ability of echocardiography is increased in the presence of large protuberant clots. False tendons, trabeculations, and misplaced papillary muscles can all cause a false-positive result. Conversely, poor endocardial border delineation, small clot size, mural thrombus, and image foreshortening may yield a false-negative result.
Cardiac magnetic resonance imaging has an increased sensitivity to detect LV thrombus and should be considered when the TTE evaluation is equivocal or suboptimal. , Transesophageal echocardiography, like all other imaging modalities, provides good specificity in the detection of LV thrombus but has a much lower sensitivity (35% to 40%).
Prognosis and treatment
When present, LV thrombus poses risk for thromboembolic complication, with stroke being the most feared. Clot protrusion and mobility have been associated with risk of embolization. Other findings associated with increased risk for embolization include heart failure, atrial fibrillation, advanced age, previous emboli, and hyperdynamic adjacent segment. Apical aneurysm may be protective because of lack of contractility and less chance of dislodgement. Layered or sessile thrombi have a lower thromboembolic potential.
Once a thrombus is identified, anticoagulation is the mainstay of therapy, with serial imaging to evaluate for thrombus regression or organization. The duration of anticoagulation is a minimum of 3 months according to the 2013 ACCF/AHA guidelines ; however, duration is influenced by the underlying condition and depends on whether serial imaging shows thrombus resolution and/or organization. In addition, management of the underlying predisposing condition, as with immunosuppressive therapy in patients with connective-tissue disease and/or eosinophilic endocarditis, is essential.
The prognosis of patients with LV thrombus is highly dependent on the causing factors and the extent and severity of LV dysfunction.
Summary
LV thrombus occurs most commonly in the presence of decreased LV systolic function but in certain conditions may occur in those with preserved LVEF. TTE is the principal noninvasive diagnostic tool used for detection, with contrast echocardiography increasing both its sensitivity and specificity. Thrombi that protrude into the lumen of the LV and are highly mobile have the highest thromboembolic potential. Current treatment is primarily focused on management of the underlying predisposing condition along with anticoagulation.
Left Atrial Thrombus
- Yoram Agmon, MD
- Jonathan Lessick, MD, DSc
- Shimon A. Reisner, MD
- Jonathan Lessick, MD, DSc
Left atrial (LA) thrombi are a major cause of cerebral and systemic embolism. Transesophageal echocardiography (TEE) is the method of choice for diagnosing an LA thrombus. The diagnosis or exclusion of an LA thrombus has become a common indication for performing TEE in routine clinical practice, particularly in the evaluation of a cardiac source of embolism or before cardioversion.
Pathogenesis of left atrial thrombus formation
A thrombus forms within the left atrium or LA appendage (LAA) primarily as a result of blood stasis within this cavity, though other component of the Virchow triad (a procoagulant state and endocardial damage) may also play an additive role, especially in atrial fibrillation (AF). LA stasis is frequently related to LA and LAA contractile dysfunction in association with atrial arrhythmias (AF or atrial flutter [AFL]). Notably, LA contraction is highly variable in patients with atrial arrhythmias, varying from near-normal contraction to complete atrial standstill. This heterogeneity is the result of multiple factors that jointly affect LA function, including the type of arrhythmia (in general, better preserved contraction in AFL compared to AF), the duration of the arrhythmia (worse contraction in longstanding arrhythmias), and additional factors that promote atrial stasis via elevation of LA pressure and/or LA dilatation—left ventricular (LV) dysfunction (diastolic dysfunction, with or without systolic dysfunction) and mitral valve disease (mainly mitral stenosis [MS] or presence of a mitral prosthesis).
An LA thrombus is very infrequently demonstrated in TEE examinations performed in patients with sinus rhythm (SR). Nevertheless, it may occasionally occur in SR in the presence of severe LA stasis (due to the causes listed earlier) or following a transient episode of AF, when TEE is performed after cessation of the arrhythmia. In patients with paroxysmal AF, LA thrombus may form during the course of the arrhythmia or during the initial period following its termination, due to transient LA dysfunction (“stunning”) occurring after conversion from AF to SR (a phenomenon that is variably observed after electrical or drug-induced cardioversion, as well as following spontaneous conversion to SR). LA dysfunction in the absence of an atrial arrhythmia may also be observed in patients with cardiac amyloidosis, due to a combination of amyloid infiltration of the LA and LV myocardium, causing LA contractile dysfunction and severe LV diastolic dysfunction.
Interventional procedures involving the left atrium, including surgical or catheter-based ablation of AF (causing endocardial damage within the left atrium), LA incisions (during mitral valve surgery), or implantation of LA devices (interatrial septal or LAA occluder devices), may also be complicated by thrombus formation within the LA cavity.
Most LA thrombi form within the LAA, because of its narrow cul-de-sac morphology that predisposes to blood stasis. Thrombi within the main LA cavity are less frequently present, and their occurrence depends on the underlying cardiac substrate. Whereas the vast majority of thrombi associated with nonvalvular AF are located within the LAA, thrombi within the LA cavity itself are not infrequently seen in patients with valvular AF or in patients with nonvalvular AF and severe LV dysfunction. These findings may have implications for LAA closure, which may be less effective in the latter subgroups of patients with AF.
Transesophageal echocardiographic diagnosis of left atrial thrombus
TEE is an ideal modality for imaging the left atrium, which is a posterior cardiac structure adjacent to the esophagus. The LAA and main LA body should be scanned separately for the diagnosis of thrombi. The LAA, the most common location of LA thrombus formation, should be meticulously scanned because of its highly variable morphology and the common presence of multiple lobes. , Scanning should be performed from the midesophageal probe position with mild TEE probe anteflexion. To fully delineate the LAA cavity and its multiple lobes, the scanning plane angle should be gradually changed from 0 degrees to approximately 140 to 150 degrees, the latter usually allowing visualization of the LAA in its widest dimension.
The main LA cavity, a much less common location of LA thrombi, should be routinely scanned in a vertical (90-degree) scanning plane with gradual clockwise rotation of the TEE probe from the left pulmonary veins (adjacent to the LAA) to the right pulmonary veins. When indicated, vertical scanning may be supplemented by horizontal (0-degree) scanning, with a gradual inferior-to-superior outward motion of the TEE probe, from the bottom to the upper part of the LA body.
An LA thrombus appears as an intraluminal mass of variable size and morphology, with an echogenicity that is typically heterogeneous and lower than that of the surrounding LA walls ( Fig. 149.1 /Video 149.1, A and B , and Fig. 149.2 /Video 149.2, A-D ), although a longstanding organized thrombus can appear more echogenic and may occasionally calcify. Once diagnosed, the embolic potential of an LA thrombus should be assessed and reported, based on its size, its morphology, the extent of its connection to the LA wall, and its mobility (a flat, immobile mural thrombus vs. a protruding mobile thrombus connected to the LA wall by a narrow stalk, or the rare occurrence of a free-floating thrombus within the LA cavity in a patient with MS).
Comprehensive scanning of the left atrium and LAA, as recommended previously, will generally overcome several diagnostic pitfalls, including (1) incorrectly diagnosing prominent pectinate muscles as LAA thrombi ( Fig. 149.3 /Video 149.3, A , B ); (2) overlooking the presence of a thrombus in a multilobed LAA; (3) incorrectly interpreting various artifacts as representing thrombi (acoustic shadowing; see Fig. 149.3 , D , and reverberation artifacts); and (4) limited-quality near-field imaging of the LA body adjacent to the TEE probe.
In addition to the presence of LA thrombus, additional echocardiographic features associated with high thromboembolic risk should be assessed, including the presence and severity of spontaneous echocardiographic contrast (SEC) and, in selected cases, a quantitative evaluation of LAA contractile function. Although attempts to semiquantify the degree of LA SEC have been previously reported, it is practical and clinically sufficient to categorize SEC as either mild or severe (“dense”) ( Fig. 149.4 , A /Video 149.4, A ). Because the appearance of SEC may be significantly affected by various machine settings, mainly gain, the image quality of the surrounding LA walls and adjacent cardiac structures should be optimized in order to avoid overestimation or underestimation of SEC severity. Importantly, the presence of dense SEC warrants a systematic scanning of the LAA for the presence of thrombus, more than so than in its absence. By contrast, because dense SEC is almost uniformly noted in patients with a LA thrombus, the absence of SEC should raise doubts regarding the diagnosis of LA thrombus and mandates additional scanning to exclude the possibility of a false-positive diagnosis of thrombus due to artifacts.
At times, severe SEC appears as “sludge,” and it may be difficult to differentiate sludge from a “soft” thrombus (see Fig. 149.4 , B and C /Video 149.4, B ). From a safety perspective, in patients with AF who are candidates for cardioversion, the presence of LA sludge should be considered as representing a thrombus, thereby contraindicating cardioversion.
LAA contractile function may be assessed by pulsed Doppler interrogation of flow at the proximal LAA cavity, adjacent to the LAA orifice, or measurements of LAA wall motion velocities by tissue Doppler. Although extensively studied and highly educational in our understanding of atrial pathophysiology, the indication for quantitatively assessing LAA function in clinical practice is limited and should be reserved mainly for patients in whom the diagnosis of an LAA thrombus is unexpected or questionable. Severe impairment of LAA contraction is occasionally observed in patients with SR, mostly in association with severe LV dysfunction ( Fig. 149.5 ) or MS, and this finding may support the diagnosis and provide a pathophysiological explanation for the relatively uncommon finding of an LAA thrombus in the absence of a previously documented atrial arrhythmia. In the presence of atrial arrhythmias, preserved LAA contraction (and absence of severe SEC) decreases the chances that an LAA thrombus will be detected, and any apparently abnormal LAA masses are more likely to present normal variants (pectinate muscles) or artifacts. Although the assessment of LAA contraction may prove useful in predicting the likelihood of immediate success of cardioversion and long-term maintenance of SR, as well as in the overall assessment of thromboembolic risk in patients with AF, its role in clinical decision making is questionable.

Three-dimensional (3D) TEE may nicely demonstrate LAA and LA thrombi ( Fig. 149.6 /Video 149.6, A and B ), although the incremental value of 3D imaging in the diagnosis of LA thrombi remains to be determined. Biplane imaging using the 3D matrix TEE probe is helpful in delineating the multiple lobes of the LAA and helps differentiate normal pectinate muscles from thrombi (see Fig. 149.3 , C /Video 149.3, C ). 3D imaging of the LAA with multiplanar reconstruction along its cavity may also be used for this purpose. En face 3D imaging of the LAA orifice from the LA body perspective, including 3D color flow imaging, may aid in the diagnosis of incomplete surgical ligation or percutaneous occlusion of the LAA orifice, which are associated with an increased risk of thromboembolism (Video 149.5). 3D TEE may also be of help in imaging of device-associated intra-LA thrombosis following implantation of atrial septal or LAA occlusion devices, although the overall importance of 3D TEE imaging in these specific niches is currently uncertain.
Additional diagnostic techniques
Transthoracic Echocardiography and Contrast Echocardiography
At times, large LA or LAA thrombi may be diagnosed using TTE ( Fig. 149.7 and Video 149.7, A ), although TTE is insensitive for the diagnosis of most small-to-moderate size thrombi commonly located within the LAA. In a small pilot multicenter trial of 118 patients with AF of more than 2 days’ duration, the findings on TTE imaging of the LAA, which included intravenous contrast administration and measurements of LAA walls velocities by tissue Doppler, were compared with TEE images, considered the diagnostic gold standard. TTE imaging with contrast correctly identified the two patients with thrombi, whereas low tissue-Doppler velocities of the LAA wall were associated with the presence of severe LAA SEC, sludge, or thrombus. Although encouraging, these preliminary results need to be adequately confirmed in larger studies before TTE imaging with contrast and/or tissue Doppler is introduced into clinical practice. The addition of intravenous contrast may potentially improve the diagnostic accuracy of a TEE examination by excluding thrombi in nonconclusive examinations and identifying previously unsuspected LAA filling defects. However, the additive value of contrast TEE over a standard noncontrast TEE examination, which is very accurate in experienced hands, needs to be proven before contrast TEE use can be recommended.
Cardiac Computed Tomography and Magnetic Resonance Imaging
LA and LAA thrombi may be detected by cardiac computed tomography (CT) ( Fig. 149.8 ), but CT is rarely the diagnostic procedure of choice for diagnosing or excluding an LA thrombus in clinical practice. Nevertheless, CT may be of clinical use in cases in which TEE is contraindicated, not feasible, or nondiagnostic, and LA thrombi are occasionally detected by CT in scans performed for other indications.

The accuracy of CT angiography in detection of LAA thrombus has been examined in at least 19 studies involving approximately 3000 patients, in various patient populations (mostly in patients with stroke or before ablation procedures for AF) using different imaging protocols. It has consistently been found to be highly sensitive (~ 100%) for the identification of LAA thrombus. However, its specificity varies considerably, depending on the technique and the patient population. This translates to a negative predictive value of 100% and a positive predictive value between 25% and 100% in different studies.
Although LA thrombus can be detected by a non-gated examination, the standard cardiac CT procedure is electrocardiography-gated. It is performed during or immediately following a contrast bolus, triggered by contrast medium reaching either the LA or thoracic aorta. This usually results in excellent contrast between the intracardiac blood and the cardiac tissues, assuming rapid mixing of the blood within each cavity. In the presence of sluggish flow with the LAA, such as that occurring in the context of atrial fibrillation and visualized as SEC by TEE, this mixing may not be complete and may result in a false-positive diagnosis of an LAA filling defect as being a thrombus. Several solutions to improve the diagnostic accuracy of CT have been introduced, including (1) scanning at a late phase, to test whether any filling defect is persistent (indicating a thrombus) or transient (indicating slow flow); and (2) measuring the CT enhancement in the suspected area within the LAA (see Fig. 149.8 ).
Magnetic resonance imaging (MRI) is also highly sensitive for the identification of intracardiac thrombi, using both noncontrast and contrast-enhanced approaches with various imaging sequences. To date, few studies have examined the use of MRI for specifically detecting LA thrombus, and the role of this imaging modality in this context remains to be determined.
Clinical implications of the diagnosis of left atrial thrombus by transesophageal echocardiography
The appropriate use criteria for TEE in diagnosing LA thrombus, adopted by multiple cardiac imaging societies (including the American Society of Echocardiography), are presented in Box 149.1 .
* Appropriateness scores in parentheses (7-9 = appropriate; 4-6 = uncertain; 1-3 = inappropriate).
for the Use of Transesophageal Echocardiography for Diagnosis of Left Atrial Thrombus- 1.
TEE as initial test—AF/AFL
- a.
Evaluation to facilitate clinical decision making with regard to anticoagulation, cardioversion, and/or radiofrequency ablation Appropriate (9)
- b.
Evaluation when a decision has been made to anticoagulate and not to perform cardioversion Inappropriate (2)
- a.
- 2.
TEE as initial or supplemental test—embolic event †
† Evaluation for various cardiovascular sources of embolism, including an LA thrombus.
- a.
Evaluation for cardiovascular source of embolus with no identified noncardiac source Appropriate (7)
- b.
Evaluation for cardiovascular source of embolus with a previously identified noncardiac source Uncertain (5)
- c.
Evaluation for cardiovascular source of embolus with a known cardiac source in which a TEE would not change management Inappropriate (1)
- a.
- 3.
TEE as initial or supplemental test—general uses
- a.
Reevaluation of prior TEE finding for interval change (e.g., resolution of thrombus after anticoagulation) when a change in therapy is anticipated Appropriate (8)
- a.
AF, Atrial fibrillation; AFL, atrial flutter; TEE, transesophageal echocardiography.
Cardiac Source of Embolism
TEE is commonly used to identify a potential cardiovascular source of embolism in patients suffering a cerebral or systemic ischemic event that is compatible with an embolic mechanism by its clinical presentation and radiologic findings. The appropriate use criteria support this approach when an alternative noncardiac source is not identified and when the identification of a cardiovascular source will affect treatment. LA thrombus is most frequently detected in patients with ischemic events in association with AF (paroxysmal or persistent). In this setting, lifelong anticoagulation is strongly indicated even without the demonstration of an LA thrombus by TEE. Therefore, a TEE examination will not affect treatment and is not clinically indicated in this scenario.
Whether TEE is useful in patients with thromboembolism in the absence of documented atrial arrhythmias is a matter of debate. LA thrombus may infrequently form in the absence of AF or AFL in the presence of the hemodynamic risk factors that predispose to LA stasis. These high-risk features can be easily identified by a TTE examination, and therefore, TEE may be selectively reserved for patients judged to be at high risk according to the TTE findings. It is conceivable that spontaneous termination of atrial arrhythmias could lead to LA thrombus formation and embolism, but, because of their transient nature, they may not be documented at or before the embolic event. Thus, the possibility of “occult” AF may still justify the routine use of TEE for all patients with thromboembolism and no proof of previous atrial arrhythmia. The frequency of LA thrombi in patients with SR at the time of the echocardiographic examination has been examined in a large series of more than 20,000 consecutive TEE examinations performed for various indications, in which 380 LA thrombi were detected, mostly in patients with AF. LA thrombi were evident in only 20 patients who had SR during TEE (0.1% of all TEE examinations) and, of these, 19 patients were clearly at risk for LA dysfunction and thrombus formation due to high-risk hemodynamic features or previously documented AF. Thus, it appears that LA thrombus formation due to occult AF is an extremely rare phenomenon, which may not justify the nonselective use of TEE to rule out an LA thrombus in all patients with thromboembolism. Nevertheless, because TEE may detect other potential cardiovascular sources of embolism, it is commonly performed in patients with ischemic events, especially in those who are relatively young, without an obvious noncardiac cause of the event, and in whom TEE findings may potentially affect treatment.
Cardioversion of Atrial Fibrillation
TEE has become a standard procedure before cardioversion in patients who are not sufficiently anticoagulated (therapeutic anticoagulation for at least 3 to 4 weeks) at the time of planned cardioversion. TEE-guided cardioversion is very safe (similar low risk of thromboembolism and a lower risk of hemorrhage, compared with prolonged pre-cardioversion anticoagulation) and is associated with higher rates of initial success of cardioversion, although there is no advantage with this approach in long-term maintenance of SR or quality of life. Various clinical patient factors are associated with a higher chance of identifying an LA thrombus by TEE in patients with AF, including the individual and overall number of the clinical components of the CHADS 2 thromboembolic risk score, although, because of the limited accuracy, the use of clinical risk assessment as an alternative to pre-cardioversion TEE cannot be advocated.
Right Heart Thrombi
- Vincent L. Sorrell, MD
- Vrinda Sardana, MD
- Steve W. Leung, MD
- Vrinda Sardana, MD
Right heart thrombi (RHT) are likely more commonly present than what is seen in routine echocardiography because these thrombi are frequently asymptomatic and require more careful evaluation than what is conventionally performed. The actual incidence of RHT is unknown, but appears to be increasing due to a combination of improved diagnostic capabilities that are able to detect smaller RHT, coupled with an increasing use of right heart catheters, pacemakers, and other devices. This chapter reviews the etiologies and characteristics of RHT, provides insight into how to differentiate them from other cardiac masses, offers suggestions on how to optimize echocardiographic acquisition and interpretation of RHT, and concludes with a brief overview on the various treatment options that exist.
Incidence
It is difficult to determine the total incidence of RHT, but some at-risk clinical populations have recently been studied, and the reported incidence of RHT serves as an estimate for the general population. RHT have been reported in 4% to 18% of cases with acute pulmonary thromboembolism (PTE). , The reported variability is likely a result of the use of inconsistent definitions of RHT, the inclusion of both symptomatic and asymptomatic patient populations, assorted diagnostic strategies, and diverse right heart device types and locations.
When associated with PTE, RHT are believed to be residual thrombi in transit from the deep venous system to the pulmonary vasculature. Other thrombi likely form in situ within the right heart and might be overlooked in reports in patients with PTE. In addition, a myriad of modifiable and nonmodifiable risk factors are known to predispose patients to the development of RHT; knowledge of these risk factors is important to ensure image acquisition, and interpretation is performed with increased scrutiny to allow earlier detection of RHT ( Table 150.1 ).
Patient | Disease | Device | Drug | Site |
---|---|---|---|---|
Hypercoagulable Protein C/S def Thrombophilic Age (≥ 65 yrs) Male Trauma/surgery Obesity Immobility Pregnancy | Cardiomyopathy ARVD/C CHF Malignancy AFib COPD Cor pulmonale RV contusion RV infarct Chronic HD IBD Behcet | CVC (size, type) Pacemaker (especially temporary) Multiple PICC size | Amphotericin B TPN No prophylaxis Cigarettes Contraceptives | IJ Location SV location Distal position Duration (> 6 d) |
Risk factors associated with right heart thrombi
Central venous catheters (CVCs) have become an essential component of medical care, and patients in this common at-risk subgroup have been adequately studied to report that they are at a sixfold increased risk of RHT, with incidence rates between 35% and 67%.
In a large prospective study of patients with CVCs, diagnostic ultrasound was performed within 24 hours of catheter removal, and an RHT was found in 33% of these routine patients (10% and 42%; subclavian and internal jugular locations, respectively). Importantly, these thrombi were not occlusive in most patients (97%). PTE occurs in up to one third of patients with upper extremity deep venous thrombosis and in approximately 15% of patients with CVC-related upper extremity deep venous thrombosis, which is associated with an appreciable risk of PTE and death.
Mechanical circulatory support devices are increasingly being used as a strategy to manage patients with heart failure. Little is known about the frequency of RHT associated with these new devices, but because of the underlying pathophysiology, poor myocardial function, and the trauma associated with cannula placement, it is likely that the risk of RHT is also significant despite routine anticoagulation.
Special at-risk clinical populations
Atrial Fibrillation
Atrial fibrillation (AF) has become an epidemic in the elderly population. The development of right atrial appendage (RAA) thrombi in the setting of AF is sometimes forgotten ( Fig. 150.1 /Video 150.1). It is well appreciated that thrombi form in the left atrial appendage (LAA) and increase the likelihood for systemic embolization and stroke, which results in the common practice of patients undergoing transesophageal echocardiography (TEE) before elective cardioversion. The RAA, despite having a wider mouth and less deep recesses, is also subject to low flow, spontaneous echo contrast (SEC), and thrombus development in AF, which warrants careful evaluation. In a judiciously performed TEE investigation of patients with AF, the RAA (and RA) was found to have a larger area, a lower ejection fraction, and a lower emptying velocity than the control group. Although the incidence of RAA thrombi was lower than LAA thrombi, the authors still found RAA clots in more than half (n = 6) of the patients with LAA thrombi (N = 11).
In a smaller study of patients with atrial flutter, these authors did not find any RAA thrombi despite finding an equal incidence of SEC in the LAA and RAA. This was likely due to differences in the incidence of thrombi between fibrillation and flutter, sample size (only 3 LAA thrombi), and the type of TEE probe used (34% mono- or biplane). It has also been reported that RAA thrombus may rarely be seen in the absence of LA thrombi.
Isolated SEC in the RA is a marker of more organized embolic potential. Compared with patients in AF without SEC who had a 7% incidence of pulmonary defects on pulmonary scintigraphy, patients with SEC had a 40% incidence of perfusion defects despite having similar left ventricular (LV) size and function, as well as similar D-dimer and fibrinogen serum thrombotic markers.
Inflammatory Bowel Diseases
Inflammatory bowel diseases (IBDs) are associated with a threefold increased risk for the development of venous thromboembolism (15-fold relative increase during disease flares). This was demonstrated in a review of the literature that identified more than 70 cases of thromboembolic disease in patients with ulcerative colitis or Crohn disease. The reason for this increased risk is multifactorial and related to prothrombotic alterations in the coagulation milieu and associated clinical comorbidities.
Children
Similar to the reported association of outcomes in adults based on the morphologic type of RHT, 50 children were accurately stratified into high- or low-risk types by the appearance of RHT on echocardiography. High-risk features were snake-shaped, mobile, size greater than 2.0 cm (any dimension), or pedunculated RHT, which carried a mortality of greater than 16.0% (3 of 18 children) versus 0% (0 of 32 children) in the low-risk children who lacked these echocardiographic features.
One hundred twenty-two cases were reported in a meta-analysis of RHT (all in the RA) in children (35 publications; mostly infants and neonates). More than 90% were associated with CVCs; more than 25% were postoperative, and nearly 20% were in patients with malignancies. More than half were incidentally detected on echocardiography, were asymptomatic, and had good outcomes.
Right heart thrombus imaging by echocardiography
Echocardiography is a portable, real-time, noninvasive, nonirradiating, immensely comprehensive diagnostic tool with proven value in detection of intracardiac thrombi. It provides immediate, real-time, cross-sectional details on the size, shape, mobility, location, and attachment site of the thrombus. Serial echocardiographic examinations are useful when the clinical status deteriorates and may demonstrate thrombus that was not detected on the initial examination. Two-dimensional transthoracic echocardiography (TTE) is regarded as the primary diagnostic imaging technique for the evaluation of cardiac mass lesions.
A TEE probe is more sensitive at visualizing small thrombi and may be more useful to visualize the superior vena cava and inferior vena cava and follow them into the right atrium. The diagnostic sensitivity to detect RHT is considered 97%, whereas the sensitivity of TTE, compared with TEE, is 50% to 60% with a false negative rate as high as 60%. , This is most evident in patients with congenital heart diseases, postcardiac surgery, and in patients with intracardiac lines and pacemakers. TEE is also superior to TTE for differentiating normal embryonic remnants in the RA.
Echocardiography will most likely remain the initial diagnostic tool for most patients with known or suspected cardiovascular pathology, and RHT in particular, despite expanded capabilities and use of cardiac magnetic resonance (CMR) and computed tomography (CCT) imaging. Recent advancements in modern ultrasound transducers provide phenomenal image quality and improved RHT detection. Manufactured ultrasound contrast agents (UCAs) are available to search for filling defects and further enhance the diagnostic acumen ( Fig. 150.2 /Video 150.2).
These technical developments are occurring at the same time that the morphology of the right heart is experiencing a renaissance with a much greater frequency of use of indwelling catheters, pacemakers, and other temporary, prolonged, or permanent intracardiac devices. These two factors increase the likelihood for both thrombus detection and thrombus development.
Optimal imaging of the right heart is a key to diagnosis and necessitates additional echocardiographic views in combination with routine TTE. It is important to combine M-mode, two-dimensional (2D), and 3D acquisitions from parasternal, apical, and subcostal views, aligned toward the right side, with physiologic data obtained with pulsed wave, continuous wave, color flow, and tissue Doppler findings. Because the presence of right ventricular (RV) dilation or dysfunction is considered when determining the optimal treatment strategy, an understanding of the various complexities unique to the RV anatomy is required.
The subannular interventricular (RV/LV) ratio is a measurement that has been recently used in contemporary device management clinical trials of patients with documented pulmonary emboli. To date, a ratio of greater than 1.0 is an inclusion criteria for many of the interventional trials, and therefore, should be a measurement that is accurately obtained ( Fig. 150.3 ).

In asymptomatic patients, echocardiography most commonly finds RHT in the RA, where the tricuspid valve apparatus seemingly acts to delay immediate advancement into the RV or the pulmonary artery (PA). In symptomatic patients, echocardiography usually demonstrates acute cor pulmonale (RV/LV ratio > 1.0; RV dysfunction; tricuspid regurgitation max > 2.8 m/sec), and any visualized RHT represent a residual portion of a PTE. In the largest study reported on symptomatic free-floating RHT, 79% of RHT were in the RA, 16% were in the RV, and 5% were in both right heart chambers. Deep venous thrombi (DVT) were found in more than 90% of patients who underwent diagnostic evaluation, and a PTE was confirmed in all but one patient (97%).
Right heart thrombi morphology and outcomes
Patients with free-floating RHT that have embolized from an upper or lower extremity DVT have a reported mortality of greater than 40%. Alternatively, RHT may form in situ from myocardial dysfunction, from endothelial injury, or may form directly on a foreign body. Because patients often have an asymptomatic clinical status, RHT may have the time necessary to become extremely large. They often mimic the chamber they form in and are seemingly lengthy and wormlike when they form within a vein, but are round and ball-like when they form in the RA.
A classification scheme has been developed and should be used in clinical practice because it correlates with an increased probability for embolization and subsequent morbidity and mortality ( Table 150.2 ). High-risk RHT are mobile, vessel-shaped masses that may extend from the RA across the tricuspid valve orifice into the RV or PA (type A). These can be exceedingly long and may extend from the vena cava into the main or branch pulmonary arteries. ( Fig. 150.4 /Video 150.4). Rarely, a large RHT may be seen to cross through a patent foramen ovale and become positioned within both the RA and LA chambers, raising the potential to embolize systemically (paradoxically), thus creating a stroke ( Fig. 150.5 /Video 150.5). These patients have a high incidence of associated DVT, and less commonly, a thrombogenic cardiac pathology or RV cardiomyopathy.
Characteristic | Type A | Neither (AB) | Type B |
---|---|---|---|
Shape | Wormlike | Mixed | Round |
Mobility | +++ | +/++ | −/+ |
DVT | Likely | Unknown | Rare |
RH pathology | Absent | Unknown | Present |
PTE (any) | 100% | Unknown | 40% |
PTE (fatal) | 27% | 0% |
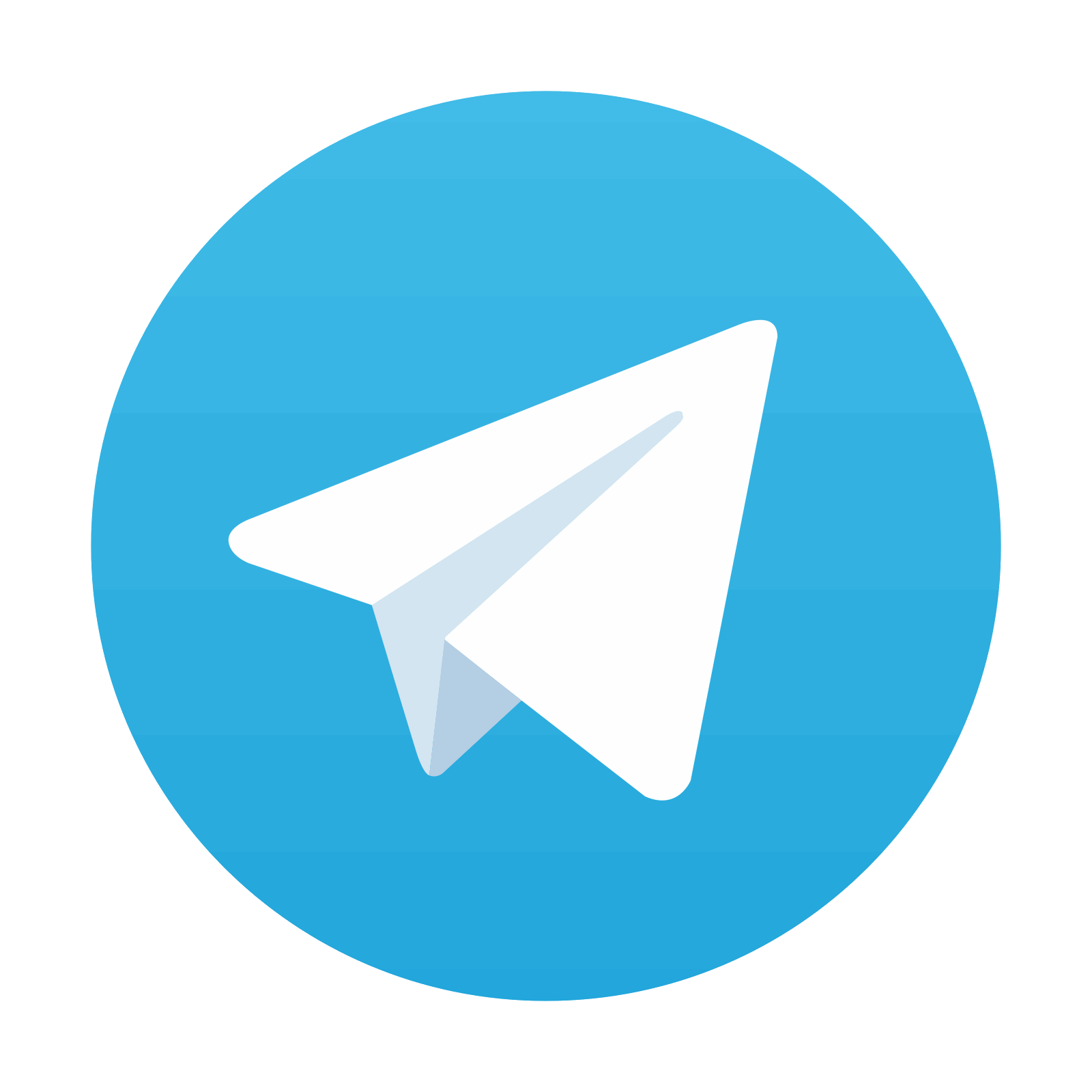
Stay updated, free articles. Join our Telegram channel

Full access? Get Clinical Tree
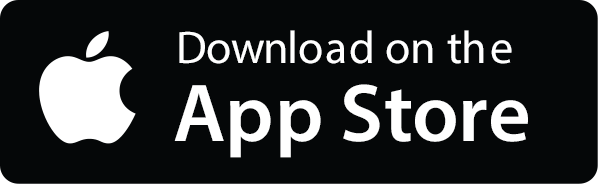
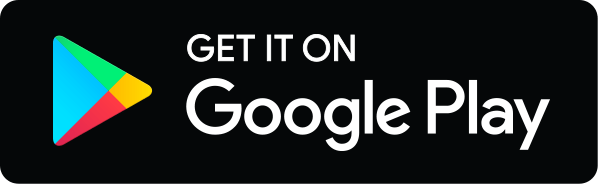
