Fig. 20.1
Transposition of the great arteries
Two main hypotheses are recognized (Marino et al. 2002). Gore and Edwards proposed that TGA was caused by the lack of the normal clockwise rotation of the aorta (AO) toward the left ventricle (LV), presumably due to the lack of maturation of the subpulmonary conus with persistence of the subaortic conus (Goor and Edwards 1973). (“Conus” specifically refers to the muscular cardiac segment between the atrioventricular valves and the semilunar valves as defined by Drs. Van Praagh and Van Praagh.) In this scenario, TGA is an extreme case of aortic malposition that is on the spectrum of defects that includes malalignment ventricular septal defects, tetralogy of Fallot, and double outlet right ventricle. This theory seems to adequately explain the morphologic differences seen in TGA related to variations of the right ventricular outflow tract (RVOT) or left ventricular outflow tract (LVOT) and the positions of the pulmonary artery and VSD, but the presentation of TGA with an intact ventricular septum is difficult to explain. A simpler theory by de la Cruz offers that linear rather than spiral septation of the truncus occurs and the failure of the aortopulmonary septum to spiral leads the aorta to remain in continuity with the anterior conus of the right ventricle (De La Cruz and Da Rocha 1956). This explanation, however, fails to clarify the origin of the large variability of conal anatomy that presents in TGA.
Terminology and Anatomy
Historically, the basis for terminology describing a complex cardiac defect is derived from pathologic examinations of cardiac specimens and refined by clinical and physiologic correlation and ultimately an understanding and description of the failure of morphologic development. The absence of a unifying theory of the maldevelopment of the heart in TGA and the large variability of anatomic presentations related to the position of the great vessels relative to each other and observed outflow tract abnormalities have made consistent descriptive terminology problematic (Mair et al. 1971). A nomenclature report on TGA published in a review in 2000 provides details of widely accepted terminology that are beyond the scope of this chapter (Jaggers et al. 2000).
The classification suggested by Van Praagh describes morphology and forms the general basis for most imaging descriptions of the anatomic variables in TGA (Houyel et al. 1995; Van Praagh 1984; Van Praagh et al. 1975). This format is useful for conceptualizing the malformation and its variations. Cardiac position is left sided (levocardia), right sided (dextrocardia), or midline (mesocardia). The main cardiac segments are the atria, ventricles, and great arteries. The connecting cardiac segments are the atrioventricular (AV) canal complex between the atria and ventricles and the conus between the ventricles and the great arteries. Malpositions of the main segments may be described as solitus, inversus, or ambiguous. Additionally the visceral situs is described as situs solitus if the pulmonary (right) atrium is on the right and the right-sided abdominal organs (the liver, gall bladder) and the trilobe lung are also on the right and the pulmonary venous (left) atrium and the left-sided abdominal organs (the stomach, spleen) and the bilobe lung are on the left. Situs inversus is mirror image reversal of the normal situs solitus. Situs inversus is rare and not usually associated with medical issues. Diagnosis is usually incidental, while the patient is undergoing imaging for other conditions. Situs ambiguous refers to the random abnormal distribution of the abdominal organs and is highly associated with heterotaxy cardiac malformations, including single ventricle lesions.
The sequential segmental analysis usually reported by echocardiogram in TGA includes the cardiac position and direction of the apex, the position and morphology of the atria, the atrioventricular connections, the ventriculoarterial (VA) connections, and the positions of the great vessels. Atrioventricular connections are concordant when the systemic venous right atrium (RA) empties into the morphologic right ventricle and the pulmonary venous left atrium empties into the morphologic left ventricle. Discordance refers to when the systemic venous right atrium empties into the morphologic left ventricle and the pulmonary venous left atrium empties into the morphologic right ventricle. The discrete commitment of the atrioventricular apparatus to the ventricular inlet is also described. A double inlet ventricle has both AV valve apparatus committed to the ventricular inlet. Ventriculoarterial or outlet connections are referred to as concordant if the pulmonary artery is connected to the right ventricle and the aorta to the left ventricle and discordant if the pulmonary artery is connected to the left ventricle and the aorta to the right ventricle. Double outlet connections refer to one great vessel and more than 50 % of the other committed to either the right or the left ventricle. Note that the above classification describes the intrinsic connections of the main cardiac and connecting segments sequentially and does not describe the positions of the great arteries relative to each other.
Simple Transposition of the Great Arteries
Pathologic heart specimens showing an aorta arising from the right ventricle and the pulmonary artery from the left ventricle have been observed since the late 1700s (Leibman et al. 1969). Historically, more than half of infants presenting as neonates with severe cyanosis have the diagnosis of TGA (Farre 1814). TGA is associated with an intact ventricular septum 55 % of the time and with a VSD of variable size 45 % of the time. The age of the demise of such patients historically seemed related to the size of the atrial septal defect, with most not surviving infancy (Leibman et al. 1969). Contemporary analysis shows that TGA occurs approximately 1:3200 live births with a strong male predilection of 1.6–3.2:1 (Hoffman and Kaplan 2002). The segmental description of simple TGA is atrioventricular concordance with ventriculoarterial discordance (Van Praagh 1984; Allen et al. 2001a). The designation of the spatial relationship of the great vessels as “D” or “L” completes the anatomic description. D-TGA refers to the location of the right and left ventricles in their normal positions. The aorta is usually anterior and to the right of the pulmonary artery, although occasionally it may be directly anterior or anterior and to the left.
The hemodynamic derangement from TGA is derived from the two parallel loops of circulation created by the transposed great arteries (Ewer et al. 2012). One loop is:


Likewise, the other loop is:


In the worst case, the highly oxygenated blood in the pulmonary venous loop never accesses the systemic circulation, and the systemic output from the aorta in the systemic venous loop never accesses the pulmonary vascular bed to allow oxygenation and carbon dioxide exchange. Extrauterine survival requires a site of mixing such as an intracardiac shunt (ASD or VSD) or extracardiac shunt (PDA) (Fig. 20.2).


Fig. 20.2
Arterial and venous mixing in TGA
An additional hemodynamic issue important in the first few months of life is related to the pulmonary vascular bed being in connection with the left ventricle. In utero and during transition from fetal to neonatal circulation, the pulmonary vascular resistance (PVR) is high. At birth, the PVR decreases rapidly as the lungs are expanded and the vascular bed relaxes because of the effect of vasoactive mediators and oxygen (Fig. 20.3). In the weeks and months following birth under normal circumstances, the medial muscle layer of the pulmonary arteriole regresses and remodels. In this period the left ventricle will decondition as PVR decreases, unless there is a nonrestrictive VSD to allow the resistance circuit of the LV to include systemic circulation via the RV.


Fig. 20.3
PVR transition
Although recent recommendations show screening of newborns routinely with pulse oximetry is effective in detecting cyanotic lesions such as TGA, this practice only benefits patients with TGA who have an adequate ASD to survive the early neonatal period (Ewer et al. 2011; Rasiah et al. 2006). Unfortunately, a routine obstetrical ultrasound may not carefully evaluate the outflow tracts and great vessels in the fetus, but a carefully performed fetal cardiac ultrasound that identifies TGA potentially allows the fetus to be delivered in a facility prepared for the expectant use of prostaglandin E (PgE) and transport availability for subspecialty cardiology care (Calderon et al. 2012). Prenatal diagnosis reduces the potential neurodevelopmental complications that may arise in a neonate with TGA when compared to patients whose diagnosis is delayed until severe cyanosis and cardiovascular collapse occur after birth because the patent foramen ovale is too small for adequate mixing and the ductus arteriosus closes (Blalock 1950).
Clinical Presentation
The clinical presentation of neonates with TGA is variable. For survival there must be a shunt at the atrial level, the ventricular level, or a patent ductus arteriosus. If there is inadequate shunting, cyanosis is severe and cardiovascular collapse occurs rapidly. Although most neonatologists would institute PgE in the face of cyanosis without overt respiratory distress syndrome, a PDA is not as efficient as an atrial septal defect in providing mixing of systemic venous and pulmonary venous blood. For this reason after diagnosis by echocardiogram, emergency balloon septostomy is routinely performed either at the bedside with echocardiographic guidance, or in the catheterization lab if the septostomy is anticipated to be complex. The helpfulness of a ventricular septal defect, if present, is variable. Depending on the size of the VSD and in particular the morphology of the conal septum, the VSD may be either an effective source of mixing or ineffective because the streaming characteristics of blood ejected through the conal septum render the VSD inoperative as a site of mixing.
Patients with TGA who have large ASDs or VSDs may be more difficult to detect at birth. Their cyanosis may be mild to moderate and if overlooked, the other physical signs of TGA may not be impressive. As the PVR decreases in the days and weeks after birth, a normal infant demonstrates a modest increase in arterial saturation, but the infant with TGA will demonstrate progressive high-output heart failure and continued desaturation. Chest x-ray will show increased pulmonary vascular markings consistent with pulmonary overcirculation and cardiomegaly, especially if there is an unrestrictive VSD present. Symptoms and signs of a left-to-right shunt such as poor weight gain, diaphoresis, tachycardia, and a cardiac gallop may be present. Persistent cyanosis is a function of the intracardiac mixing that occurs despite an elevated Qp/Qs ratio.
Historical Therapeutic Approaches and Outcomes
Before discussing the details of contemporary surgical management of TGA, it is useful to review the advances in surgical technique and physiologic insight that led to the current application of the arterial switch. Early attempts to palliate TGA preceded the era of open-heart surgery. Autopsy specimens had led to the understanding that the age of a patient’s demise was strongly related to the presence and size of an intra-atrial communication. The Blalock-Hanlon procedure was described in 1950 and represented the first palliative operation that seemed to be associated with improved survival in older infants compared to the natural history of the unrepaired lesion (Blalock 1950; Blalock and Hanlon 1948). Using a modified technique of inflow occlusion, a surgical ASD was created via a right thoracotomy. Increasingly surgery was performed in younger infants with an expected early survival over 85%, with most patients operated on from the 1940’s to the 1970’s experiencing improvements in percent arterial saturaion (Hermann et al. 1975). The midterm and late results were not nearly as good (Alexi-Meskishvili and Sharykin 1984). Mortality in the weeks after surgery was still substantial, but survivors of the first few months tended to have prolonged survival to 10 years or more. Nonetheless it was clear that a more definitive approach to palliation was required.
Early attempts to repair the lesion by arterial switch were largely unsuccessful because of the technical difficulty in transferring the coronary arteries to the neoaorta (Yacoub and Radley-Smith 1978; Senning 1959; Bailey et al. 1954). Animal experiments by Alfred Blalock and C. Rollins demonstrated in a canine model the feasibility of connecting the pulmonary veins to the superior vena cava (SVC) and the right atrium (RA) and hypothesized that a similar approach might be made in infant patients with TGA (Blalock and Hanlon 1948). This introduced consideration that instead of reversing the aorta and pulmonary artery, the pulmonary and systemic venous pathways might be reconstructed, obviating the need for coronary reconstruction inherent to the arterial switch. In 1952 Walton Lillehei attempted an extracardiac “venous switch” by connecting the right pulmonary veins to the right atrium and the inferior vena cava (IVA) to the left atrium (Lillehei and Varco 1953). Further modifications by Thomas Baffes and Willis Potts in 1956 were described and as these procedures were done without cardiopulmonary bypass, they were commonly used for the next 10 years with increasing short-term success (Baffes 1956). In 1954 Harold Albert published the use of an intra-atrial flap to redirect systemic and pulmonary venous flow to the contralateral atrioventricular valve in dogs (Albert 1954). William Mustard and others advanced the technique that became the Mustard procedure, which used a prosthetic patch to create the intra-atrial baffle on cardiopulmonary bypass, diverting pulmonary venous blood to the tricuspid valve and systemic venous blood (SVB) to the mitral valve (Mustard et al. 1964). Also in the late 1950s, Ake Senning demonstrated the atrial switch could be done with autologous material (pericardium), although the procedure seemed more difficult (Senning 1959) (Fig. 20.4). Initially in the 1960s Senning’s procedure was largely abandoned in small infants because the technical challenges of creating the patch reduced the size of the atrial chambers, and low cardiac output after surgery was common (Mustard et al. 1964). The Mustard procedure was more successful in most surgeons’ hands and its survival statistics continued to improve. However by 1975, the midterm shortcomings of the Mustard procedure were becoming more obvious as older survivors presented with right- and left-sided venous obstruction as they grew (Quaegebeur et al. 1977). Arrhythmias and heart block were also increasingly common by 10 years after a Mustard procedure. Resurgent interest in Senning’s procedure, which avoids nonautologous materials, and the increasingly successful technical application of the technique in small infants, made it the most common palliative procedure performed for TGA through the 1980s (Senning 1959). Baffle obstruction due to patient growth in the first 10 years was reduced compared to the Mustard procedure and cardiac surgeons better managed the technical complexities of the baffle in infants. In many centers, infant survival of the Senning and Mustard procedures was equivalent and the midrange outlook for the patients with Senning baffles somewhat better (Williams et al. 2003).


Fig. 20.4
Mustard/Senning intracardiac blood flow
In institutions performing Senning and Mustard procedures for TGA, care of the neonate and young infant was focused on expedient cardiac diagnosis and resuscitation of hypoxemia and low cardiac output. Atrial septostomy during stabilization was essential and was performed by balloon or blade, usually in the catheterization laboratory. As adequate atrial mixing was established, vasopressor and PgE infusions could usually be weaned and discontinued. Oxygen and ventilator therapy were also weaned and discontinued. In many instances, the stabilized infant was observed to make sure that atrial mixing remained adequate and that pulmonary vascular resistance was falling and then discharged home with careful follow-up.
In the 1980s the mortality for complex cardiac surgery in neonates was higher than contemporary survival data, partly because other than extracorporeal membrane oxygenation, reliably effective medical therapy for pulmonary hypertension was not available. (Nitric oxide by inhalation became available in the early 1990s in some institutions, and in that decade the management of persistent pulmonary hypertension in pediatric cardiac patients who were still in transition when undergoing surgery became more effective.) It was common to defer when possible repair of TGA for at least a few weeks to months. When the infant was observed to have overcirculation of the lungs and mild high-output heart failure, an atrial switch procedure was performed electively. Most institutions felt that the intra-atrial baffle part of the procedure was technically easier on an infant who was 4 kg rather than on a neonate, and surgery better tolerated when PVR had fallen. With regard to intraoperative management of inspired oxygen (FiO2), minute ventilation, and expected arterial saturations (SaO2), cardiac anesthesiologists in that era learned from the neonatal care of infants with TGA that although increases in pulmonary to systemic flow ratios (Qp/Qs) could be accomplished with ventilator maneuvers and increased FiO2, in the presence of adequate atrial level mixing, the changes in Qp/Qs are not reflected in major improvements in arterial saturation.
As survival data from patients with TGA from the 1950s onward accumulated, it became obvious that although atrial switch palliation was superior to atrial septectomy in improving survival through infancy and childhood, there were still long-term challenges for the 84 % who survived 10 years and the 77–80 % who survived 20–30 years (Williams et al. 2003). Event-free incidence in survivors over three decades was less than 20 % (Cuypers et al. 2014). Events were described as late failures of the operative procedure (reoperation required for baffle-related obstruction of systemic or pulmonary venous flow). Events could also be natural history issues such as arrhythmias and heart block, and late heart failure due to systolic dysfunction and tricuspid regurgitation as the inability of the right ventricle and tricuspid valve (physiologic “mitral valve”) to function as systemic pumping structures over a lifetime became more obvious (el-Said et al. 1972; Culbert et al. 2003; Martin et al. 1990).
Contemporary Management and Interventions Prior to Surgery
Medical stabilization of the newborn diagnosed with TGA involves managing acidosis and hypoxemia that occurs when the intracardiac shunts are insufficient to allow adequate mixing and the ductus arteriosus closes. In any newborn patient with cyanosis of cardiac origin, PgE is administered to reconstitute ductal patency and allows blood flow into the lungs from the aorta (Fig. 20.5). Although in TGA, this maneuver increases pulmonary blood flow, unless there is an adequate amount of intracardiac mixing, the improvement in cyanosis may be negligible. Therefore, in the absence of a large ASD or VSD, balloon or blade atrial septostomy is usually required to improve atrial level mixing (Fig. 20.6). Successful enlargement of the atrial level shunt should result in immediate improvement in arterial saturation (SaO2) as measured by pulse oximetry.



Fig. 20.5
TGA/PDA

Fig. 20.6
Atrial septostomy
Some neonates and infants who have adequate mixing after an atrial septostomy may fail to improve their oxygen saturations if they also have pulmonary hypertension (Newfeld et al. 1974). Pulmonary hypertension as a transitional problem in neonates with cardiac disease is common, usually transient, and may be managed with nitric oxide by inhalation. Additionally, TGA patients are known to occasionally present with persistent pulmonary vascular disease that seems out of proportion to the duration of time they experience physiology that predisposes them to pulmonary overcirculation (Roofthooft et al. 2007). In this small subset of TGA patients, pulmonary hypertension may be less reactive, and careful hemodynamic assessment is required before surgical correction is undertaken to rule out other lesions that may have put the pulmonary vascular bed at risk by elevating pulmonary venous pressure.
An unresolved issue is the contribution of atrial septostomy to the ultimate development of brain injury due to embolic stroke or intracerebral hemorrhage. Although the Rashkind septostomy has been performed since the 1960s, the issue of optimizing neurodevelopmental outcome was less relevant in the era where surgical outcomes were not as successful (Rashkind and Miller 1966). As surgical palliation with the Senning and Mustard procedures was replaced in most centers with surgical correction by the arterial switch, survival outcomes continued to improve through the 1990s until today. Much research is now devoted to optimizing functional outcomes, including neurodevelopmental achievement and long-term freedom from reoperation. When imaged with MRI, patients with TGA (like many cardiac anomalies), have a higher incidence of structural brain anomalies and periventricular leukomalacia than infants without cardiac defects. Although there is literature indicting the septostomy procedure as an additional risk for brain injury by provoking embolic phenomena, the clinical context of hypoxia and decreased cardiac output may play a contributory role (McQuillen et al. 2006; Petit et al. 2009). The independent relation of the septostomy in causing neurologic injury has been both confirmed and discarded by conflicting studies.
The presence of a VSD is an unreliable site of mixing in many cases because of streaming of pulmonary and systemic venous blood past the septal defect into the respective great vessels. Nonetheless, a VSD does have implications on the hemodynamic modeling of left ventricular systolic performance prior to surgery in that if the VSD is large enough, the LV does not decondition as the PVR drops. In TGA with an intact ventricular septum, the normal fall in pulmonary vascular resistance that occurs the first few weeks of life is associated with reduced systolic pressure in the left ventricle. In the era of atrial switch palliation where the left ventricle remained the pulmonary ventricle, this was not a relevant issue. But the current application of the arterial switch procedure that restores the left ventricle as the systemic ventricle makes it an important consideration (Adhyapak et al. 2007). The arterial switch procedure is usually performed the first few weeks of life in order to avoid imposing systemic workload on a deconditioned left ventricle. Performing the procedure while the LV is still conditioned to perform at “systemic” pressures is associated with improved outcomes and reduced need for prolonged vasopressor support or extracorporeal support for ventricular failure.
Contemporary Surgical Management and Outcomes
In the 1970s when most infants were undergoing Mustard and Senning procedures for TGA, it was identified that patients with TGA/VSD continued to have poor outcomes despite early good results in TGA with intact ventricular septum. The arterial switch procedure was therefore applied to these patients with poor but improving short-term outcomes. Unlike the Norwood procedure for hypoplastic left heart syndrome where the “transfer of technology” was very slow and widespread improvement in outcomes delayed, the technical details associated with success of the arterial switch were widely adopted, and by the mid-1980s most neonates with TGA with or without a VSD underwent arterial switch, accomplishing anatomic correction.
The arterial switch procedure was described by Jatene in 1976 and has undergone modifications, including those proposed by Lecompte in order to improve the technical performance of the great vessel reconstruction, and improvements in techniques of coronary reimplantation especially when there are variations from the normal origination of the coronary ostia. Although there are institutional variations in technique, the common elements are:
- 1.
Median sternotomy is performed with harvesting of pericardium for patch augmentation of the neopulmonary artery or septal defect closure.
- 2.
The coronary artery anatomy is inspected to identify coronary anomalies and plan reimplantation sites.
- 3.
The great vessels, branch pulmonary arteries, and patent ductus arteriosus are mobilized.
- 4.
Heparin is administered and cardiopulmonary bypass initiated with bicaval or single atrial venous cannulation. The PDA is ligated as bypass is initiated. Temperature and perfusion techniques vary among institutions, but deep hypothermic circulatory arrest is rarely indicated.
- 5.
Venting of the left ventricle is accomplished by a pulmonary venous catheter or through the ASD.
- 6.
Aortic occlusion and cardioplegia administration are routine.
- 7.
Patch closure of any septal defects is done.
- 8.
The aorta is transected above the sinotubular junction and the coronary arteries are disconnected with a button of aortic tissue.
- 9.
The main pulmonary artery (MPA) is transected.
- 10.
Coronary button reimplantation to the neoaorta and anastomosis of the ascending aorta to the proximal neoaorta is performed.
- 11.
Relocation of the pulmonary artery bifurcation to the neopulmonary artery with patch augmentation as needed is done.
- 12.
De-airing of the heart and reperfusion after removal of the aortic cross clamp begins the period where the reconstruction is critically assessed. Coronary perfusion should be immediately obvious and myocardial recovery rapid as evidenced by the return of a normal electrocardiogram. During reperfusion, echocardiographic evidence of adequate systolic ventricular function should be verified with particular assessment for wall motion abnormalities of the left ventricle that might indicate a technical problem with coronary reimplantation. Usually coronary issues result in overt ventricular dysfunction in the distribution of the left main or right coronary artery, and this assessment is straightforward when the aortic occlusion time is not excessive and myocardial protection meticulously performed. However in the event of a prolonged cross-clamp time or technical issues that interfere with adequate cardioplegia administration, the differential diagnosis of ventricular systolic dysfunction is more problematic (coronary issue versus myocardial stunning). Direct inspection of the coronary arteries and their perfusion as well as the epicardial appearance of well-perfused myocardium is essential.
The issue of LV reconditioning after repair in the TGA patient manifests even after successful surgery. (The fragility under stress of apparently “normal” ventricular function may be especially important in infants whose convalescence after surgery is complex, necessitating additional operative procedures or imaging studies requiring anesthetics.) The only patients who (intraoperatively) retain systolic LV performance adequate to easily assume systemic workload are the TGA patients who have a large VSD. All others can be assumed to have some intolerance to the requirement that they accomplish a “normal” systolic pressure after repair. This may persist for days to weeks after repair.
Additionally the issue of poor ventricular diastolic function makes intraoperative management of the patient’s hemodynamics challenging. The conduct of reperfusion after removal of the aortic cross clamp is critical. The LV after arterial switch is extremely noncompliant and easily distends with small volume overload. The release of the vena cava tapes and filling of the heart on bypass require great care and are just as critical as when weaning from bypass in order to avoid distending the LV. Ventricular distension causes longitudinal stretch of the coronary arteries leading to further ventricular dysfunction due to myocardial ischemia. The author’s preference is preemptive inotropic support with dopamine or low-dose epinephrine and lusitropic support with milrinone in order to optimize systolic and diastolic function with a small left ventricular end-diastolic volume. The value of nitroglycerin to prevent coronary spasm in neonates is controversial. Potent vasodilators such as nitroprusside are difficult to use in neonates with poor diastolic function because the margin between effective afterload reduction and overt hypotension may be small. If cardiac distension occurs after weaning from bypass, rapid removal of intravascular volume may be necessary or return to cardiopulmonary bypass may be required.
Additional echocardiographic examination to discover residual lesions such as intracardiac defects or valve problems should be performed during reperfusion. As the cardiac chambers are gently filled during bypass, evidence of pulmonary hypertension should be evaluated by examining the amplitude of the Doppler jet of tricuspid insufficiency. Overt right ventricular failure is rare even in the presence of pulmonary hypertension, but leftward ventricular septal shift may compromise left ventricular function. The right and left ventricular outflow tracts and proximal great vessels should be interrogated for evidence of turbulence indicating problems with a VSD patch or arterial reconstruction. The pulmonary artery branches should be visually inspected to make sure they are not under tension. The great artery reconstruction usually places the main pulmonary artery anterior to the aorta with the branch pulmonary arteries draping to either side making recannulation of the aorta somewhat more difficult if needed after cannula removal. Complete examination of the anatomic adequacy of repair should be performed prior to decannulation.
Postoperative intensive care is typical of any neonate having complex cardiac surgery. Control of the inflammatory effects of cardiopulmonary bypass and the resulting coagulopathy and capillary leak, optimization of cardiac output especially in the first eight hours after reperfusion when myocardial energy stores are being normalized, and careful hemodynamic monitoring and intervention are required. Monitoring acid-base status is often supplemented by determinations of lactate levels, mixed venous oxygen saturation, and cerebral and somatic near-infrared spectrometry to assess cardiac output (De 2008; Kreeger et al. 2012; Weiss et al. 2005; Ranucci et al. 2010; Chakravarti et al. 2009). Injury currents on the electrocardiogram are abnormal and should prompt consideration of coronary insufficiency that is likely technical in origin. Temporary renal insufficiency is often seen and meticulous attention to fluid management, electrolytes, and diuresis is required. Glucose management is controversial, and although insulin is often required postoperatively for hyperglycemia, the unconfirmed benefits of “tight control” must be weighed against the risk of iatrogenic hypoglycemia to the neonatal brain (Falcao et al. 2008; Scohy et al. 2011). For some centers, leaving the sternum open for a brief time postoperatively is an institutional preference. Ventilator support is typically provided until hemodynamic stability is accomplished and sedation weaned. Although narcotics and minor tranquilizers are commonly used for postoperative sedation, emerging strategies, such as dexmedetomidine, an alpha 2 agonist, are accumulating an increasing evidence basis for use (Chrysostomou et al. 2009; Obayah 2006; Jones 2013). The use of propofol for postoperative sedation is problematic because of its deleterious effects on hemodynamic function and the rare possibility of lactic acidosis. Muscle relaxants may be used intermittently if needed in the intubated patient, but their routine or prolonged use may lead to increased incidents of failed or delayed weaning from ventilator support. Neonates who have been given muscle relaxants must be carefully assessed prior to extubation, ideally with a nerve stimulator. Residual weakness is common and the duration of action of even intermediate acting agents is prolonged when given repeatedly or as an infusion, especially in the neonate and small infant whose pharmacokinetic processes are not mature.
As initial application of the arterial switch procedure progressed as an alternative to a Senning or Mustard procedure in the patient with TGA/VSD in the 1970s to its increasing application as the standard of care in the 1980s, the surgical mortality declined significantly (Cohen and Wernovsky 2006). Institutional mortality rates for simple TGA with either intact interventricular septum or with a VSD vary between 2 and 11 % and often are used to benchmark the performance of a pediatric cardiac surgery program (Rudra et al. 2011). Known risk factors for increased mortality include low birth weight or prematurity, complex coronary anomalies or intramural coronary artery, concomitant LV obstruction at the conal septum or aortic arch (in patients with a VSD), and late presentation and diagnosis beyond the neonatal period. Institutional experience of the operating team, including the surgeon, anesthesiologist, and perfusionist, seems to also be related to success (Hirsch et al. 2008).
Not only are the expected short-term outcomes excellent, the long-term outcomes are much better than those seen in patients who have had a Mustard or Senning procedure (Dibardino et al. 2004). Late survival of over 90 % is expected, mostly intervention free (Ruys et al. 2013). Arrhythmias, heart block, and ventricular dysfunction are rare, and most patients have a normal functional class (American Heart Association) and exercise tolerance. Late follow-up of these patients is focused on the long-term fate of the main pulmonary artery and its branches and the aortic valve. Late stenosis of the pulmonary artery branches and dilation of the pulmonary artery or aortic root are described and are the focus of technical modifications of the surgical technique. Occasional reoperations to revise the aortic root or replace the aortic valve are reported (Cohen and Wernovsky 2006). There are a small number of postoperative patients (up to 10 %) who have silent coronary insufficiency detected on myocardial perfusion imaging that has prompted preemptive stent intervention in the catheterization lab. These coronary problems may be related to ostial injury, late fibrosis or reactive injury to the artery, or late kinking from epicardial scarring, and their natural history is unknown (Bonnet et al. 1996). The oldest survivors of successful arterial switch have not yet commonly undergone coronary revascularization.
Comorbidities
TGA is rarely associated with genetic syndromes (e.g., Turner, Noonan, Williams, Marfan, or Down syndromes), chromosome abnormalities such as trisomy 8 and 18, or anomalies involving the same embryologic substrate as the cardiac tube or branchial arches (e.g., VACTERL and CHARGE syndromes) (Ferencz et al. 1995, 1997; Bonnet et al. 1996; Marino 1996). There is an occasional infrequent association with chromosome deletion 22q11 and DiGeorge syndrome (Melchionda et al. 1995; Van Mierop and Kutsche 1986). Heterotaxy and isomerism syndromes are the only genetic syndromes highly associated with TGA (Ferencz et al. 1995).
Additional Anesthetic Considerations
The preanesthesia evaluation should focus on defining the patient’s anatomic findings and the physiologic impact of those findings. Adequacy of neonatal resuscitation in patients who presented with severe cyanosis and shock should include the recovery of end organ performance such as cardiac function, renal and liver function, and assessment of any neurologic injury. The adequacy of mixing after septostomy by echocardiogram and the patient’s pulse oximetry should help identify patients who have pulmonary hypertension and are significantly desaturated despite imaging evidence of an adequate ASD. This should prompt consideration of whether the expected transitional change in PVR is delayed or alternatively if an unappreciated cardiac anomaly exists that threatens the pulmonary vascular bed. If PgE is being administered, the presence of apnea or other complications of its use such as fever, hypotension, or reduced gastrointestinal perfusion pressures should be considered. In the patient with a large, widely patent ductus arteriosus and a VSD, the possibility that there is also an unrecognized coarctation of the aorta should be considered. This disastrous finding would present when the PDA is ligated at the onset of bypass as elevated resistance to flow and delayed lower body cooling on cardiopulmonary bypass and venous desaturation and metabolic acidosis despite adequate perfusion flows.
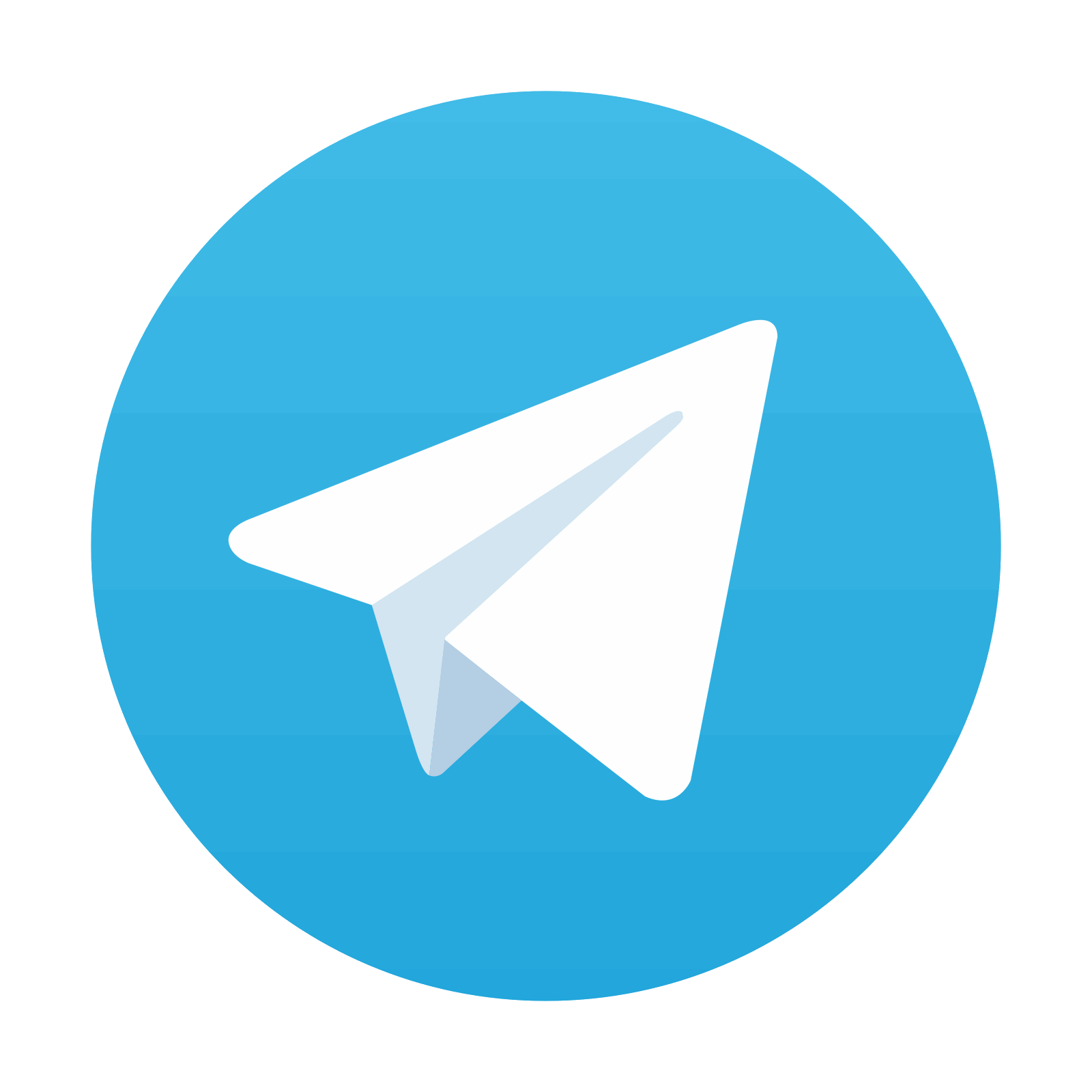
Stay updated, free articles. Join our Telegram channel

Full access? Get Clinical Tree
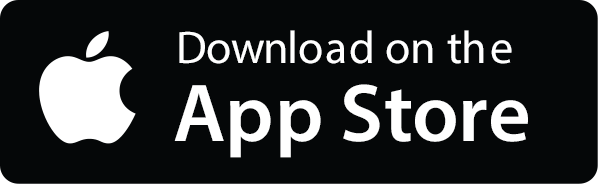
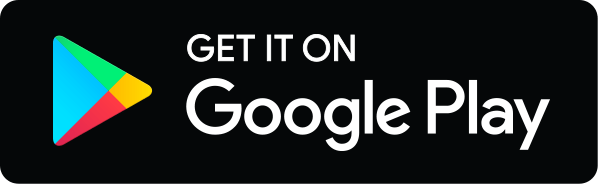